Imaging the Optic Nerve Head, Peripapillary, and Macula Regions in Glaucoma
Fabio Lavinsky, MD, MBA; Gadi Wollstein, MD; Lindsey Folio, MS, MBA; and Joel S. Schuman, MD, FACS
Glaucoma is the second leading cause of blindness worldwide.1,2 There is no current cure for this irreversible disease; therefore, it is best managed with early diagnosis and timely medical treatment. Glaucoma presents as an optic neuropathy, accompanied by characteristic vision loss and structural damage. Early methods of clinical glaucoma assessment included optic nerve head (ONH) examination, intraocular pressure (IOP) measurement, and visual field testing. However, these methods are limited because they are subjective and rely heavily on clinical interpretation. Additionally, it has been shown that 25% to 35% of the retinal ganglion cells (RGCs) are damaged before the appearance of visual field abnormalities.3,4 Recent advances in imaging technologies have greatly improved glaucoma management because they are capable of obtaining quantitative and reproducible measurements of the ONH, peripapillary and macula regions. However, in early stages of glaucoma, the functional abnormalities are often not detected even when changes in circumpapillary retinal nerve fiber layer (RNFL) are present. A “tipping point” was reported before in which there is structural changes without functional changes and after which there is a strong association between the two.5 Studies have shown imaging technologies offer a similar or improved glaucoma diagnostic ability to that of clinical evaluation of optic disc photographs.6–11 Current clinical glaucoma management combines both structural and functional evaluation to establish glaucoma diagnosis and detect progression.
This chapter will present a summary of methods for optic nerve, RNFL, and macula region evaluation in glaucoma and describe the different imaging modalities to assess glaucoma including confocal scanning laser ophthalmoscopy (CSLO), scanning laser polarimetry (SLP), and optical coherence tomography (OCT). The novel iterations of OCT technology and the expanded number of parameters available for glaucoma diagnosis, monitoring, and progression detection also will be described in this chapter.
HISTORICAL PERSPECTIVE
The first observation of the ONH was documented with the invention of the ophthalmoscope in 1851.12 Four years later glaucoma was detailed by von Graefe as consisting of excavation of the optic nerve.13 Schnabel was the first to relate nerve fiber loss to glaucoma in evaluating tissue cavities.14 However, it was not until 1922 that Fuchs and Elliot described in great detail the structural changes to the optic nerve caused by glaucoma.15 Fuchs observed a disappearance of anterior glial fibers, followed by deeper glial fibers, accompanied by bending backward and thinning of the lamina cribrosa. Fuchs noted that these ONH changes precede vision loss and coincide with an increased IOP. Since these observations, the gold standard in glaucoma evaluation has focused on ONH examination, IOP measures, and visual field tests. However, there are many limitations to these 3 non–imaging-based evaluation techniques. ONH examination can be performed using direct ophthalmoscopy, indirect ophthalmoscopy, and slit lamp biomicroscopy. The subjectivity associated with ONH visual examination presents as a limitation because the assessment can be inconsistent between visits and has been found to vary between clinicians.16 IOP is limited because of its wide diurnal variation, absence of IOP abnormality in a substantial percentage of glaucoma patients, namely patients with normal-tension glaucoma, and it does not directly assess the degree of damage. Visual field tests are shown to be inconsistent with repeated examination.17 Moreover, visual fields in early glaucoma may not show damage, such as the central points when assessed with 24-2 testing strategy in comparison with the 10-2 testing strategy.18 In advanced glaucoma, there is a reduction in reproducibility in points with threshold sensitivity below 19 dB.19 These limitations result in a great need for objective methods of glaucoma assessment, which was fulfilled by imaging devices.
Figure 9-1. Stereoscopic fundus photograph of a healthy individual.
Ocular imaging devices have become an integral part of clinical glaucoma assessment. They remove subjectivity by providing unmatched visualization of ocular structures and presenting quantitative data to aid in glaucoma diagnosis and monitoring disease progression. As first pointed out by von Graefe and Schnabel, glaucomatous damage appears most clearly in the optic nerve and RNFL. Therefore, imaging methods in glaucoma have primarily focused on quantifying the tissue morphology from these 2 regions. The evolution of OCT technology also enabled quantification of the macula, including the ganglion cell and the inner plexiform layers.20 These areas have quantifiable changes that were shown in longitudinal studies to be associated with functional glaucomatous damage.21
ASSESSMENT OF THE OPTIC NERVE HEAD
Precise examination and subsequent documentation of the ONH are critical components of glaucoma evaluation. The ONH is composed mainly of load-bearing connective tissues and neural tissues. The neural tissues are in the form of approximately 1 to 1.2 million RGC axons.22 These axons comprise the RNFL, which then converge to form the neuroretinal rim of the ONH. Damage that presents as cupping to the ONH with focal or diffuse loss of the neuroretinal rim provides an indicator of the state of health of the RGCs and the ONH connective tissues. Additionally, one of the postulated locations for the initial glaucomatous damage is at the point where the RGC axons intersect the lamina cribrosa mesh-like structure. This reinforces the necessity to accurately assess the ONH region in glaucoma evaluation. Fundus photography, CSLO, and OCT all provide reliable and reproducible methods to evaluate the health of the ONH.
Figure 9-2. Stereoscopic fundus photograph of a patient with glaucoma with an optic disc hemorrhage (arrow).
Stereoscopic Fundus Photography
A fundus camera is a special camera designed to effectively capture the inner workings of the eye, including the retina, optic disc, and macula. The benefit of the fundus camera over clinical ONH examination techniques, such as direct ophthalmoscopy, indirect ophthalmoscopy or slit-lamp biomicroscopy, is that it creates a snapshot at a given time point enabling qualitative and quantitative comparison with future exams (Figure 9-1). The stereoscopic feature allows a 3-dimensional (3D) fundus image to be captured, giving the clinician a more realistic view to assess cupping of the ONH tissues. Given that glaucoma is a slowly progressing disease, minor changes to the ONH can easily go unnoticed until damage reaches a severe level.
Stereoscopic fundus photography also offers visualization of certain features characteristic of glaucoma that are best seen with photos than with other imaging methods. This includes the characteristic optic disc pallor, or the ability to document disc hemorrhage (Figure 9-2), a common occurrence in glaucomatous eyes. Other important glaucomatous features usually seen with stereoscopic fundus photography are neural tissue loss, which leads to a global enlargement of the ONH cup or a localized loss seen as a rim notch, changes in blood vessels with baring of the circumlinear vessels and peripapillary atrophy with alpha (peripheral area of hypo- and hyperpigmentation) and beta (closer to the disc area of atrophy of the retinal pigment epithelium and the choriocapillaries) zones, all of which can be seen in glaucoma. For these reasons, fundus photography will continue to be an important component of glaucoma clinical management in the foreseen future.
The major limitation of fundus photography is the subjective component of the image assessment. Studies have shown substantial disagreement between glaucoma experts in glaucoma discrimination determined from stereophotos.6,23,24 Semiautomated quantification of ONH structures from the photographs is also available.25,26 Other approaches involve the presentation of the photographs in ways that enhance the ability to detect ONH changes.
Confocal Scanning Laser Ophthalmoscopy Optical Coherence Tomography
CSLO was first described by Webb and colleagues in 1987.27 A beam of light is directed at the retinal tissue of interest, and the back reflected light is detected by a sensor. To reach the sensor, the reflected light must pass through a set of 2 conjugated pinholes, located in front of the detector and adjacent to the light source. This method ensures that only light reflected from a specific focal plane is detected by the sensor.28 To acquire a 3D representation of the ONH, the focal plane is adjusted during image acquisition. These images obtained from the multiple parallel focal planes are then reconstructed to form one image that represents the topography of the ONH.
The most commonly used CSLO device is the Heidelberg Retinal Tomography (HRT; Heidelberg Engineering). The device uses a 670-nm wavelength light source to capture consequent and parallel 2-dimensional scans. The device is capable of acquiring scans with 10-μm transverse and 300-μm axial resolutions. To obtain quantitative parameters from the image, the user is required to define the ONH margin by marking a contour line surrounding the disc. Parameters abstracted from the image include disc area, rim area, rim volume, cup area, cup volume, and cup-to-disc ratios. CSLO measurements have been shown to be highly reproducible29 with improved sensitivity to discriminate between healthy and glaucomatous eyes than stereophotos (Figure 9-3).7
The HRT includes Moorfields Regression Analysis (MRA) and Glaucoma Probability Score (GPS), aiding in detecting glaucomatous ONH abnormality. MRA is comparing the predicted rim area, after accounting for the disc area and age, to the measured rim area globally and in 6 predefined sectors. The GPS software provides an operator-independent method to assess the ONH where there is no need to manually delineate the disc margin. This method relies on characteristic 3D configuration of the ONH region and automatically determines the probability of glaucomatous changes in this region.30
HRT provides a progression analysis, also known as Topographic Change Analysis (TCA). This assessment compares a baseline scan to follow-up images and accounts for possible variabilities between scans when reporting change (Figure 9-4). The variability is defined for each individual based on the first 2 baseline tests, and a consequent change that exceeds this variably will be marked as progression.
Limitations of CSLO include the operator dependence of the ONH margin’s location, the required reference plane to obtain quantitative measurements of the ONH that might vary between visits, and the inclusion of blood vessels as part of the rim, thus overestimating this structure while underestimating cup measurements.
At the time of this writing, the device is no longer available for purchase in the United States.
Optical Coherence Tomography
OCT is an imaging technique primarily used to evaluate the ONH, RNFL, and the macula. The first ophthalmic application of the technology was described by Huang et al in 1991.31 This technique is rapidly evolving with multiple iterations introduced for clinical use since its initial presentation. It is a noninvasive, noncontact technique that uses the differences in optical properties of tissue structures to create an “optical biopsy” (Figure 9-5).32
The most common OCT iteration at the time of this writing, spectral-domain (SD) OCT, also known as frequency-domain OCT, was first reported for ophthalmic use by Wojtkowski and colleagues in 2001.33,34 A broad-bandwidth, low-coherence light originating from a super-luminescent diode laser is projected toward the eye and divided into light projected toward a stationary mirror, contained in a reference arm, and toward the tissue of interest. The back reflected light is detected by a spectrometer that divides the interference pattern into its frequency components. Each frequency of light represents a different tissue depth; therefore, each axial scan (A-scan) can be acquired instantaneously. Current SD-OCT systems are capable of capturing images at a frame rate of more than 100,000 axial scans/s35 and axial resolutions on the range of 2 to 7 μm.36,37 The rapid scan speed of SD-OCT enables the creation of 3D images, allowing advanced visualization and sophisticated post-processing. One of these capabilities is the C-scan, or en face image, composed by projection image of scans perpendicular to the scanning axis that provide visualization of specific layers.
Several commercially available SD-OCT devices are offered, varying with the scan patterns and the parameters provided. Common ONH region scan patterns used by these devices include radial scans centered on the ONH in a spoke-pattern configuration, combination of radial and concentric circle scans, and consecutive parallel linear scans (raster scan). The radial scan pattern allows the acquisition of a limited number of linear scans with dense sampling in the center of the ONH but sparse sampling toward the periphery. The combined radial and concentric circles overcome the sparse peripheral sampling but suffer from reduced measurement reproducibility due to the time lapse between the acquisition of the radial and concentric scans. The raster scan pattern allows resampling at any location within the scanned region and 3D reconstruction (Figure 9-6). Quantitative parameters from SD-OCT ONH scans include (may vary between devices): RNFL, optic disc area, optic cup area, rim area, minimal rim width, nerve head volume, cup volume, rim volume, cup-to-disc area ratio, and local radial rim and disc measurements (Figures 9-6 through 9-8).38,39
Figure 9-3. HRT report with a healthy left eye and reduction of the rim area in the right eye. This report also shows cup and RNFL parameters.
The advantages of OCT include its ability to provide real-time visualization and quantification of retinal pathologies.40,41 Images and data obtained from OCT have been shown to be reproducible and provide powerful glaucoma discrimination capability.36,42–45 Studies have also shown that structural damage in OCT images correspond to visual field defects and histological retinal assessment.46–48 OCT and CSLO have been shown to be highly correlated in ONH assessment.49 Detection of glaucoma progression using parameters of ONH obtained with SD-OCT was also demonstrated to be useful.50
Figure 9-4. HRT progression analysis showing gradual loss in the inferior region of the optic nerve. Adjacent thinning of the RNFL is noticeable in the last 2 visits. Changes marked along blood vessels are usually disregarded as they reflect the various stages of the blood pulsation at the time of the image acquisition.
Limitations of OCT include imaging difficulty when ocular opacities are present and patient movement that can cause errors in data collection. Post-acquisition techniques, however, have been able to correct some motion artifacts by realigning 3D cubes. Several SD-OCT devices include a real-time eye movement tracking system that corrects for motion artifacts during image acquisition. Registration techniques can be used to match image locations from visit to visit. This aids in reducing intervisit variability, thus improving the ability to detect structural changes or progression over time. OCT progression software analysis will be discussed in the RNFL section below.
Figure 9-5. (A) CSLO image of the ONH. (B) Spectral-domain OCT B-scan across the ONH for a healthy individual (obtained with Spectralis HRA+OCT, Heidelberg Engineering).
Figure 9-6. ONH 3D cube scan for a healthy individual (obtained with 3D-OCT 1000, Topcon 3D Cube scan).
The lamina cribrosa has been suggested as an important site for axonal injury in glaucoma. Advanced OCT imaging modalities allow in vivo evaluation of lamina cribrosa–associated structures such as the prelaminar tissue thickness, lamina cribrosa thickness, localized defects, insertion depth, and posterior displacement (Figure 9-10).51–55 These parameters, together with the biomechanical characteristics of the lamina cribrosa and its relationship with IOP, are potential biomarkers for glaucoma diagnosis and disease progression that are currently being explored.56,57
ASSESSMENT OF THE RETINAL NERVE FIBER LAYER AND THE GANGLION CELL LAYER
Glaucoma is associated with damage to the RGCs and their axons that form the RNFL on their way toward the optic nerve.36 RNFL photography is obtained using a red-free, blue or green light that are highly absorbed by the retinal pigment epithelium and choroid, while the axon bundles reflect the light and appear as silvery striations. It permits evaluation of abnormalities of the RNFL such as focal (wedge defects) and diffuse RNFL damage. Quantitatively measuring structures related to these tissues will therefore closely assess the damage to the ganglion cells. RNFL measurements obtained with imaging devices, such as SLP and OCT, have shown excellent glaucoma discrimination ability and provide important information regarding disease progression.58,59
Figure 9-7. ONH and RNFL analysis obtained with Cirrus HD-OCT (Zeiss). Circumpapillary B-scan with global, quadrant, and clock hour RNFL thickness measurements with color-coded comparison with the normative data (obtained with Cirrus HD-OCT Optic Disc Cube 200×200 scan) are shown demonstrating more prominent abnormal ONH parameters and significant RNFL thinning in the left eye compared to the right eye.
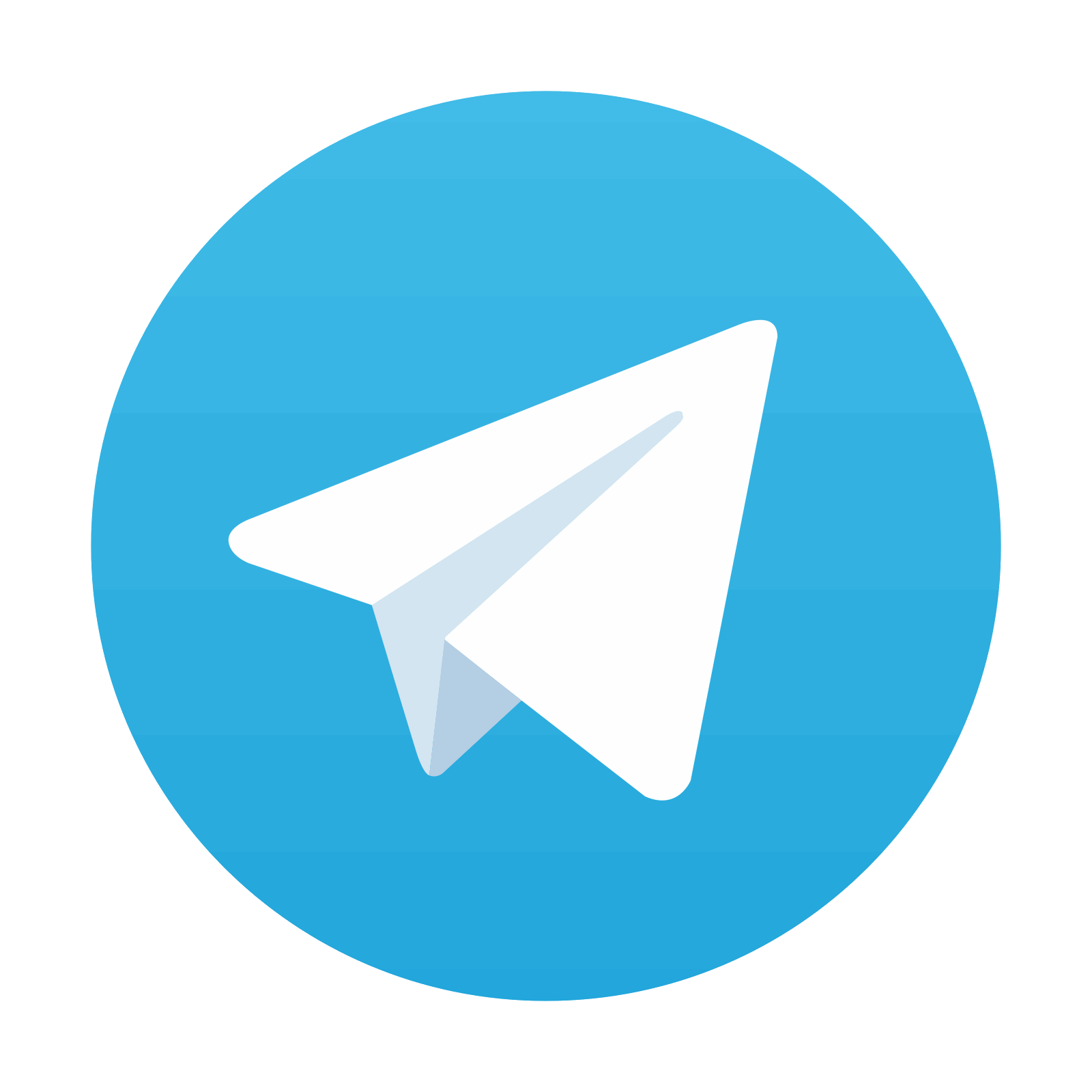
Stay updated, free articles. Join our Telegram channel

Full access? Get Clinical Tree
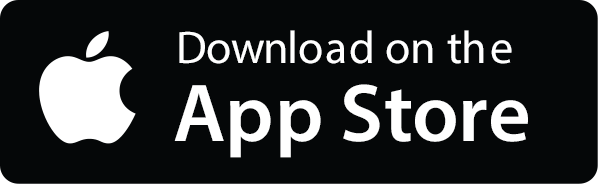
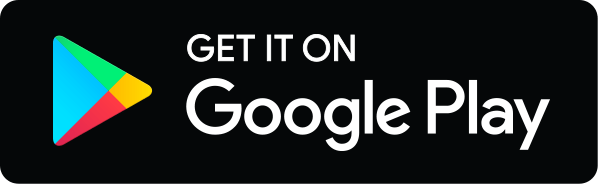