Fig. 15.1
Axial (a) and coronal (b) fluid-attenuated inversion recovery magnetic resonance images of the brain from a 61-year-old patient with migraine without aura. Multiple hyperintense, small, punctuate lesions are visible in the deep and juxtacortical white matter
Several theories have been considered to explain the increased susceptibility to form WMls of migraine patients. Some authors have suggested that hemodynamic changes detected during the attacks, including oligemia and focal hypoperfusion, may lead to such a damage [10, 11]. Other mechanisms include atherosclerotic causes, such as common cardiovascular risk factors, endothelial dysfunction, medications with vasoconstrictor activity taken to treat headache (e.g., triptans), local excessive neuronal activation, and cardiac abnormalities including patent foramen ovale (PFO) [11]. The association between WMls and PFO in patients with migraine has been the argument of several investigations, with controversial results. Indeed, some studies have reported that migraine patients, especially those with MWA, had a higher prevalence of PFO and right to left shunt (RLS) than non-migraineur controls with a relationship between these cardiac abnormalities and WMls [12], whereas other studies did not confirm these results [10].
Neurogenic inflammation has also been considered in the pathogenesis of WMls of migraineurs. Increased neuropeptides and proinflammatory cytokines have been reported during ictal and interictal periods in these patients [10]. In addition, in many cases the imaging features of WMls of migraine patients resemble those of WM lesions of patients with multiple sclerosis (MS), satisfying MS diagnostic criteria for disease dissemination in space in up to 34 % of the patients [13, 14]. Clearly, these patients with migraine and multiple WMls may represent a diagnostic challenge, particularly when neurological symptoms (even if reversible) and/or signs are present. A recent study has shown that double inversion recovery sequences may provide important pieces of information in the diagnostic workup of these patients, since, differently from what is usually observed in MS, no cortical lesions are detected with these techniques in migraineurs [13].
The definition of how early WMls occur in patients with migraine and the evaluation of their rate of progression over time may provide further insights into the mechanisms responsible for their formation. Recent studies in pediatric patients with migraine have demonstrated that WMls occur in a relatively high proportion of these patients (more than 30 % in some studies) [15], have imaging features similar to those of adult patients, and are not associated with stroke, migraine type, the presence of PFO, or the degree of RLS [16, 17]. Recent longitudinal studies have shown that the rate of progression of WMls, in both adult [18] and pediatric [17] patients with migraine, is similar to that found in individuals without migraine.
The clinical relevance of WMls in migraine patients remains unknown. Camarda et al. [19] have suggested a potential association between the presence of executive deficits and WMls in migraine patients. However such an association has not been confirmed by other investigations [20].
15.3 MRI and Pathophysiology of Migraine
15.3.1 MR Imaging of Cerebral Vasculature
Early MRI studies have supported the conceptualization of migraine as a vascular disorder. Numerous MR angiography (MRA) studies have shown that patients who undergo imaging examinations during an aura characteristically have hypoperfusion associated with vasoconstriction, whereas those who undergo imaging examinations during the headache phase show vasodilatation and hyperperfusion. Decreased cerebral blood flow (CBF) and venous dilatation during a migraine attack have also been confirmed using susceptibility-weighted imaging (SWI) [2]. However, other studies have suggested that modifications of vascular diameter might not occur, or might not be required, during migraine pain [2, 21].
15.3.2 Functional Imaging Techniques
Functional imaging techniques allow one to assess hemodynamic abnormalities in migraine patients and are not only improving the understanding of the mechanisms responsible for initiation and propagation of migraine attacks but also contributing to shed light on the cyclic aspect of the disease and the modifications in cortical and subcortical regions during the different phases of migraine.
15.3.2.1 Positron Emission Tomography and Single Photon Emission Computerized Tomography
Positron emission tomography (PET) and single photon emission computerized tomography (SPECT) are two imaging techniques that use radiolabeled molecules to provide information about function and metabolism of different tissues. These techniques are based on the detection and quantification of gamma rays emitted indirectly by a radionuclide (tracer), which is introduced into the body on a biologically active molecule. Three-dimensional images of tracer concentration within the body are then constructed by computer analysis. Using both SPECT and PET, a focal reduction of regional CBF (rCBF) in the occipital cortex was reported in migraine patients during the aura phase. Posterior cerebral hypoperfusion has been reported also in migraine patients without aura (MWoA) during a spontaneous migraine attack [2]. Hypoperfusion in migraine patients is not restricted to posterior regions of the brain, but may also involve regions located in the frontal and temporal lobes, which have been variously implicated in nociception [22].
By defining the patterns of neuronal activation involved in migraine, numerous PET studies have contributed to move from a purely vascular hypothesis of migraine pathophysiology to a neurovascular or a CNS theory. An important early study revealed an increased blood flow in the brainstem, cingulate, auditory, and visual association cortices during spontaneous migraine attacks. However, only the contralateral brainstem activation persisted after relief from headache and phono- and photophobia induced by sumatriptan injection, thus suggesting a role of brainstem nuclei (involved in antinociception) in the pathogenesis of migraine [23]. The activation of brainstem nuclei, in particular in the midbrain and dorsal pons, has been confirmed by subsequent studies in both episodic and chronic migraine, thus supporting its role as “migraine generator” [2, 3].
Repetition of migraine attacks leads to metabolic abnormalities of brain regions belonging to the central pain matrix, such as the insula, anterior and posterior cingulum, and prefrontal and primary somatosensory cortices. Indeed, hypometabolism of these regions has been negatively correlated with disease duration and lifetime headache frequency [2].
The application of PET to investigate patients with medication-overuse headache (MOH) [5] has allowed to demonstrate that metabolic abnormalities may be reversible, since abnormal metabolic activity of cerebral regions involved in pain processing recovered to almost normal levels after the medication was withdrawn. The only exception was found in the orbitofrontal cortex, which showed a further reduction of activity after medication withdrawal, indicating a role for this structure in the predisposition to analgesic overuse.
Brain metabolism alterations in patients with vestibular migraine have been studied only recently using PET in two patients during and between attacks [24]. Compared with interictal images, ictal PET showed increased metabolism in the bilateral cerebellum, frontal and temporal cortices, posterior insula, and thalami and deactivation of the bilateral posterior parietal and occipitotemporal areas. These results suggest that patients with vestibular migraine activate the vestibulothalamo-vestibulocortical pathway during the migraine attack. In addition, decreased metabolism in the occipital cortex may represent reciprocal inhibition between the visual and vestibular systems.
15.3.2.2 Perfusion MRI
Brain tissue perfusion can be estimated using either exogenous tracers (e.g., gadolinium chelates) or endogenous arterial water (arterial spin labeling – ASL). Several perfusion-weighted MRI studies have reported a reduced rCBF in brain regions contralateral to the side of aura symptoms (e.g., occipital regions contralateral to the affected visual hemifield during visual aura) and a subsequent cerebral hyperperfusion during pain in migraine patients [2, 25, 26]. Such perfusion abnormalities regress over time without permanent sequelae or cerebral ischemia on follow-up [26, 27]. Studies of interictal patients showed both hyper- and hypoperfusion of brain areas that are not specific for migraine pathophysiology [2].
15.3.2.3 Functional MRI
Functional (f) MRI is a noninvasive technique which allows to study (CNS) function and to define abnormal patterns of activation and/or functional connectivity (FC) caused by injury or disease. The signal changes seen during fMRI studies depend on the blood oxygenation level-dependent (BOLD) mechanism. Local increases in neuronal activity result in rise of blood flow and oxygen consumption. The increase of blood flow is greater than the oxygen consumption, thus determining an increased ratio between oxygenated and deoxygenated hemoglobin, which enhances the MRI signal [28]. By analyzing these data with appropriate statistical methods, it is possible to obtain information about the location and extent of activation as well as connectivity of specific areas involved in the performance of a given task in healthy subjects and in patients with different neurological conditions. Recently, a completely task-free approach, based on the assessment of functional correlations of neural networks at rest (resting state [RS] fMRI), has been developed (for a review see [29]).
In line with PET investigations, several fMRI studies have contributed to the classification of migraine as a neurovascular or even a brain disorder. The pioneering study by Hadjikhani and coworkers [30] has shown that during induced and spontaneous visual aura, a focal increase of BOLD signal (possibly reflecting vasodilation) developed within occipital extrastriate cortex (area V3A) (Fig. 15.2). This BOLD change progressed contiguously and slowly over the cortex, congruent with the retinotopy of the visual percept, and, then, following the same retinotopic progression, it diminished (possibly reflecting vasoconstriction). This spreading signal disturbance had striking similarity with cortical spreading depression (CSD) phenomenon, thus supporting the theory that CSD was the electrophysiological correlate of visual aura. Notably, a spreading neural activity suppression has also been described in MWoA patients during triggered migraine attacks [31].
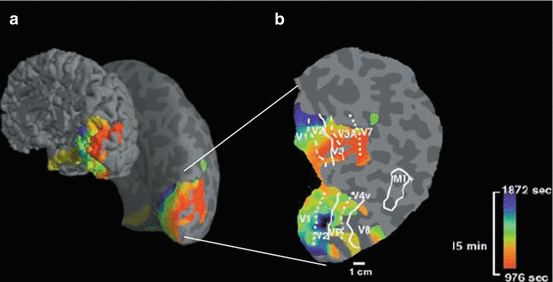
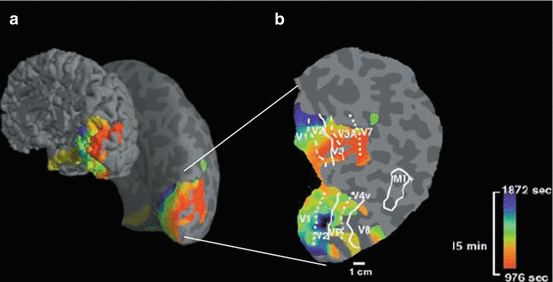
Fig. 15.2
Source localization and time of onset of the blood oxygenation level-dependent (BOLD) signal changes during induced and spontaneous visual aura attack in patients with migraine: (a) data are represented on inflated cortical surface shown from a posterior-medial view; (b) a fully flattened view of the cortical surface of the involved region. Cortical regions showing the first BOLD perturbations are coded in red (according to the color-coded scale representing variation in time), and locations showing the BOLD perturbations at progressively later times are coded by green and blue (according to the color-coded scale representing variation in time). The aura-related changes appeared first in extrastriate cortex (area V3A) and then progressed contiguously and slowly over the cortex following the same retinotopic progression of visual disturbance (Reproduced with permission from Hadjikhani et al. [30]. Copyright (2001) National Academy of Sciences, USA)
FMRI studies have also confirmed dysfunctional activity of brainstem nuclei involved in pain modulation during both the ictal and interictal phases [2]. Stankewitz et al. [32] confirmed an increased activation of the dorsal pons in migraine patients during induced migraine attacks. This study also revealed a selective gradient-like activity in the spinal trigeminal nucleus: after trigeminal nociceptive stimulation, interictal migraine patients exhibited lower activations of this nucleus; however, shortly before the migraine attack, patients had an increased activation at this level. Of interest, the time interval to the next headache attack could be predicted by the amplitude of signal intensities in the spinal trigeminal nuclei, suggesting that this oscillating behavior may represent a key phenomenon in migraine pathogenesis.
Studies which have explored cerebral activity within the pain network in migraine using experimental pain stimulation have shown abnormalities of a widespread subcortical and cortical brain network involved in pain processing in these subjects. However, one of the main challenges in the interpretation of these results is to differentiate findings consistent with a general pain response from those that might be specific to migraine [33]. Using a contact thermode as a noxious stimulation paradigm, migraine patients were found to exhibit a greater activation in the anterior cingulate cortex at 51 °C and less activation in the bilateral somatosensory cortex at 53 °C [34], thus supporting the presence of an increased antinociceptive activity in these patients, which could represent a compensatory functional reorganization aimed at modulating pain perception to the intensity of healthy controls. Other fMRI studies have demonstrated alterations in pain modulatory/inhibitory circuits, which may be related to the lack of habituation after repetitive painful stimulation and increased cortical excitability to painful stimuli that may lead to the development of allodynia [3]. The thalamus is now considered to play a pivotal role in the manifestation of allodynia. Burstein et al. [35] showed that brush and heat stimulation at the skin of the dorsum of the hand produced larger BOLD responses in the posterior thalamus of patients undergoing a migraine attack with extracephalic allodynia than the corresponding responses registered when the same patients were free of migraine and allodynia.
An increased activation of cortical regions mediating the affective dimension of pain has also been demonstrated in migraineurs. During spontaneous and induced migraine, these patients had increased BOLD signal intensities in limbic structures (e.g., the amygdala and insula) and exhibited a stronger recruitment of affective cortical areas when exposed to emotional inputs [3]. Based on these data, a model of migraine as a dysfunction of a “neurolimbic” pain network has been proposed [36].
Abnormalities of function of pain-processing regions have also been investigated in patients with chronic migraine, particularly those with MOH. Before the withdrawal of the offending medications, these patients had reduced pain-related activity in areas of the lateral pain pathway, including the primary and secondary sensorimotor areas. Such abnormalities regressed after treatment withdrawal [37]. In addition, patients with MOH presented dysfunctional activity of the meso-cortico-limbic dopamine circuit, including the ventromedial prefrontal cortex and the substantia nigra/ventral tegmental area complex, during the execution of a decision-making under risk paradigm. The ventromedial prefrontal cortex dysfunctions were reversible and attributable to the headache, whereas the substantia nigra/ventral tegmental area complex dysfunctions were persistent despite treatment withdrawal, suggesting that MOH may share some neurophysiological features with addiction [3].
It is well established that migraine patients show also hyperresponsiveness of the primary visual cortex and a lack of habituation to visual stimuli [2]. These phenomena are more pronounced in patients with MWA [10]. Hyperresponsiveness of the visual cortex in migraine extends beyond primary visual areas, even in the interictal period. Antal et al. [38] demonstrated significantly stronger activation of the extrastriate, motion-responsive MT area, representing the medial-superior temporal area, in migraine patients versus healthy controls in response to coherent/incoherent moving dot stimuli. This cortical hyperexcitability may represent the biological basis for the clinical observation of heightened vulnerability to motion sickness that migraine sufferers often report [2].
Numerous studies provided a conceptual framework for understanding vestibular migraine as a variant of MWA produced by the convergence of vestibular information within migraine circuits. Several fMRI studies showed that vestibular stimulation activate cerebral regions that are generally involved in migraine and pain perception, such as the posterior and anterior insula, orbitofrontal cortex, and the posterior and anterior cingulate gyri, thus suggesting that central constituents of the migraine circuit might include components of central vestibular pathways [5]. However, so far, fMRI has not been applied yet to investigate functional cortical abnormalities in patients with vestibular migraine.
RS fMRI studies have shown that FC is generally increased in pain-processing networks in migraineurs, whereas it is decreased in pain modulatory circuits [39]. In particular, migraineurs with a history of allodynia exhibit significantly reduced RS FC between PAG, prefrontal regions, and anterior cingulate cortex compared with migraineurs without allodynia [40]. These RS FC abnormalities have been related to the frequency of migraine attacks and disease duration [39]. Significant abnormalities of RS FC occur also in affective networks [39], the default mode (DMN) [41], and the executive network [33].
15.3.3 Quantitative Structural and Metabolic MRI Techniques
The notion that patients with migraine might have structural abnormalities in association with the previously described functional imaging abnormalities is relatively recent and is prompting the extensive application of modern, quantitative MRI techniques. The results obtained by this effort have consistently demonstrated that, similar to what has been observed in other neurological conditions, brain damage in patients with migraine extends beyond abnormalities detectable with conventional MRI sequences.
15.3.3.1 Morphometric Techniques
Several approaches are currently available to define the regional distribution of morphometric abnormalities among different cohorts of subjects, including voxel-based morphometry (VBM) (which allows an automatic comparisons of regional WM and GM volumes, on a voxel-by-voxel basis) and surface-based morphometry (SBM) (which allows a more precise and direct measurement of cortical thickness [CT] and cortical surface area [CSA], on a vertex-by-vertex basis) [10]. As extensively reviewed elsewhere [42], while a pioneering VBM study [36] did not find any significant morphometric abnormality of WM and GM in patients with episodic migraine, several more recent studies question this negative finding by showing that, similar to other chronic painful conditions, migraine patients exhibit a reduction of GM volume [2], CT, and CSA [43] in a network of areas that are involved in pain processing, including the insula, cingulum, and frontal, parietal, and temporal cortices. In some of these studies, GM atrophy was significantly associated with longer disease duration, higher frequency of attacks and higher T2 lesion load (in those patients with WMls), thus supporting the view of migraine as a progressive disorder. However, this hypothesis is in part contradicted by the fact that migraine is a self-remitting disease, which usually resolves with age, and by the results of other VBM and SBM studies which found no correlation between morphometric abnormalities and the number of attacks, disease duration, and WM lesion load, which has led some authors to postulate that the observed cortical abnormalities might represent a phenotypic biomarker of the disease [43]. As a consequence, longitudinal studies are needed to clarify this point. At present, only one study has applied VBM to track longitudinal modifications of GM volume in MWoA patients and found significant reduction of GM volume of the superior frontal gyrus, orbitofrontal cortex, hippocampus, precuneus, and primary and secondary somatosensory cortices after 1 year, which were not associated with changes in headache activity parameters [44]. It is important to note that recent longitudinal studies have shown that the GM volume reduction found in chronic pain syndromes can be normalized by treatment [45, 46], suggesting that it may represent a reversible aspect of chronic nociceptive transmission.
Concomitantly with such a diffuse pattern of GM volume reduction, several morphometric studies in patients with migraine have also reported increased GM volumes in regions of the brainstem (such as the PAG GM and the pontine nuclei), regions involved in visual processing (such as the MT/V5 complex and V3A), and the primary sensorimotor cortices. Whether these abnormalities contribute to the triggering of migraine attacks, or, conversely, are the consequence of repeated attacks, is still a matter of debate [2].
A recent VBM study of pediatric patients suffering of migraine [15] has shown that morphometric abnormalities occur relatively early in the course of this disease, since GM atrophy of frontal and temporal regions involved in nociception was detected also in this population (Fig. 15.3). Notably, pediatric patients with migraine had also an increased GM volume of the right putamen, which was inversely correlated with disease duration, suggesting that the involvement of the putamen likely occurs early in migraine patients with a pediatric onset of the disease.
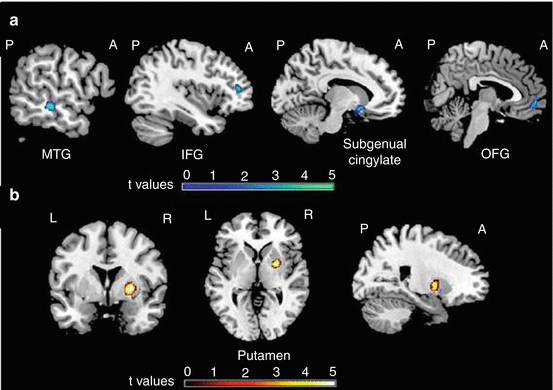
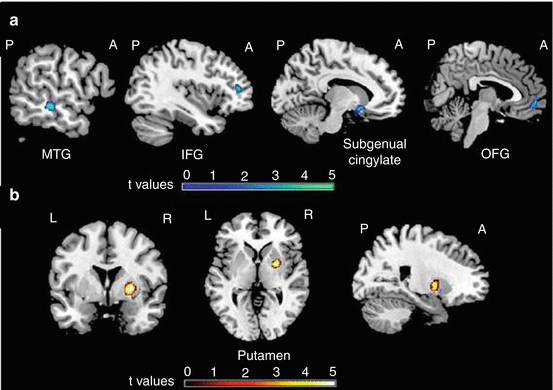
Fig. 15.3
Differences of regional gray matter (GM) volume between pediatric patients with migraine and healthy controls: (a) compared to healthy controls, pediatric patients had reduced GM volume of the left middle temporal gyrus (MTG), inferior frontal gyrus (IFG), subgenual cingulum, and right orbitofrontal gyrus (OFG) (blue-green scale according to t values); (b) compared to controls, pediatric patients had an increased GM volume of the right putamen (red-yellow scale according to t values). The GM differences have been superimposed on a high-resolution T1-weighted template (neurological convention) (Reproduced with permission from Rocca et al. [15])
The application of morphometric techniques is contributing to identifying structural correlates of some of the clinical deficits that are typically detected in migraineurs in clinical practice. For instance, significant correlation has been found between decreased GM volume of the frontal lobes and deficits of executive functions [2].
15.3.3.2 Diffusion-Weighted MRI
Diffusion-weighted (DW) MRI is a quantitative technique that exploits the diffusion of water within biological tissues [47]. The diffusion coefficient measures the ease of this translational motion of water. In biological tissues this coefficient is lower than that in free water because the various structures of the tissues (membranes, macromolecules, etc.) impede the free movement of water molecules [47]. For this reason, the measured diffusion coefficient in biological systems is referred to as the “apparent diffusion coefficient” (ADC) [47]. Pathological processes that alter tissue integrity typically reduce the impediments to free water motion, and, as a result, these processes tend to increase the measured ADC values. A full characterization of diffusion can be provided in terms of a tensor [48], which has a principal axis and two smaller axes that describe its width and depth. The diffusivity along the principal axis is also called parallel or axial diffusivity (AD), while the diffusivities in the two minor axes are often averaged to produce a measure of radial diffusivity (RD). It is also possible to calculate the magnitude of diffusion, reflected by the mean diffusivity (MD), and the degree of anisotropy, which is a measure of tissue organization that can be expressed by several indexes, including a dimensionless one named fractional anisotropy (FA).
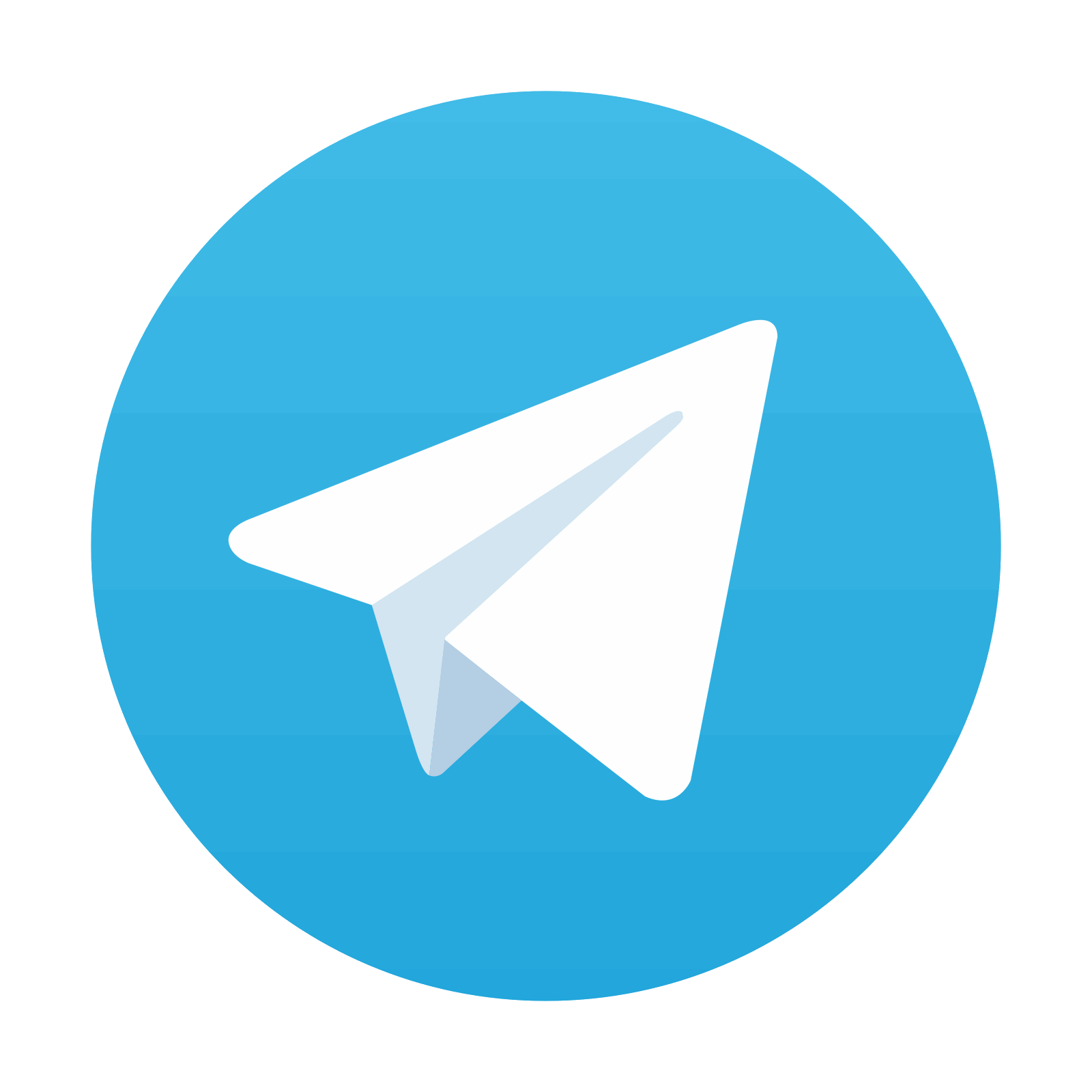
Stay updated, free articles. Join our Telegram channel

Full access? Get Clinical Tree
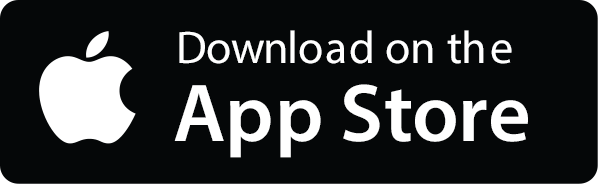
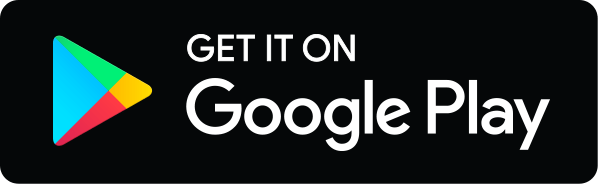