Genetics in Rhinology
Summary
Diseases that affect the upper airways, including allergic rhinitis and chronic rhinosinusitis (CRS), are complex disorders with clear genetic factors, most of which remain unknown. The pathobiology underlying these conditions represents a complex interplay between multiple genetic and environmental factors. Although the genetic studies conducted on allergic diseases to date have greatly contributed to our understanding of the pathogenesis of allergic rhinitis (AR), asthma, and CRS, as evidenced by association of single nucleotide polymorphisms with the allergic phenotype, most of these observations remain to be translated into clinical practice. In contrast to cystic fibrosis (CF), which is an example of a single gene disorder resulting in severe airway diseases, these disorders are more complex, as each of them is influenced by several different genes and gene networks that contribute to the disease pathogenesis. The identification of specific environmental risk factors that interact with the subject′s genome may help prevent disease onset or can help alleviate allergic symptoms. As we uncover more disease-causing genes, we also will be able to improve the diagnosis of and treatment for patients with allergies and CRS based on subclassifications of disease through genetic profiling.
Introduction
Atopic (allergic) diseases are among the most common chronic disorders in the developed world. Although the underlying cause remains unknown, atopic diseases are recognized as complex genetic disorders in which multiple genes interact with each other and with the environment to trigger variable expressions of the atopic phenotype. As such, the atopic manifestation can occur in the airways/lungs (i.e., asthma), on the skin (i.e., atopic dermatitis), or in the gastrointestinal tract (i.e., food allergies or eosinophilic esophagitis), as well as in the eyes and nose, in the form of rhinoconjunctivitis, and systemically, as seen in food allergy and anaphylaxis.
Until recently, the genetics of atopy have been investigated using genome-wide linkage analysis and candidate gene association studies.1,2 More recently, genome-wide association studies (GWASs) have been used to investigate allergic diseases, asthma, and CRS. Indeed, the, GWAS approach is rapidly replacing the more traditional candidate gene studies and microarray-based linkage mapping approach due to its comprehensive and unbiased strategy to identify causal genes that underlie complex diseases, such as asthma, AR, and CRS. This progress was made possible by key developments in human genomics over the last decade, with the completion of the human genome sequence being the first step.3
When using the candidate gene approach, genes are selected based on their known or suspected biology, suggesting they may have a role in disease susceptibility. After the candidate gene is selected, sequence variations within the gene are compared between individuals with disease and controls without disease. Sequence variations (polymorphism) that are found more often in individuals with disease are then further investigated. This approach has been hampered by the lack of reproducibility. Candidate gene studies are also limited by the current state of knowledge regarding susceptibility genes, which can result in study bias because only known genes are included.4
Caution
Candidate gene studies can easily result in study bias because only known genes are studied.
Positional cloning by linkage is a hypothesis-independent approach and starts with the investigation of families. Linkage mapping relies on studying the transmission of multiple microsatellite markers, which are DNA variants that consist of simple sequence length polymorphisms scattered throughout the genome. A microsatellite marker allele tends to be inherited with the disease if the marker allele and the disease-causing allele are physically situated in close proximity on the same chromosome. The genes positioned in the region that have linkage between a particular marker and the phenotype can be examined for possible involvement in the disease process and the presence of disease-causing mutations in affected individuals. Linkage mapping has been a very useful tool in identifying genes causing single gene disorders, such as CF. A gene is identified based on its physical position along the length of the chromosome rather than from any prior knowledge of its biology. Linkage mapping, however, has not been as useful in identifying genes that have only a small or moderate effect on a disease, including many common disorders such as asthma.4 Association studies are most commonly used to investigate complex genetic disorders. Single nucleotide polymorphisms (SNPs)5 and copy number variants (CNVs)6 or other structural variations in DNA sequence due to deletions or duplications can be compared between cases and controls using this approach. Association is determined by identifying any statistically different frequency in one or more genetic markers between the two groups. GWAS scans use hundreds of thousands of SNPs and/or chorionic villus sampling (CVS) with microarray technology to study complex genetic disorders such as asthma. This allows the use of genome-wide, hypothesis-independent association studies that, unlike positional cloning by linkage, do not require large family-based samples. GWAS also has greater statistical power to detect small effect sizes, which is a function of the number of subjects examined. GWAS has now revolutionized the study of genetic factors in complex common diseases such as asthma and other allergic diseases.7,8
GWASs have been very successful in indentifying disease loci, using single-marker-based association tests that examine the relationships between each SNP marker and the trait of interest. However, this strategy is not designed to identify disease-causing variants, which require further fine mapping or resequencing. Some genes may be genuinely associated with disease status but may not reach a stringent genome-wide significance threshold in GWASs due to small sample sizes and lack of statistical power. Pathway-based approaches use prior biological knowledge on gene function to facilitate more powerful analysis of GWAS datasets. These approaches typically examine whether a group of related genes in the same functional pathway are jointly associated with a trait of interest.9 In GWAS the basic principle is to compare the frequency of each genetic variant between cases and controls. A statistically significant increased frequency in cases compared with control subjects provides evidence that a genetic variant is related to disease susceptibility. For example, for the National Institutes of Health (NIH) catalog of GWASs, P values ≤ 5 × 10 − 8 are required for statistical clearance of an association to a region of the genome referred to as the locus (www.genome.gov/GWAS).10 Replication studies to verify results are needed in multiple populations to determine the importance of the identified gene variants. Also, functional studies of identified variants are required to understand the role of these genes and the genetic variants in the pathogenesis of the disease.
Caution
Genome-wide association studies are not suitable for identifying disease-causing variants.
The ability to study the genetics of phenotypes that are intermediate between DNA variants and a clinical disease phenotype of interest can provide substantial additional power in (1) identifying predisposition loci and (2) inferring the true biological effect, which is essential for understanding disease etiology. Gene expression is one of these key intermediate phenotypes, and a number of studies have shown their value in the disease context, including asthma.11
Interindividual and intertissue variations in gene transcript levels are important in mediating disease susceptibility; these variations may be caused by either genetic or epigenetic differences. Epigenetic effects relate to environmental influences, where, for example, identical alleles may exhibit different phenotypes due to aging or environmental exposures that impact DNA function through methylation or other modifications of the DNA. Genetic variants influencing transcription may also include large-scale structural changes in the genome, such as gene duplications and deletions, or smaller changes, such as polymorphisms that influence the gene′s regulatory elements.
A wide array of epigenetic modifications can influence gene expression ( Fig. 5.1 ). DNA cytosine methylation plays an essential role in cellular differentiation and development and has been firmly linked with disease susceptibility.12 Recent studies have shown significant interindividual patterns of methylation and changes in patterns over time with aging and with exposure to environmental stressors, all of which can influence disease development and progression.13,14
Allergic Rhinitis
AR is the most common chronic disease in developed countries; for example, the prevalence of clinically significant AR varies between 17 and 29% in Europe.15 AR is characterized by immunoglobulin E (IgE)–mediated inflammatory reaction in the nasal mucosa following exposure to an allergen, in most cases proteins but sometimes also chemicals (haptens), that then bind to proteins to form an allergen. Clinical symptoms include itching, sneezing, rhinorrhea, nasal congestion, and stuffiness. Symptoms are reversible after exposure discontinues or after symptoms are treated with allergy medications. AR significantly decreases the quality of life of patients and is known to be a risk factor for developing asthma.

It is generally accepted that asthma and AR are the result of inappropriate immunologic responses to common environmental allergens in genetically susceptible individuals. Association has been described between AR and certain chromosomal regions (e.g., chromosomes 2, 3, 4, and 9). These associations have been observed in different populations, including in studies from the United States, Europe, Scandinavia, and Japan. Based on twin studies, it has been estimated that AR exhibits an inheritability of 0.33 to 0.75.16
SNP studies of genes encoding molecules involved in the possible pathogenesis of AR have been published in recent years. There are reports of polymorphisms in genes encoding chemokines or their receptors (e.g., CCR1 [cluster of deviation 191, CD191], CCR2, CCR5, CCXCR1, SDAD1, CXCL9, CXCL11, CSCL10, RANTES [regulated upon activation, normally T-expressed, and presumably secreted], and eotaxin-3),17–20 interleukins or their receptors (e.g., IL-13, -18, and -28RA and GATA),21–23 eosinophils,24 and leukotrienes (e.g., LTC4S).25 Analysis of SNPs in the T cell receptor pathway has revealed a stretch of DNA sequence (haplotype) in the inducible T cell tyrosine kinase as a risk factor for seasonal AR.26 The difficulty of identifying disease-associated SNPs in AR has been hampered by small effect sizes of the contributing variants and underpowered studies. One gene or SNP alone typically confers only small effects on the disease; therefore, combinations of genes and SNPs are needed for large-scale effect in AR.
Chronic Rhinosinusitis
CRS is a common inflammatory disorder of the sinus mucosa that has been classified as having two different phenotypic forms: CRS with nasal polyps (CRSwNP) and CRS without nasal polyps (CRSsNP), according to the European Position Paper on Chronic Sinusitis (EPOS).27 Several gene studies and SNPs have been published that relate to both forms of CRS. Population-based genome-wide screening for CRS among 291 Hutterites, members of a genetically isolated religious group in the United States and Canada, linked a locus on chromosome 7q to the disease, suggesting that the CF transmembrane conductance regulator (CFTR) gene may influence disease susceptibility.28
An association of IL-1A with the development of CRSwNP was reported in a replication study of 206 patients with severe CRS.29 Reduced expression of several epithelial genes, such as S100A7, S100A8, and SPINK5 (serine protease inhibitor Kazal-type 5), has been reported in CRSwNP and CRSsNP. These findings suggest alteration in epithelial barrier function and host defense in CRS.30
Several different polymorphisms associated with CRS have been published but not replicated, including polymorphism in the tumor necrosis factor (TNF)-α gene in the Japanese population31 and IL-1 receptor-associated kinase 4 (IRAK4) polymorphism in the Canadian population.32
A subset of CRSwNP patients have Samter triad, which is characterized by the presence of aspirin sensitivity, CRSwNP, and asthma. Five different genes have been reported to be associated with CRS and aspirin-sensitive asthma.33 The gene most characteristic of the acetylsalicylic acid (ASA) phenotype was periostin (POSTN), which demonstrated upregulated expression in nasal polyp tissue in patients compared with controls. Also, the met proto-oncogene (MET) and protein phosphate 1 regulatory subunit (PP1R9B) were upregulated, whereas prolactin-induced protein (PIP) and zinc α2 glycoprotein (Azgp1) were downregulated. These candidate genes are believed to play a role in the pathogenesis of polyps associated with CRS and ASA.33
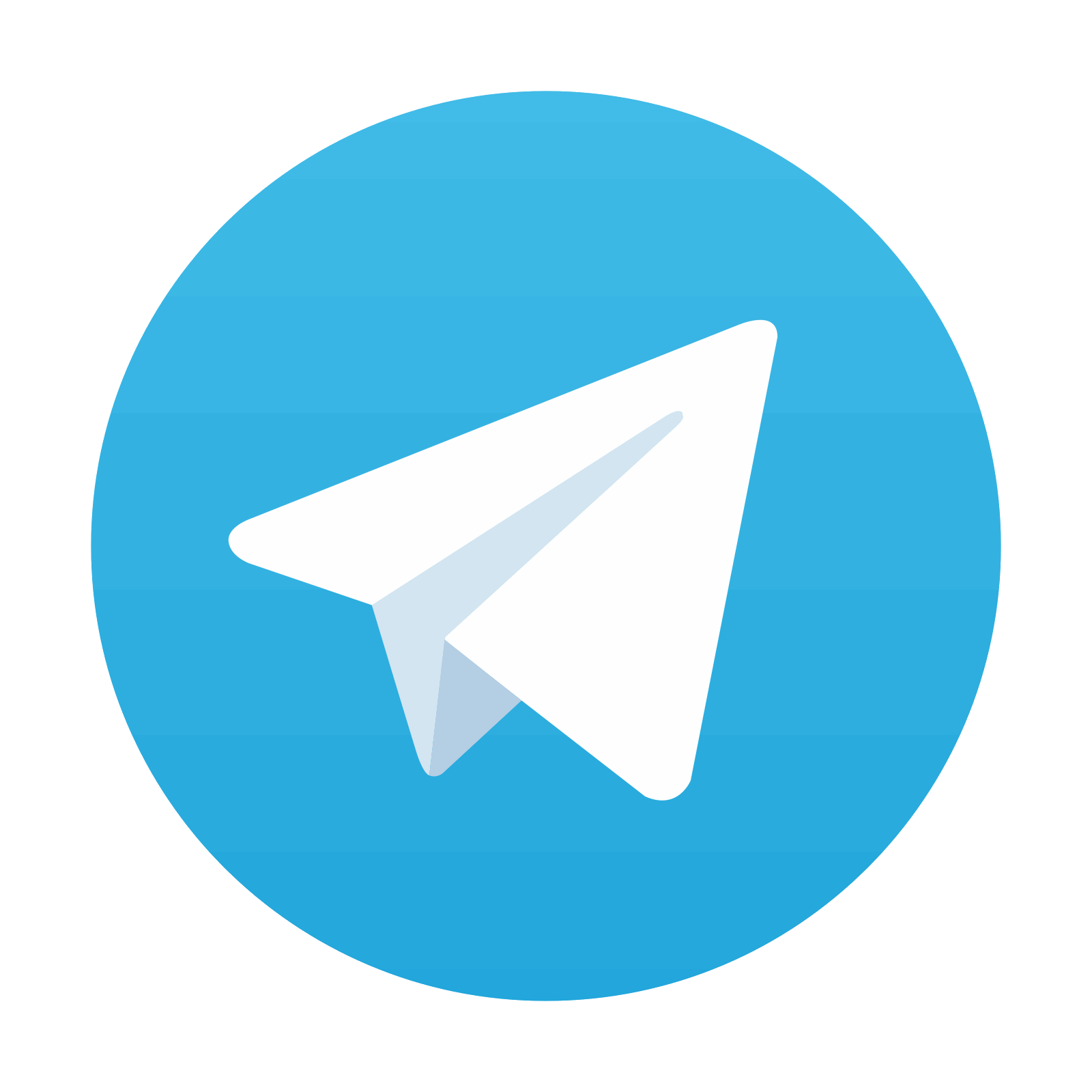
Stay updated, free articles. Join our Telegram channel

Full access? Get Clinical Tree
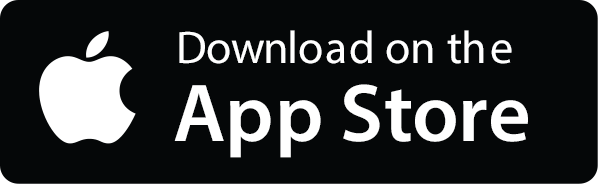
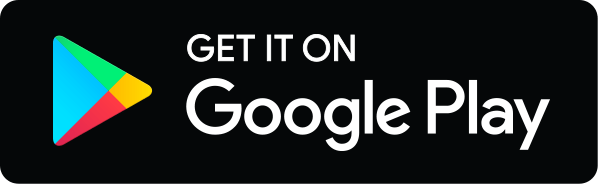