(1)
Division of Endocrinology and Metabolism, McMaster University, 1280 Main St W, Hamilton, ON, Canada, L8S 4L8
(2)
Clinical Medicine, Divisions Endocrinology and Metabolism and Geriatrics, McMaster University, 1280 Main St W, Hamilton, ON, Canada, L8S 4L8
Keywords
HypercalcemiaHyperparathyroidismCalciumHormoneParathyroidectomyHypoparathyroidismVitamin DMalignancyIntroduction
Hypercalcemia is a common medical problem in clinical practice. Many patients are asymptomatic at presentation [1]. Mechanisms of hypercalcemia include increased bone resorption, increased gastrointestinal absorption of calcium and/or increased renal calcium reabsorption. Hypercalcemia can be classified into parathyroid hormone (PTH)-dependent and PTH-independent causes [2]. Primary hyperparathyroidism (PHPT) and malignancy-associated hypercalcemia (MAHC) represent the most common etiologies of hypercalcemia and comprise approximately 80–90 % of the causes of hypercalcemia [3]. In this chapter we will discuss the etiology, clinical manifestations, diagnostic approach, and management of hypercalcemia (Table 9.1).
Table 9.1
Causes of hypercalcemia a
PTH-dependent hypercalcemia |
Primary hyperparathyroidism |
Familial hypocalciuric hypercalcemia |
Tertiary hyperparathyroidism |
Ectopic PTH production by a tumor |
PTH-independent hypercalcemia |
Increased bone resorption |
Humoral hypercalcemia of malignancy (HHM) |
Local osteolytic metastasis |
Hyperthyroidism |
Vitamin A intoxication |
Immobilization |
Increased calcium absorption |
Malignancy-induced 1,25 dihydroxyvitamin D |
Granulomatous diseases |
Vitamin D intoxication |
Milk alkali syndrome |
Parenteral nutrition |
Drugs: thiazides, lithium, theophylline toxicity, PTH analogues |
Miscellaneous causes: Adrenal insufficiency, Pheochromocytoma, Rhabdomylolysis, Jansen’s Metaphyseal Chondrodysplasia, Congenital Lactose Deficiency, William’s Syndrome |
Etiology
PTH-Dependent Hypercalcemia
Primary Hyperparathyroidism (PHPT)
PHPT is the leading cause of parathyroid hormone (PTH)-dependent hypercalcemia. The reported incidence is approximately 66/100,000 women and 25/100,000 in men [1]. This condition is usually identified at an asymptomatic stage due to routine measurements of serum calcium. In countries without routine serum calcium measurements PHPT is often symptomatic at presentation [4].
PTH is a key regulator of serum calcium [5]. The synthesis and secretion of PTH from the parathyroid chief cells increases upon detection of low circulating calcium levels by the calcium sensing receptor (CaSR) in the parathyroid chief cells. PTH binds to PTH receptors (PTH1R), the G protein coupled receptors on the cellular surface of bone osteoblasts and osteocytes [6]. This leads to increases in the expression of RANKL (ligand for the receptor activator of NFkB) by the osteoblast which in turn binds to its receptor RANK on preosteoclasts and osteoclasts and increases the formation, function, and survival of osteoclasts enabling increased bone resorption and the mobilization of calcium from skeletal reserves [6]. PTH may also mobilize the release of calcium from the bone surface without increasing bone resorption; however, this possible mechanism requires further study [5].
In the kidney PTH binds to PTH1R receptors on proximal and distal tubule cells and increases renal calcium reabsorption within minutes. The cortical thick ascending limb (CTAL) of Henle’s loop and the distal convoluted tubule (DCT) are the major sites of action for PTH and 1,25 dihydroxyvitamin D (1,25(OH)2 vit. D) [5–7]. PTH increases the renal production of 1,25(OH)2 vit. D by stimulating the synthesis of 1-alpha hydroxylase, allowing for increased absorption of calcium in the gut. 1,25(OH)2 vitamin D also increases RANKL expression on osteoblasts and thus increases bone resorption and the release of calcium from the skeleton [7].
In PHPT abnormal parathyroid tissue continues to synthesize and secrete PTH inappropriately in the presence of hypercalcemia. The precise pathophysiology for the development of this condition in sporadic cases is not known. It appears that the calcium set point is higher than normal. This set point is the calcium level where half-maximal suppression of PTH occurs. In sporadic PHPT this set point appears to be altered by 15–30 % permitting ongoing PTH secretion in the presence of high serum calcium [5]. In cases of PHPT specific gene abnormalities lead to failure of tumor suppressor activity, over expression of PTH precursor proteins, alterations in the calcium sensor and failure of inhibitors of cell growth [7].
The majority of individuals with PHPT have a single parathyroid adenoma (80 %), while four gland hyperplasia is seen in only 10–15 % [7, 8]. The genetic conditions implicated are multiple endocrine neoplasia type 1 (MEN1), multiple endocrine neoplasia type 2 (MEN2), multiple endocrine neoplasia type 4 (MEN4), hyperparathyroidism-jaw tumor syndrome (HPT-JT), and isolated familial hyperparathyroidism [7, 8]. Parathyroid carcinoma is fortunately a rare cause of PHPT [7, 8].
PHPT may be familial or sporadic. Familial PHPT is inherited as an autosomal dominant trait and may be part of a syndrome. Sporadic PHPT may also be due to a germline mutation which is de novo and the patient may be the only known case in that specific family. It is also possible that family members with PHPT may not have been identified and may have had asymptomatic disease or may have died before the condition developed. Sporadic parathyroid adenomas may be caused by a single gene mutation in a progenitor cell, leading to unregulated proliferation of parathyroid tissue. Chromosome 11 breakage and inversion leads to overexpression of a PTH promoter regulatory protein, cyclin D1. This rearrangement has been reported in 5 % of parathyroid adenomas. The cyclin D1 protein is overexpressed in 18–41 % of all parathyroid adenomas. Another implicated chromosome is chromosome 1p32-pter [7, 8]. Parathyroid adenomas are usually composed of parathyroid chief cells; however, oxyphil cell adenomas have been reported [9]. These lesions are more commonly seen in women post menopause.
Familial PHPT
The most common types of familial hyperparathyroidism are multiple endocrine neoplasia type 1 (MEN1) and type 2 (MEN2). MEN1 is associated with tumors of the parathyroids, pancreatic islets, and the anterior pituitary and is due to a germline mutation in the MEN 1 gene. This gene encodes menin a tumor suppressor [8]. This protein inhibits tumor formation in pancreatic, pituitary, and parathyroid tissues. Hyperparathyroidism has nearly 100 % penetrance in affected patients, and usually presents in the third decade [10].
MEN2 is due to mutations in the RET (rearranged during transfection) proto-oncogene which encodes a tyrosine kinase receptor and leads to the development of parathyroid tumors, medullary thyroid cancer, and pheochromocytoma [10]. MEN 3 is also due to RET mutations and is associated with medullary thyroid cancer, pheochromocytoma, and a marfanoid habitus. It is also associated with mucosal neuromas, medullated corneal fibers, and dysfunction of the autonomic ganglia in the bowel leading to megacolon and diverticulosis. Parathyroid tumors are rare in MEN 3 [10].
Patients with MEN4 have tumors in the parathyroids, anterior pituitary as well as gonads, adrenals and kidneys [10] and have mutations in the CDNK1B gene which encodes the cyclin-dependent kinase inhibitor CDK1 p27 kip1 [11]. To date, patients reported with MEN4 have had parathyroid adenomas and primary hyperparathyroidism, whereas expression of other endocrine tumor types has been variable. Cyclin-dependant kinase inhibitors (CDKIs) are cell cycle regulators which may become inactivated in endocrine neoplasias [11].
HPT-JT is associated with PHPT, jaw tumors, renal cysts, and renal hamartomas. Pancreatic adenocarcinoma, uterine and testicular tumors may also occur. Like MEN1, hyperparathyroidism is strongly expressed, occurring in 90 % of affected patients. However in HPT-JT the parathyroid tumors are usually single adenomas or carcinoma whereas in MEN 1 multiglandular disease is present [10]. In HPT-JT syndrome the HRPT2 gene is inactivated. This gene encodes a tumor suppressor parafibromin. When parafibromin is inactivated permissive tumor growth occurs [8].
Parathyroid Carcinoma
Parathyroid carcinoma is an unusual cause of primary hyperparathyroidism. It may arise sporadically or may develop in abnormal parathyroid tissue such as parathyroid hyperplasia, adenoma, following neck irradiation, renal disease, or prolonged secondary hyperparathyroidism [12, 13]. These associations have not been consistently observed or reported and require further study. Familial parathyroid carcinoma does occur and is associated with HPT-JT syndrome in which there is an abnormal chromosome 1q31.2 and in isolated familial hyperparathyroidism [8, 13]. Like parathyroid adenomas, parathyroid carcinomas frequently demonstrate overexpression of cyclin D1. This overexpression of cyclin D1 has been reported in up to 91 % of these tumors [13]. Parathyroid carcinoma is rare and occurs in only 1–2 % of all PHPT [13].
The presentation of parathyroid carcinoma is similar to the presentation of PHPT; however, patients with carcinoma usually have severe hypercalcemia in comparison to individuals with benign parathyroid disease [12, 13]. In parathyroid carcinoma significant elevations in PTH and calcium levels are present with serum calcium usually being higher than 3.5 mmol/L. The mean PTH elevation in one review was 10 times above the upper limit of normal [13]. This distinguishes carcinoma from benign parathyroid disease. The mean age for the presentation of parathyroid carcinoma is in the fifth decade. Parathyroid carcinoma occurs equally in males and females, whereas benign parathyroid disease occurs more commonly in females. Individuals with parathyroid carcinoma may present with a neck mass. Despite these clinical clues, parathyroid carcinoma is often diagnosed on surgical pathology [12, 13].
Familial Hypocalciuric Hypercalcemia
Familial hypercalcemic hypocalciuria (FHH) is a rare autosomal dominant disorder due to an inactivating mutation of the CaSR on the parathyroid cells and in the kidney tubules [14–18].
In this disorder, the PTH level may be increased due to decreased parathyroid cell sensitivity to the elevated serum calcium concentration. Approximately 5–10 % of patients have a minimal elevation of PTH [18]. The renal tubular calcium reabsorption is increased in association with impaired function of the CaSR in the kidney in addition to increased PTH secretion [15]. The loss of CaSR function enhances renal tubular reabsorption of magnesium [18]. Patients usually present during childhood and have a positive family history of hypercalcemia. Almost all patients have hypercalcemia; however, the degree of hypercalcemia is mild and the majority of patients are asymptomatic or have minimal symptoms only [19]. Calculating the calcium/creatinine (Ca/Cr) clearance ratio enables differentiation of FHH from PHPT [20]. In approximately 80 % of cases of FHH the Ca/Cr clearance ratio is less than 0.01. In the remaining patient population a higher calcium to creatinine clearance ratio can be seen up to 0.02 making it difficult to differentiate FHH from PHPT particularly in the presence of vitamin D insufficiency or renal insufficiency [18]. DNA sequencing of the CaSR gene with identification of an inactivating mutation enables confirmation of the diagnosis [20]. Parathyroidectomy will not normalize the elevated serum calcium in FHH; however, in the rare circumstances of a homozygous inactivating mutation of the CaSR gene, a condition known as neonatal severe hyperparathyroidism, immediate parathyroidectomy is the treatment of choice [21].
Tertiary Hyperparathyroidism
Autonomous PTH secretion associated with hypercalcemia in patients with chronic kidney disease is known as tertiary hyperparathyroidism [22]. Although the mechanisms of tertiary hyperparathyroidism are not well understood, many theories suggest prolonged stimulation of the parathyroid glands results in anatomic hyperplasia with autonomous function of the parathyroid glands [23]. The expression of the CaSR and vitamin D receptors (VDRs) in tertiary hyperparathyroidism is decreased leading to further increases in PTH secretion. This may result from continuous parathyroid stimulation in secondary hyperparathyroidism, followed by further development of polyclonal autonomy [24]. Parathyroidectomy is the treatment of choice to eliminate the adverse effects of hypercalcemia and hyperparathyroidism [25].
Ectopic Parathyroid Hormone
Ectopic PTH secreting tumors are extremely rare. A few published cases in the last two decades have reported ectopic PTH secreting tumors. PTH secretion from small cell lung cancers, ovarian cancers, and papillary thyroid cancers have been described. Clinically this is characterized by increased parathyroid hormone and serum calcium concentration in the absence of parathyroid adenoma or hyperplasia on radiologic images [26–28].
PTH-Independent Hypercalcemia
Malignancy-Associated Hypercalcemia
Malignancy-associated hypercalcemia (MAHC) is the most common cause of hypercalcemia in hospitalized patients. It accounts for approximately 90 % of inpatient hypercalcemia [2]. Hypercalcemia is usually evident clinically when the diagnosis of malignancy is made and may predict poor prognosis; however, it is unlikely to be the initial presenting symptom of malignancy [29]. Men are at a higher risk for developing hypercalcemia in the setting of malignancy. Though in general most hypercalcemic patients are women [29, 30].
Four mechanisms are responsible for the increased serum calcium concentration seen in malignancy. These include increased parathyroid hormone-related peptide (PTHrP) production which is a mechanism for humoral hypercalcemia of malignancy (HHM) , increased production of osteolytic factors, increased production of 1,25 dihydroxyvitamin D (1,25(OH)2), and finally ectopic parathyroid hormone release by tumor cells [2]. Eradication of the tumors is the treatment of choice in MAHC [2].
Humoral Hypercalcemia of Malignancy
Excessive secretion of PTHrP is the most common mechanism of MAHC. It accounts for approximately 80 % of MAHC [2]. The majority of the patients have squamous cell carcinoma most commonly lung tumors. Other malignancies including bladder, renal, breast, and ovarian carcinoma may be associated with elevated PTHrP. It can also rarely be seen in hematological malignancies such as Non-Hodgkin lymphoma and leukemia [2].
PTHrP is a member of the PTH family, identified in 1987 in cancer patients with hypercalcemia [31]. PTHrP shares a similar sequence homology with PTH in the first 13 amino acids at the N terminus [32]. Secretion of PTHrP, activates osteoclast activity and suppresses osteoblast activity leading to the release of calcium from the skeleton [32]. PTHrP increases renal calcium reabsorption and decreases phosphate reabsorption in renal tubules resulting in hypercalcemia, hypocalciura, hypophosphatemia, and hyperphosphaturia [33]. In contrast to PTH, PTHrP does not increase the intestinal calcium reabsorption due to an inability to activate 1-ɑ hydroxylase and hence 1,25(OH)2 vitamin D production [33]. This difference in the action of PTHrP in comparison to PTH relates to differences in the parathyroid hormone 1receptors (PTH1R) in comparison to PTH. In addition, PTHrP does not bind to the PTH2R which is present in the gastrointestinal tract (GIT) [34, 35]. The elevations in PTHrP seen in gynecologic tumors and in pheochromocytoma normalize with surgical removal of these tumors [36–38].
Local Osteolytic Metastasis
Local osteolytic metastasis contributes to hypercalcemia and account for approximately 20 % of malignancy-associated hypercalcemia in one large series [2]. Some solid tumor cells produce local PTHrP, this occurs in metastatic breast cancer to the bone with upregulation of RANKL expression in bone [39, 40]. In contrast, myeloma cells produce cytokines including interleukin-6 (IL-6), IL-3, IL-1, and macrophage inflammatory protein 1α (MIP1). These osteoclastogenic molecules increase RANKL expression and decrease the production of osteoprotegerin (OPG) . The elevated RANKL/OPG ratio increases the osteoclast activity and bone resorption [41–43]. Other hematological malignancies may mimic multiple myeloma in increase calcium release from the skeleton [44].
Thyrotoxicosis
Hypercalcemia can be seen in hyperthyroidism. Thyroid hormone activates the RANKL/RANK system via increased expression of osteoclastic cytokines. This results in increased calcium concentration in the serum [2]. It is usually associated with increased levels of the circulating IL-6 [45]. Although calcium absorption decreases in the gut and calcium excretion increases through the kidney due to suppressed PTH, up to 50 % of hyperthyroid patients have elevated total or ionized calcium [46]. Most of these patients have mild elevation of calcium; severe hypercalcemia in hyperthyroid patients is rarely seen [2]. Correction of thyroid hormone level will restore the balance of osteoclast/osteoblast activity in the bone and normalize calcium and PTH levels in the blood [47].
Hypervitaminosis A
Vitamin A is a fat soluble vitamin which is stored in the liver. Hypercalcemia can be caused by vitamin A intoxication. The mechanism is not well understood but may be due to direct stimulation of osteoclast bone resorption or stimulation of other cytokine expression [48]. The causes of hypervitaminosis A are related to high vitamin A intake as a supplement especially in chronic kidney disease or as a treatment of certain tumors or dermatological diseases by retinoic acid derivatives [49].
Immobilization
Prolonged immobilization can increase osteoclast activity and suppress osteoblast activity causing calcium release from the bone into the circulation. Hypercalciuria occurs as a result of increased bone remodeling [50]. Diseases associated with prolonged immobilization include spinal cord injury, stroke or multiple fractures and may present with hypercalcemia. Immobilized patients with Paget’s disease may also present with hypercalcemia [2]. Both young adults and the elderly are at risk for increased calcium levels with prolonged immobilization [51]. In addition to bisphosphonate administration, early mobilization and adequate hydration will reduce the risk of hypercalcemia by suppressing bone resorption and increasing the urinary excretion of calcium [51, 52]. Denosumab can be used in hypercalcemic immobilized patients after partial or transient response to bisphosphonate [53].
Malignancy-Induced 1,25 Dihydroxyvitamin D
Overexpression of the enzyme 1-α hydroxylase by malignant cells or adjacent normal cells converts 25-hydroxyvitamin D into abnormally elevated 1,25(OH)2 vitamin D (calcitriol) level [2]. This leads to increased calcium absorption from the gut and results in hypercalcemia in the presence of suppressed PTH level, with normal phosphate level in the blood, absence of renal phosphate wasting and increased renal calcium excretion initially and decreased renal clearance over time as a result of dehydration [2]. Calcitriol also has a direct effect on RANKL/RANK system leading to increased osteoclast activity and bone resorption [2]. These pathophysiological abnormalities have been reported in all types of lymphoma, particularly Hodgkin lymphoma and more than 30 % of non-Hodgkin lymphoma [54]. This mechanism also has been described in patients with ovarian dysgerminomas [55] and chronic granulomatous diseases such as sarcoidosis.
Granulomatous Diseases
Similarly, granuloma cells continue to produce 1α hydroxylase which converts 25-hydroxyvitamin D to 1,25(OH)2 vitamin D. This occurs in spite of suppressed PTH, leading to increased intestinal calcium absorption, bone resorption, hypercalciuria, and hypercalcemia [2]. Elevated calcitriol and calcium concentration in anephric patients with sarcoidosis support the idea of granuloma cells as the synthetic source of 1,25-dihyroxyvitamin D in the systemic disease not only the kidney [56]. Nearly all known granulomatous diseases have been reported to cause hypercalcemia via this mechanism. In sarcoidosis, about 30 % of patients have hypercalciuria and 10 % have hypercalcemia during their life [57]. The risk of hypercalcemia is aggravated with prolonged exposure to sun light and eating a diet rich in vitamin D [57]. In tuberculosis, the prevalence of hypercalcemia varies from one country to another depending on dietary intake of calcium and vitamin D. If the calcium or vitamin D intake is inadequate the prevalence of hypercalcemia will be low, but if the intake is adequate or high the prevalence will be high [58].
The treatment of choice in granulomatous diseases is prednisone. Calcium level often starts to normalize after 2 days of starting steroid therapy [2, 57]. Treatment of the underlying cause with antituberculosis or antifungal agents or adequate hydration as well as a low vitamin D and calcium diet with avoidance of prolonged sunlight exposure will reduce and maintain calcium level in the normal reference range [2]. Antifungal treatment such as Ketoconazole can be used as a second line therapy if patients with sarcoidosis do not respond to steroid therapy [57], or have severe symptoms [59].
Hypervitaminosis D
Vitamin D is a fat soluble vitamin which is stored in the liver with excess vitamin D being stored in fat cells. High concentrations of vitamin D, inactive 25-hydroxyvitamin D, and the active metabolite 1,25(OH)2 D, can cause hypercalcemia by increasing intestinal calcium absorption and calcium efflux from the bone [2]. Hypervitaminosis D may be a result of excess intake of any vitamin D preparation orally or topically [60]. Cases have been reported of excessive vitamin D fortification of milk [61]. A further etiology is via the endogenous production of calcitriol associated with lymphomas and granulomatous diseases, discussed previously. Prolonged exposure to sunlight will not lead to vitamin D intoxication as excess photoconverted previtamin D3 and vitamin D3 will form two inactive metabolites, lumisterol3 and tachysterol3, which maintain vitamin D levels within a normal range [62].
Cessation of calcitriol and adequate hydration are the best treatment for hypercalcemia secondary to calcitriol due to its short half-life. Hypercalcemia induced by 25-hydroxyvitamin D (Calcidiol) requires more aggressive treatment with a bisphosphonate as it persists over a longer time period [63].
Milk Alkali Syndrome
In the past, milk alkali syndrome was described in patients with peptic ulcer disease who were treated with an excessive amount of milk and sodium bicarbonate. Now, it is more common in patients who are taking excessive amounts of calcium carbonate [64]. High calcium supplement intake results in increased calcium concentrations that induce diuresis by activation of CaSRs in the ascending limb of the Loop of Henle and interferes with antidiuretic hormone (ADH) action in collecting duct. This will cause volume contraction and increase bicarbonate absorption in the renal tubule leading to an elevated pH concentration in the blood [64]. Metabolic alkalosis may worsen the hydration status and aggravate the calcium level [64]. High renal tubule calcium concentrations activate the CaSRs in the distal convoluted tubules leading to increases calcium reabsorption via the transient receptor potential vanilloid member 5 (TRVP5 channels [65]. The glomerular filtration rate (e-GFR) will be reduced as a direct effect of hypercalcemia on renal arterioles and volume contraction in the acute stage, and nephrocalcinosis and nephrolithiasis in the chronic stage [64]. Thiazide diuretics , non-steroids anti-inflammatory drugs, angiotensin-converting enzyme inhibitors, or any drugs or medications that reduce glomerular filtration rate may increase the risk to develop this syndrome [66]. Milk Alkali syndrome is characterized by hypercalcemia, metabolic alkalosis, and renal impairment. Treatment includes cessation of the insulting drug and administrating adequate hydration [64].
Parenteral Nutrition
The exact mechanism of parenteral nutrition-induced hypercalcemia is not fully understood. Administration of total parenteral nutrition (TPN) has been associated with hypercalcemia, hypercalciuria, and osteomalacia [67]. Hypercalcemia may be caused by excess calcium in the TPN solutions or increased bone resorption by excess vitamin D [67, 68]. TPN containing contaminated casein hydrolysate with aluminum can cause low bone turnover resulting in unbalanced activity of markedly low osteoblasts and low or normal osteoclasts [68, 69].
Drugs
Thiazide
The direct action of thiazide on calcium reabsorption occurs in the distal convoluted tubule. Thiazides can also increase calcium reabsorption in the proximal tubule indirectly thorough the effect of volume depletion as a result of increased sodium and water excretion [70]. In general thiazides can cause mild hypercalcemia and hypocalciuria; however, severe hypercalcemia has been reported only in the setting of underlying primary hyperparathyroidism [71].
Lithium
Hypercalcemia may develop in patients on lithium therapy as a result of increased PTH secretion; this may occur more frequently in the elderly [72]. Lithium may unmask the presence of PHPT or lead to parathyroid adenoma formation or hyperplasia. Lithium has been postulated to inactivate the CaSR on parathyroid glands [2, 73]. Lithium also stimulates calcium reabsorption in the renal tubule and inhibits renal cyclic AMP formation directly causing mild hypercalcemia, hypocalciuria, and low renal cyclic AMP [2, 74]. If a hypercalcemic patient cannot tolerate lithium withdrawal, close monitoring with active surveillance is a valuable treatment strategy. Cinacalcet, a calcimimetic agent, has been reported to normalize calcium levels in lithium-induced hypercalcemia [75]. Neck exploration and parathyroidectomy can be considered in symptomatic and severe hypercalcemia [76].
Theophylline Toxicity
Several reports have described hypercalcemia in the setting of theophylline toxicity. This occurs in a PTH-independent fashion. Normalization of calcium levels after propanolol administration to these patients suggests that an adrenergic effect may be responsible [77].
Parathyroid Hormone Analogues
Parathyroid hormone analogues are anabolic agents used for the treatment of osteoporosis. Two molecules are available: PTH (1-34) and PTH (184). Each is associated with mild and/or transient hypercalcemia which is rarely severe or persistent. Usually no intervention is required; however, dose reduction of PTH or even cessation of therapy may be required in severe cases [78].
Miscellaneous Causes
Other causes of hypercalcemia include adrenal insufficiency, pheochromocytoma, rhabdomyolysis, and rare genetic conditions such as Jansen’s metaphyseal chondroplasia, congenital lactose deficiency, and William’s syndrome.
Adrenal Insufficiency
Adrenal insufficiency is rarely complicated by hypercalcemia. This is described in the setting of acute adrenal crisis and responds to steroid replacement therapy. The etiology of hypercalcemia in this setting may be related to hemoconcentration or increased renal absorption of calcium at the level of the proximal tubules [79].
Pheochromocytoma
Pheochromocytoma is very rarely the cause of hypercalcemia. However, in patients with pheochromocytoma hypercalcemia may be the result of MEN2A (PHPT rather than pheochromocytoma is the true cause of hypercalcemia in this setting), or alternately the pheochromocytoma itself may produce PTHrP. VIPoma are also thought to produce PTHrP [80].
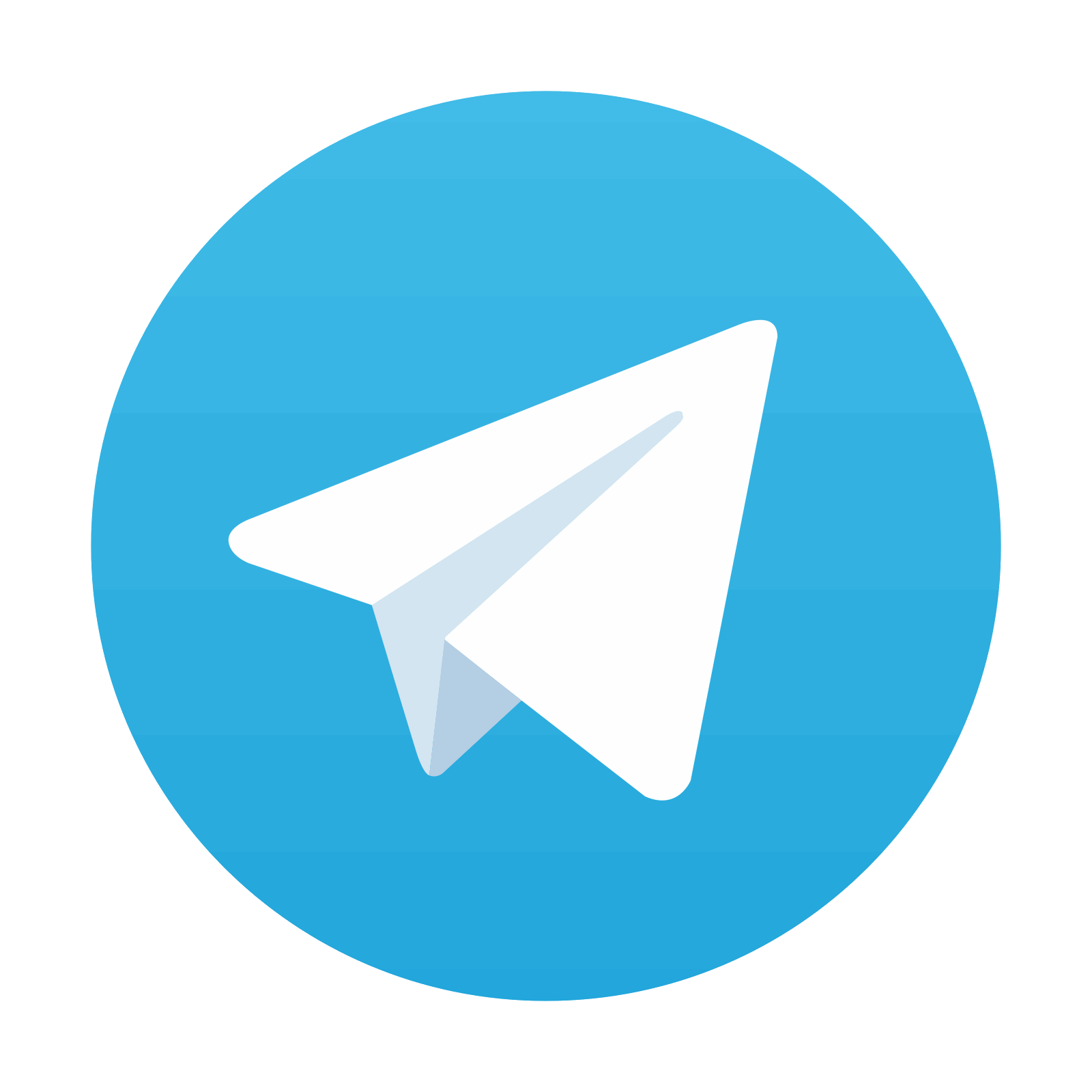
Stay updated, free articles. Join our Telegram channel

Full access? Get Clinical Tree
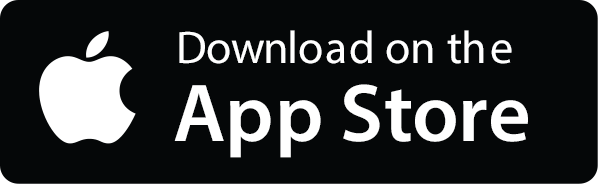
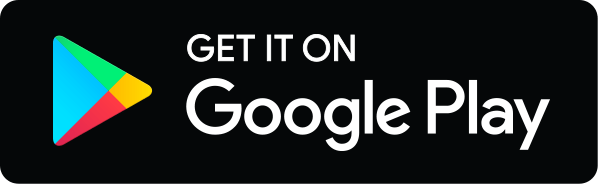
