At a minimum, successful cochlear implantation requires that electrical impulses be delivered to a surviving spiral ganglion cell population, and that these impulses be transmitted to a functioning auditory cortex by an existent neural connection. Accordingly, imaging the auditory pathway of the implant candidate is necessary to screen for morphologic conditions that will preclude or complicate the implantation process. In addition to radiography, increasing resolution of computed tomography and magnetic resonance imaging technology has provided the clinician with more detailed information about the integrity of the auditory pathway.
Imaging and anatomy considerations for cochlear implantation
Radiographic imaging plays a major role in cochlear implantation with regard to preoperative candidacy evaluation, intraoperative monitoring, and postoperative evaluation, as well as research and experimental techniques. At a minimum, successful cochlear implantation requires that electrical impulses be delivered to a surviving spiral ganglion cell population, and that these impulses be transmitted to a functioning auditory cortex by an existent neural connection. Accordingly, imaging the auditory pathway of the implant candidate is necessary to screen for morphologic conditions that will preclude or complicate the implantation process. Increasing resolution of computed tomography (CT) and magnetic resonance (MR) imaging technology has provided the clinician with more detailed information about the integrity of the auditory pathway. As technologies evolve, a clear understanding of what information can be obtained as well as the limitations of various imaging modalities is essential to proper candidacy evaluation, and selection of the ear to be implanted in complex cases.
Preoperative imaging
Preoperative imaging is instrumental in determining the feasibility and facility of cochlear implantation. Analysis is preformed in a stepwise approach, answering of the following 3 questions. Are there cochleovestibular anomalies that preclude implantation? Is there evidence of luminal obstruction? Are there additional findings that may complicate the surgery or subsequent patient management? This section is not intended to review principles or techniques of image acquisition, but to provide a platform for discussion between the implant team and the radiologist.
Are There Cochleovestibular Anomalies that Preclude Implantation?
Approximately 20% of patients with congenital sensorineural hearing loss have radiographically identifiable morphologic abnormalities of the inner ear. In general, inner ear malformations can be associated with a wide range of hearing sensitivity. These patients can manifest progression of hearing loss, though many may retain useful hearing into adult life. As a general rule, however, the more severe is the deformity, the worse the hearing. Due to the variability and progressive nature of hearing loss in these disorders, most large implant centers are likely to evaluate several patients with a variety of malformations. Given the current technology, the minimum requirement for cochlear implantation is the presence of an implantable cavity in proximity to stimulable neural elements whose projections connect to the auditory cortex. Accordingly, the first question that must be answered is: are there any cochleovestibular anomalies that preclude implantation?
Embryology
To fully appreciate the wide variety of possible cochleovestibular malformations, it is helpful to first review the embryogenesis of the inner ear, considering separately the formation of the membranous labyrinth, the bony otic capsule, and the cochleovestibular nerves and ganglia.
The development of the combined cochlear and vestibular membranous labyrinthine system begins with the formation of the otic placode as an ectodermal thickening, which forms on the surface of the neural tube in the third gestational week. The otic placode invaginates from the surface and forms the otocyst in the fourth gestational week. The otocyst develops 3 infolds in the fifth week. The resultant pouches represent: the primordial endolymphatic sac and duct; the utricle and semicircular canals; and the saccule and cochlea. Beginning in the sixth week, the cochlear duct grows from its primordial bud beginning from the basal region spiraling apically to reach its full two-and-a-half to two-and-three-quarter turns by the eighth to tenth week. The neuroepithelial end organs continue to develop beyond this period, with the organ of Corti completing its formation in the 25th week.
The semicircular canals begin their formation as 3 small folded evaginations on the primordial vestibular appendage. The canals develop as disklike outpouchings whose centers eventually compress and fuse to ultimately form the semicircular duct structure. By the sixth week of gestational life, this compression and fusion has taken place in first the superior and then the posterior canals. The 3 canals continue to enlarge and complete their formation to full adult size in sequence, beginning with the superior around the 20th week, followed by the posterior, and finally the lateral semicircular canals. Of interest, the endolymphatic sac and duct are the first to appear and the last to complete their development.
The osseous otic capsule eventually forms from a morphologically fully developed cartilage precursor model via 14 centers of ossification, beginning around the 15th gestational week, and is completed during the 23rd gestational week. The cartilage model and underlying membranous labyrinth continue to grow in the region of the posterior and lateral semicircular canals while other structures, which have previously attained their final shape and size, have begun ossifying. The cochleovestibular nerves and ganglia develop in concert with the membranous labyrinth and cochleovestibular end organs. These structures are of neural crest origin and migrate between the epithelial layer and basement membrane of the otic vesicle during the fourth gestational week.
Cochlear malformations
There is much confusion in the literature regarding the nomenclature of cochlear morphologic anomalies, especially regarding the term “Mondini malformation.” In 1791, Carlo Mondini presented his findings on an anatomic dissection of a young deaf boy. According to his writings, prior reports of human deafness were attributed to abnormalities of the external auditory canal and Eustachian tube, tympanic membrane, middle ear and ossicles, or compression of the auditory nerve. During his dissection on the posterior face of the petrous bone Mondini discovered significant vestibular aqueduct enlargement, and commented that the usual bony lip that “protects the vestibular aqueduct” was missing and was substituted by a membranous plate of dura. He noted that the vestibule was not deformed but was of greater than usual size. He also noted an increase in the size of the elliptical recess, though it was normal in shape. He commented that the semicircular canals appeared normal and that the positions of their openings into the vestibule were unremarkable. In observing the medial opening of the vestibular aqueduct, Mondini commented that it was quite enlarged and was larger than the size of the common crus. With regard to the cochlea, it was described to possess only one-and-a-half turns. He described the cochlea as ending in a cavity corresponding to the last spiral turn and described an incompletely formed interscalar septum. The more contemporary term “incomplete partition” is commonly used to describe this classic anomaly, and denotes this specific aspect of the deformity. In this historic subject, the deformity was bilateral.
Because of its relative frequency as well as its historical significance, the term “Mondini malformation” is commonly used to describe all forms of cochlear morphologic abnormalities and not just the incomplete partition. The term Mondini dysplasia was used by Schuknecht in an in-depth analysis of the histopathology and clinical features of cochlear anomalies. Schuknecht’s treatise described a variety of malformations including one patient with “the normal 2 1/2 turns but measur[ing] only 23 mm in length (normal: 32 mm)” and another with “Mondini dysplasia limited to the vestibular system,” as well as several patients with cochleae possessing one-and-a-half turns, and other variant morphologies of both the cochlear duct and vestibular system. Schuknecht histologically described these malformations as isolated findings or in association with other named syndromes, namely Klippel-Feil, Pendred, and DiGeorge. His work detailed the clinical nature of these disorders as being unilateral or bilateral, and associated with acoustic and vestibular dysfunction, which is variable in severity, static, or progressive.
Phelps reserves the term “Mondini deformity” for cochlea whose basal turns are normal and that possess a deficiency of the interscalar septum of the distal one-and-a-half coils. He differentiates these cochleae from those termed “dysplastic” owing to their widened basal turn being in wide communication with a dilated vestibule. According to Phelps, the significance lies in the clinical absence of spontaneous cerebrospinal fluid (CSF) leak and meningitis in patients with his strict definition of Mondini deformity, as opposed to those patients with dysplasia who did manifest these complications in a series of 20 patients studied.
Since the writings of Mondini, several investigators have documented a variety of inner ear malformations. Though not the first to describe or name these malformations, Jackler and colleagues proposed a classification system in 1987 for the congenitally malformed inner ear based on the theory that a variety of deformities result from arrested development at different stages of embryogenesis. These investigators clearly stated that their classification could not describe all observable abnormalities but was meant to serve as a framework on which other describable anomalies could be added, which by their supposition would have resulted from aberrant, rather than arrested development.
This body of work deserves mention, as it is often cited and serves well as an initial systematic basis for the interpretation of images. Jackler and colleagues formulated their classification system on review of polytomes and CT scans of 63 patients with 98 congenitally malformed ears, and provided the categorization listed in ( Box 1 ). The disorders identified as having normal cochleae were subdivided solely for the purposes of Jackler’s classification scheme. It is important to realize that disorders of the vestibule, semicircular canals, and vestibular aqueduct are also often found in conjunction with cochlear malformations. Inner ear deformities tend to occur bilaterally in 65%. When bilateral, there is a 93% chance that they will be similar, although various combinations of morphologic classes have been documented.
Absent or Malformed Cochlea
- 1.
Complete labyrinthine aplasia
- 2.
Cochlear aplasia
- 3.
Cochlear hypoplasia
- 4.
Incomplete partition
- 5.
Common Cavity
Normal Cochlea
- 1.
Vestibule—lateral semicircular canal dysplasia
- 2.
Enlarged vestibular aqueduct
Complete labyrinthine aplasia, also called Michel deformity, could result from arrest prior to formation of the otocyst, resulting in complete absence of inner ear development. This malformation is the rarest among those classified here ( Fig. 1 ).
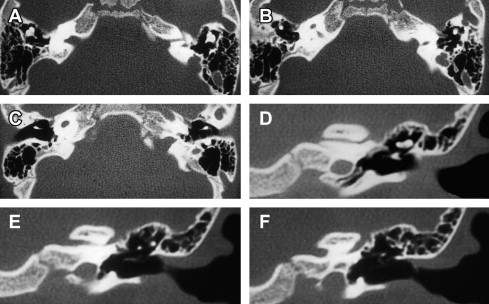
Cochlear aplasia is defined as absent cochlea with an intact but often variably deformed vestibular labyrinth. This cochlear malformation is the second rarest noted by Jackler and colleagues, representing approximately 3% of identified cochlear malformations.
The term cochlear hypoplasia has been used to describe a range of abnormalities from a rudimentary cochlear diverticulum to an incompletely formed cochlear bud of several millimeters ( Fig. 2 ). This group comprised 15% of cases reported by Jackler and colleagues, was believed to represent arrested development during the sixth gestational week, and may be associated with either a normal or malformed vestibule and semicircular canals.
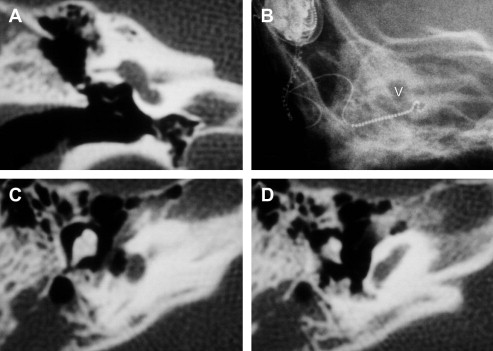
Incomplete partition is a term used by Jackler and colleagues in their study, and it is pointed out that this is the closest to the malformation originally described by Mondini. This cochlear abnormality is the most commonly described, making up 55% of the study described. It is believed to represent arrest in development during the seventh gestational week, a time at which the cochlea would have completed one to one-and-a-half turns. Radiographically, these cochleae possess only one-and-a-half turns comprising a basal turn leading to the appearance of a confluent middle and apical turn, which may also be viewed and described as incomplete partitioning by a deficient interscalar septum. These cochleae may also manifest varying degrees of abnormalities of the vestibular system and endolymphatic duct and sac ( Fig. 3 ).
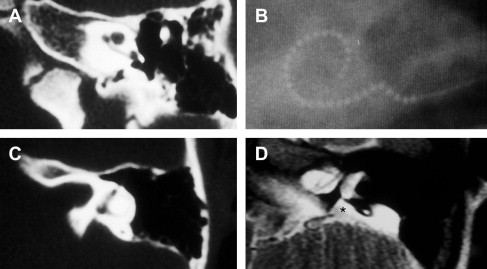
The term common cavity is used to denote confluence of the cochlea and vestibule into a common rudimentary cavity that usually lacks an internal architecture and is often associated with abnormally formed semicircular canals. This abnormality is the second most common described, and comprised 26% of the study by Jackler and colleagues ( Fig. 4 ).
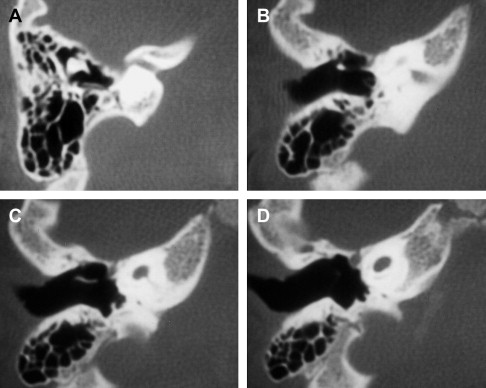
The classification scheme proposed by Jackler and colleagues is not all-inclusive. There are varieties of disorders that may be encountered that defy classification, as the investigators well noted. A very narrow internal auditory canal of a diameter 2 to 2.5 mm or less on either conventional tomography or CT has been reported in association with a normal inner ear as well as a variety of inner ear malformations. This condition has been reported unilaterally and bilaterally, in association with a variety of other congenital anomalies and as an isolated disorder. The clinical significance of this finding with regard to preimplant evaluation is that there is a high likelihood that this represents the presence of only a facial nerve and the absence of the cochleovestibular nerve. A CT scan demonstrating an internal auditory canal of less than 2 to 2.5 mm is considered by many investigators to be an absolute contraindication to cochlear implantation. Evaluation of the contents of the contents of the internal auditory canal using MR imaging may be warranted in selected patients, as increased experience is being gained with high-resolution scanning techniques.
There is also a particular form of X-linked deafness that has been both radiographically described and genetically identified. It is seen in some severely deaf males who possess a deficiency of bone between the lateral end of bulbous internal auditory canal and the basal turn of the cochlea. It has been detailed by both CT and MR imaging, and holds the clinical implications that there is an obvious large communication between the CSF-containing internal auditory canal and the cochlea. This situation also presents the concern that a multichannel electrode array may be introduced into the internal canal at the time of implantation.
In summary, the specific terminology used is less important than the detail in which reported cases are described with regard to radiographic and histologic features of each element of the inner ear: specific cochlear morphology; size and relation to the vestibule; patency of the bony modiolus; and the nature of inner ear aqueducts. Jumping to conclusions regarding their association with various clinical features such as hearing, implantation outcome, and complications may in this way be avoided.
Patient evaluation
Initial radiologic evaluation of the cochlear implant candidate is typically performed with high-resolution CT scanning. Patients with a malformed inner ear or narrow internal auditory canal may undergo supplemental MR imaging. MR imaging of inner ear malformations requires different parameters to those commonly used in the evaluation of adult hearing loss. The acquisition of appropriate images requires higher resolution and magnets of greater strength. Intravenous contrast is rarely used. The reasons for obtaining MR imaging in a patient with an inner ear anomaly are twofold: identification of nonosseous partitioning of the malformed cochlea and identification of the neural structures contained within the internal auditory canal ( Fig. 5 ). CT and MR studies are only macroscopic evaluations of the cochleovestibular apparatus; form does not necessarily imply function. Evidence of the existence of a stimulable auditory neural pathway, either by documentation of prior or residual hearing or by use of promontory stimulation testing, predict a more favorable outcome ( Figs. 6 and 7 ).
A few cochleovestibular anomalies do preclude implantation. Complete labyrinthine aplasia would be an absolute contraindication for implantation on the affected side. The determination of cochlear aplasia should involve the careful differentiation from a common cavity deformity by a combination of MR imaging and promontory stimulation in selected patients, to evaluate the possible presence of an adjacent stimulable cochlear nerve ganglion cell population. The failure to identify a cochlear nerve by high-resolution MR imaging would also contraindicate implantation regardless of the presence of an implantable cavity.
With careful patient selection and preoperative planning, using the various imaging and electrophysiologic testing modalities available, and employing experienced device programmers, many patients with a variety of cochlear malformations have been successfully implanted.
Is There Evidence of Luminal Obstruction?
In the absence of morphologic contraindications to implantation, the next question that must be answered is: is there any evidence of luminal obstruction? Inner ear inflammation, abnormalities of bone metabolism or trauma, may ultimately result in luminal obstruction either by ingrowth of fibrous scar tissue or pathologic neo-ossification. The origin most commonly encountered, especially in pediatric cochlear implant candidates, is postmeningitic labyrinthitis ossificans. Other postinflammatory causes include suppurative labyrinthitis secondary to otitis media or cholesteatoma, and hematogenous infections (septicemia, mumps, rubella, or other viral infections). Metabolic bone disorders include otosclerosis and Paget disease. Common posttraumatic causes include labyrinthectomy and temporal bone fractures. Wegener granulomatosis and autoimmune inner diseases such as Cogan syndrome have also been reported to result in labyrinthine ossification.
Bacterial meningitis is the most common cause of acquired severe sensorineural hearing loss in children. In retrospective analyses some degree of hearing loss has been reported to develop in 7% to 29% of survivors of meningitis. Deafness may follow bacterial meningitis in children in 2% to 7% of cases, with 1.5% being severe and bilateral. The organisms commonly responsible for postmeningitic deafness are Haemophilus influenzae and Streptococcus pneumoniae . Neisseria meningitidis is also a causative organism, though is believed to result in a lower incidence of postinfectious deafness. Although most series report H influenzae as the leading causative organism in most meningitic deafness, it is of note that a greater proportion of children surviving pneumococcal meningitis (33%) develop hearing loss, as opposed to H influenzae type b (9%) or meningococcal meningitis (5%). Pneumococcal meningitis, which presents a gram-positive exotoxin, is additionally associated with severe ossification, whereas the ossification associated with Haemophilus is generally less severe, owing to the effects of endotoxins that may be diminished by corticosteroids. Some degree of cochlear neo-ossification may be encountered intraoperatively in as many as 70% of patients deafened by meningitis. In series including all causes of deafness, some degree of basal-turn cochlear neo-ossification has been reported in approximately 15% of adult patients and in as many as 28% to 35% of pediatric patients.
Pathophysiology of labyrinthine ossification
The cochlear aqueduct is a bony channel that connects the subarachnoid space of the posterior cranial fossa to the scala tympani. It opens adjacent to the round window and is lined with a loose network of fibrous tissue termed the “periotic duct,” which is an extension of the arachnoid. This location is believed to be the site of origin of the inflammatory process into the inner ear in cases of meningitis. Other possible routes include the internal auditory canal and modiolus, the middle ear windows secondary to otitis media, lateral canal fistulization secondary to chronic inflammatory processes, trauma, and hematogenous spread. When encountered, ossification is nearly always most severe in the region of the round window and proximal scala tympani in the basal turn, adjacent to the opening of the cochlear aqueduct. The middle and apical turns are less commonly affected and the scala vestibuli is often spared. Because most cases of labyrinthitis ossificans are partial and the extent of obstruction commonly manifests asymmetrically within an individual patient, preoperative imaging plays an essential role in selection of which side to implant. Total cochlear ossification may occur, and is more commonly seen in children than in adults.
Cochlear ossification following meningitis is associated with a severe loss of cochlear hair cells as well as a decreased spiral ganglion cell population. There is no clearly predictable relationship between the extent of ossification and the number of injured spiral ganglion cells. Hinojosa and colleagues studied the temporal bones of deaf patients with labyrinthitis ossificans, and found that the remaining neuronal cell population ranged from 6310 to 28,196 with a mean of 17,152; this in comparison with the total cochlear neuronal population of approximately 35,500 in the human infant. Linthicum and colleagues studied the postmortem effects of implants on neuronal population and found that benefit may occur with as few as 3300 neurons. Cochlear ossification does not contraindicate cochlear implantation per se; it does, however, complicate electrode insertion.
There are several theories regarding the pathogenesis of labyrinthine neo-ossification. In 1936 Druss described two types of new bone: metaplastic bone originates from ingrown fibrous scar or connective tissue while osteoplastic bone originates from the adjacent otic capsule after disruption of the endosteum. Postlabyrinthitis ossification is thought to occur via the metaplastic process. During the initial acute stage of infection, bacteria within the perilymphatic spaces induce an acute inflammatory reaction characterized by leukocyte infiltration and fibroblast proliferation. Labyrinthine fibrosis is considered to be the early stage of ossification, and may occur within weeks of initial infection. Ossification eventually ensues, and this is termed the osseous or late stage of labyrinthitis ossificans. According to Suigiura and Paparella, undifferentiated mesenchymal cells originating in the endosteum, modiolar spaces, and basilar membrane likely differentiate into fibroblasts and either subsequently or directly into osteoblasts, and form local or diffuse osseous deposits.
Several investigators have postulated that the pathogenesis of metaplastic bone formation may be related to disruptions of cochlear blood supply, which has been demonstrated experimentally and observed histologically in the temporal bones of patients having undergone a variety of surgical procedures. This theory has been claimed to be supported by cell culture experiments performed by Gorham and Test, in which low oxygen tension favors bone formation while high oxygen tension favors osteoclastic resorption. Additional investigators have commented on the similar findings between the ossification of vascular occlusion and those of suppurative labyrinthitis.
The two types of neo-ossification were further characterized in histologic studies performed by Kotzias and Linthicum on human temporal bones with a variety of pathologic processes including patients who had undergone a variety of neuro-otologic procedures. The metaplastic form is characterized by high cellularity and the relative absence of eosinophilia. There are no osteoblasts on the surface. Although its margins are indistinct, it is confined to the lumen of the cochlea. The osteoplastic form occurs only when there has been disruption of the endosteum, such as occurs during trauma or a surgical defect. It is characterized by less cellularity and increased eosinophilia, and is characteristically lamellar in form, with clear margins and osteoblasts on the surface and not clearly distinct from the endosteal layer.
The postmeningitic neo-ossification is thought to occur via the metaplastic process with the ectopic bone being typically chalky white, whereas the native otic capsule bone is generally ivory in hue. The difference in color and its being confined to the lumen of the cochlea aids in differentiation of the neo-ossified bone and the native otic capsule while drilling the ossified cochlea during the implantation procedure.
Advanced otosclerosis may in rare cases cause luminal obstruction that is usually limited to the round window or first few millimeters of the scala tympani. It has been suggested by Kotzias and Linthicum that the otosclerotic process may damage the endosteal layer, resulting in the osteoplastic form of neo-ossification. Green and colleagues histologically identified foci of otosclerosis within the areas of neo-ossification. Moreover, all their specimens demonstrated the pathology to be limited to the first 6 mm of the basal turn in the scala tympani. The pattern of ossification induced by trauma is less predictable.
Evaluation of cochlear patency by CT scanning
Multiple investigators have reported discrepancies between the CT interpretation of cochlear patency and the findings at implant surgery, likely due in part to the thicker image slices available at the time these studies were performed, as well as the early stage of experience of the image interpreters. Early fibro-ossific changes are frequently encountered during surgery in postmeningitic patients and are frequently not identified on CT scanning, which would be especially likely when there is little ossification within the fibrous matrix. The time course for metaplastic ossification is quite variable but is thought to begin with fibrosis as early as 8 days to a few weeks after the initial insult. The ultimate time frame and extent of eventual osseous deposition is variable. It has been reported to be detected as early as 2 months postmeningitis in humans by CT scanning. Evidence of ongoing ossification has also been detected to be present histologically in human temporal bones as late as 30 years after the initial insult.
The reported accuracy of high-resolution CT identification of cochlear ossification has ranged from 53% to greater than 90%. A review of these studies is useful because they detail the pattern and likelihood of ossification found among various etiological factors of deafness as well as the potential pitfalls of CT-scan interpretation with regard to particular regions of the cochlea.
Literature reviews for CT assessment of cochlear patency
Jackler
In 1987 Jackler and colleagues compared CT interpretations with intraoperative findings on 35 cochlear implant patients (17 adults and 18 children) with a variety of deafness causes. The group included 1 adult and 7 children deafened by meningitis, making up 23% of the study population. CT scans were performed on a GE 8800 system with 1.5-mm contiguous axial sections processed using a bone algorithm. Axial scans were taken parallel to the infraorbital-meatal line, and coronal scans were tilted 105° from this plane. The CT data were reported as either patent or ossified (partial or complete), and detailed the location (round window, basal turn, middle turn, apical turn).
All patients deafened by meningitis had some degree of ossification found at the time of surgery; however, only 5 of 8 had a preoperative CT interpretation suggesting ossification, with the remaining 3 interpreted as normal. This study yielded a 37% false-negative rate among patients deafened by meningitis. However, looking at the specific case data presented, it is apparent that among the 3 instances of postmeningitic false-negative CT interpretation, 2 had partial ossification limited to the round window and the third had soft tissue in the round window region, and that all cases of basal-turn involvement were correctly identified by CT.
When all causes were considered, there were an additional 3 false-negative CT interpretations making a total of 6 out of 13, or a 46% false-negative rate. This total included one patient with Cogan syndrome who had ossification found in the round window and basal turn and one patient with trauma and basal-turn ossification, as well as one patient with prior malignant otitis externa, who had undetected complete cochlear soft tissue obliteration. All the children with congenital deafness (10) or ototoxicity (1) and all of the adults with progressive familial, viral, syphilis, Ménière, and unknown origins had patent readings on CT and no ossification noted at the time of surgery. The effects that these findings had on insertion and outcome are difficult to ascertain, as the 18 children in the study were implanted with a House 3M single-channel device and no performance data were provided; this is especially true in light of the fact that the great majority of patients today are implanted with long multichannel arrays.
Seicshnaydre
In 1992 Seicshnaydre and colleagues compared preoperative CT interpretation with intraoperative findings on 31 children who received a Nucleus multichannel cochlear implant, with scanning done using a high-resolution bone algorithm with slice thicknesses of 1 to 1.5 mm in the axial and coronal plane. These investigators analyzed their data differently from the previous study, considering 4 categories with regard to ossification: normal, narrowed basal turn, bony lip at round window, and ossified cochlea. Their cases were also subdivided into postmeningitic and nonmeningitic causes. A look at their specific data reveals the difficulty in interpreting the more subtle findings of narrowed basal turn and abnormalities of the round window. Among patients whose CT scans were interpreted as positive for narrowing or the basal turn, 71% were true positives and 29% were false-positive interpretations after confirmation at surgery. Of note is that all the false-positive cases were in nonmeningitic cases. When looking at abnormalities of the round window, there was an 80% false-positive rate of interpretation in nonmeningitic cases whereas all the meningitic cases were correctly interpreted.
Seidman
In 1994 Seidman and colleagues performed a retrospective comparison of preoperative CT radiologic reports and findings during cochlear implant surgery. CT scans were performed on a GE 9800 system with a slice thickness of 1.5 mm nonoverlapping, and used a high-resolution bone algorithm. Their analysis included 32 patients deafened by meningitis. Whereas 22 (69%) patients were found to have intraoperative evidence of ossification, only 7 were properly identified preoperatively. Ten patients were correctly identified as patent while 15 were falsely identified as patent, yielding a 60% (15/25) false-negative rate for preoperative CT interpretation of cochlear ossification among postmeningitic patients in this study. Of interest, these investigators also reported on one false-positive CT interpretation in a patient with osteogenesis imperfecta with a high-resolution CT scan, suggesting cochlear otospongiotic changes and luminal occlusion.
Langman and Quigley
In 1996 Langman and Quigley reported a sensitivity of 100% and specificity of 86% for the identification of cochlear obstruction using CT scans taken at 1.5-mm contiguous slices on a GE 9800 scanner; however, as they pointed out, only 14% of the patients in their study were deafened by meningitis. Langman and Quigley did not report data specific to etiology.
Summary of CT findings
In summary, it has been the author’s observation as well as those reported in the literature that advances in CT technology and radiologist experience have improved the ability to predict luminal obstruction on the basis of CT scanning, as current technology allows for the routine acquisition of 1-mm slice thicknesses. A subset of these patients, particularly those deafened by meningitis, may benefit from MR imaging to help distinguish the early fibrous phases of labyrinthitis ossificans from a patent fluid-filled lumen, as both may appear gray on CT scans. The evaluation of cochlear patency is initially performed by high-resolution CT scanning. Ossified obstruction can reliably be identified, involving the basal segment in isolation or extending distally into the middle and apical turns. Involvement limited to the basal segment on CT may be further evaluated by MR imaging so that a patent fluid-filled distal lumen may be differentiated from soft-tissue obliteration, as this may influence surgical planning ( Figs. 8–11 ).
Are There Additional Findings that May Complicate the Surgery or Subsequent Patient Management?
The initial objectives of preoperative sectional imaging are the determination of cochlear morphology and luminal patency. Additional useful information may be derived that can optimize safety and facility of surgery, as well as influence subsequent patient management. Proper surgical planning must involve careful review of sectional images so that potential complications may be anticipated and properly managed. Preoperative imaging often provides valuable information that would not preclude implantation, but rather helps assess which would be the technically easier ear to implant.
Vascular anatomy of the ear
Aberrant middle ear vascular anatomy that might complicate mastoidectomy and a facial recess approach to the cochleostomy may be anticipated by the routine acquisition of preoperative CT scanning. An extreme anterior displacement of the sigmoid sinus with approximation against posterior canal wall has been reported in 1.6%, and a high-riding jugular bulb may be present in 6% of the general population. It is rare, though possible, that a jugular bulb or diverticulum may overlie the round window niche or promontory ( Fig. 12 ). The distance between the round window and carotid artery may be determined in cases where a drill-out procedure is planned. Abnormal course or dehiscence of the carotid canal may also be detected.
Facial nerve
Preoperative CT scanning is especially useful in identifying the position of the aberrant facial nerve that may be associated with cochlear malformations. It has been well documented in such cases that the course of the facial nerve may be unusual and at increased risk of injury during implantation surgery. By careful preoperative mapping of the course of the facial nerve canal, such patients may be safely and successfully implanted ( Fig. 13 ). Careful review of the position of the facial nerve is also warranted in patients without cochlear malformations, as there may be dehiscence of the intratympanic portion that may be encountered during approach to the cochleostomy site.
In some patients with otosclerosis, the presence of spongiotic bone between the apical turn of the cochlea and the pregeniculate facial nerve canal permits unwanted stimulation of the facial nerve during implant use. Careful analysis of the CT study helps to anticipate that certain electrodes will require deprogramming ( Fig. 14 ).
Mastoid and tympanic cavity
The mastoid air cell system and tympanic cavity should also be included in the analysis of preoperative CT studies. The degree of mastoid pneumatization is especially useful information when operating on very young children. Though considered fully developed at birth, the depth of the facial recess as well as its degree of pneumatization may be anticipated.
Radiographic findings in conjunction with clinical severity may be considered in side selection as well as determination of the most appropriate course of therapy for patients with associated chronic ear disease. Chronic ear disease need not be an absolute contraindication for cochlear implantation if carefully selected patients are managed with staged procedures. Traditional canal wall surgery or a more extensive exenteration with a blind-sac and oversew type of operation is possible in more severe cases. Subsequent implantation may be performed in a stable, well-protected, and well-healed fat-obliterated mastoid cavity.
The usual landmarks for performing mastoidectomy and facial recess may be distorted or absent in patients with cochlear abnormalities. Careful review of CT images is essential in safely performing surgery on these patients. There are often associated morphologic abnormalities of the vestibular system and ossicular chain. Overall, the lateral semicircular canal is considered the most frequently malformed inner ear structure, which is speculated to be a result of its late embryonic formation.
The cochlear aqueduct
There is a consensus that the cochlear aqueduct plays a role in the pathologic process of labyrinthine ossification. Its role in the pathogenesis of spontaneous and intraoperative CSF fistula is less clear. Gushers during stapedectomy have been traditionally hypothesized to be associated with enlargements of the cochlear aqueduct. Jackler and Hwang doubted that the cochlear aqueduct plays a significant role in the etiology of CSF fistula. Radiographic reports of cochlear aqueduct enlargement are rare. Jackler and Hwang recommended that radiographic enlargement should be reported as a diameter exceeding 2 mm in its narrowest mid otic capsule portion. These investigators pointed out that the lateral otic capsule portion of the cochlear aqueduct is consistently narrow despite variability in the medial opening into the subarachnoid space, and is filled with a complex mesh of loose connective tissue.
Traditional arguments implicating the cochlear aqueduct as the source of CSF fistula assert that despite the presence of the tissue mesh, there is a contiguous lumen. Jackler and Hwang, however, suggest that there is no correlation between the clinical scenario of perilymphatic gusher and demonstrable radiographic enlargements of the cochlear aqueduct, even casting doubt on the existence in the literature of radiographically demonstrable enlargements of the lateral otic capsule portion of the cochlear aqueduct. Others have argued that even slight increases in the diameter may cause increases in flows, which may be at measurements smaller than can be detected on CT. It has been rebutted, however, that this argument does not take into account the baffling effect of the membranous mesh.
It is plausible, nonetheless, that the cochlear aqueduct may account for the “oozer” but not the “gusher” seen on entering the vestibule during stapes surgery. It is also possible that a similar pathogenesis may exist when easily controllable pulsatile perilymph is encountered during implant surgery at the time of cochleostomy. Its potential management should be anticipated if the presence of an abnormally enlarged cochlear aqueduct is seen on a preoperative CT scan of the implant candidate.
There is at least on case report in the literature, from 1982, of a 15-year-old boy with normal hearing in whom a CSF fistula between the posterior fossa and middle ear via and enlarged cochlear aqueduct was demonstrated by both metrizamide and Pantopaque contrast cisternography performed using conventional polytomography and CT scanning. Middle ear exploration reportedly revealed CSF leaking through a defect in the round window, which was patched. Two years later, during neurosurgical exploration via craniotomy for the treatment of recurrent CSF rhinorrhea and pneumococcal meningitis, it was reported that a tube of arachnoid was passing through a bony defect at the level of the cochlear aqueduct. This tube was believed to be successfully obliterated with muscle, with subsequent resolution of the CSF rhinorrhea and meningitis. The patient was reported as having otherwise normal inner ears and preservation of hearing.
A more likely encounterable and well-documented etiology for CSF leakage is through defective partitioning between the internal auditory canal fundus and the malformed inner ear. This situation was also documented in the aforementioned article by Park and colleagues in 2 other patients managed for recurrent meningitis and CSF fistula with demonstrable cochlear malformations. Both were children with unilateral deafness and inner ear malformations. One had a unilateral incomplete partition or classic Mondini deformity, and the other had bilateral dilated enlargements of the cochlea and vestibule.
The vestibular aqueduct
The association of enlargement of the vestibular aqueduct and congenital sensorineural hearing loss is well recognized. Radiographically, it may occur in conjunction with other identifiable inner ear anomalies as previously discussed, or as an isolated finding on CT or MR imaging (see Fig. 3 ; Fig. 15 ). Radiographic enlargement has been reported using different imaging modalities and criteria, but is generally considered to exist when the aqueduct’s diameter is greater than 1.5 to 2.0 mm at its midpoint, measured between the common crus and the external aperture into the posterior fossa.
