Fig. 14.1
Alterations within the area of geographic atrophy. (a) SD-OCT showing the absence of the outer retinal layers and remaining of a thin line on the outer margin of the original site of band 4, most likely representing Bruch’s membrane. (b–d) SD-OCT showing plaques (b, arrows), elevations (b, asterisks), and clumps (d, arrows) based on the remaining of band 4. (e) SD-OCT showing a dotted line, seemingly continuing the elevated part of band 4 that splits at the atrophy margin that can be especially found in the “diffuse-trickling” FAF phenotype (Fleckenstein et al. 2011). (f–h) SD-OCT showing irregular elevations (f), crown-like elevations filled with hyperreflective “debris” (g), and irregular hyperreflective material (h) also based on band 4 that can be distinguished from the previous findings (Image obtained from Fleckenstein et al. 2008)

Fig. 14.2
Sequential loss of outer retinal bands. (I) Atrophy border in fundus autofluorescence image for comparison. (II–VI) SD-OCT showing loss of retinal bands ordered from the inner to outer retina. First, the ellipsoid zone (termed inner segment/outer segment photoreceptor layer [IPRL] here) is disrupted (IV). This is followed by choroidal signal enhancement (VI), visible RPE attenuations (V), external limiting membrane (ELM) loss (III), and the entire outer nuclear layer (ONL) loss (II) (Image obtained from Schmitz-Valckenberg et al. (2011), with permission)

Fig. 14.3
Visualization of the progression of geographic atrophy. Serial SD-OCT imaging shows atrophy enlargement over time as progressive loss of the inner part of the RPE-BM complex, the ellipsoid zone, and the ELM, respectively, and thinning of hyporeflective band representing the ONL at the border of atrophy. Moving into the atrophic lesion, the ONL progressively thins, and the hyperreflective band above (OPL) approaches the remaining part of band 4 (assumed Bruch’s membrane) (Image with permission from Fleckenstein et al. 2010)

Fig. 14.4
Different types of borders as visualized by SD-OCT. (a, b) The assumed RPE-BM complex (band 4) narrows; and an outer layer remains throughout the atrophic area (assumed Bruch’s membrane). According to Brar et al. (2009), the margin depicted in (a) represents a type 1 border with smooth margins and no alterations of the outer retina, and the margin in (b) represents a type 2 border with severe alterations in the outer retinal layers and irregular margins. (c) In contrast to (a) and (b), the “splitting” border is characterized by splitting of the RPE-BM complex in inner and outer regions (Image obtained from Fleckenstein et al. 2011)
Plaques at the level of the former RPE-BM complex correlating with highly reflective lesions in the IR image (Fig. 14.1b) (Fleckenstein et al. 2008).
Clumps that rest on the thin remainder of RPE-BM, scattered throughout the atrophy. They may be noted also in other retinal layers (Fig. 14.1d) (Fleckenstein et al. 2008).
Irregular elevations in place of the inner portion of the RPE-BM band (Fig. 14.1c) (Fleckenstein et al. 2008; Ooto et al. 2014).
Elevations with crown-like appearance with presumed debris beneath these structures, also termed hyperreflective pyramidal structures (Fig. 14.1g) (Bonnet et al. 2014; Fleckenstein et al. 2008).
Larger accumulations of irregularly highly reflecting material (Fig. 14.1h) (Fleckenstein et al. 2008).
Branching, round, or ovoid hyporeflective structures with a hyperreflective border located in the outer nuclear layer (termed outer retinal tubulations – ORT) (Zweifel et al. 2009).
A thin, discontinuous hyperreflective band between the inner nuclear layer (INL) and the remaining outer part of RPE-BM band may be visible. This dotted band is most pronounced close to the atrophy border zone (Fig. 14.1e) (Fleckenstein et al. 2008, 2011).
The nature and the prognostic relevance of most of these structures are yet unknown. Elevations or accumulations of highly reflective material as well as plaques at the former RPE level might represent residual sub-RPE deposits and reflect various stages of regressing drusen, which may develop focal calcification and become increasingly irregular (Fleckenstein et al. 2008; Green and Key 1977; Sarks et al. 1988). The thin, discontinuous hyperreflective band between the INL and the remaining outer part of RPE-BM band is predominantly present in the “diffuse-trickling” GA phenotype, which is associated with rapid atrophy progression (Fleckenstein et al. 2011; Moussa et al. 2013). ORT are hypothesized to represent degenerating photoreceptors that rearrange in a tubular fashion. With respect to the prediction of atrophy progression rates, recent works have produced competing results showing that ORT predict significantly faster (Moussa et al. 2013) or slower progression rates (Hariri et al. 2014).
14.3 Atrophy Border Zone
Atrophic areas typically enlarge over time. During this process, not all outer retinal layers are lost at the same time. Loss of the ellipsoid zone may spatially precede the loss of the remainder OCT bands (Fleckenstein et al. 2008; Schmitz-Valckenberg et al. 2011). It is followed by attenuations in the RPE, first becoming more transparent to light reflecting from the choroid (“choroidal signal enhancement”) and subsequently showing visible RPE alterations (Schmitz-Valckenberg et al. 2011). Complete ONL loss can usually be observed within the area of RPE loss (94 % of the cases) (Bearelly et al. 2009; Schmitz-Valckenberg et al. 2011). However, ONL loss does not occur abrupt. It rather thins gradually initiating on average 61 μm outside of the atrophy borders. Loss of ELM starts slightly inside the border of RPE atrophy (Fig. 14.2) (Bearelly et al. 2009). The inner retinal layers usually remain intact across the atrophic border (Wolf-Schnurrbusch et al. 2008). The morphologic alterations as described here are associated with remarkable functional impairments as shown by microperimetric examinations (Sayegh et al. 2014). In a longitudinal study, it could be confirmed that expansion of GA patches was accompanied by advancing the loss of ELM, ellipsoid zone, the inner part of the RPE-BM complex, and a thinning of the ONL (Fig. 14.3) (Fleckenstein et al. 2010). In accordance with a progressive thinning of these layers, a decrease of retinal thickness over time (average – 14 μm/y) could be observed (Fleckenstein et al. 2010).
The exact shape of outer retinal layer loss at the atrophy border underlies interindividual differences. Three main types of atrophy border can be discriminated (Fig. 14.4). A first classification introduced by Brar et al. classified two distinct border types (Brar et al. 2009). Type 1 shows smooth margins and no alterations of the outer retina (Fig. 14.4a), while type 2 exhibits severe alterations in the outer retinal layers and irregular margins with or without increased optical reflectivity of the RPE (Fig. 14.4b). Subsequently, an additional border type termed “splitting” was described that is characterized by a splitting of the RPE-BM complex (Fig. 14.4c) (Fleckenstein et al. 2011). Of note, these atrophy border types are not patient or eye specific. Rather surrounding a single atrophic lesion, several of these types may occur. Little is known about the impact of border morphology on atrophy progression. A recent longitudinal study suggests that disruption of the ellipsoid zone predicts the direction of atrophy growth (Nunes et al. 2013). This observation suggests that photoreceptor damage occurs prior to RPE loss (Schmitz-Valckenberg et al. 2011). The “splitting” type atrophy border was shown to be predominant in the rapidly progressing “diffuse-trickling” type of GA (Fleckenstein et al. 2011). Consequently the “splitting” border type itself is also associated with rapid atrophy progression (Moussa et al. 2013). Using minimum intensity en face SD-OCT projections, a recent study found a tendency of GA progression to preferentially occur in areas of increased minimum intensity. The minimum intensity of an OCT A-scan is found in the ONL; these results thus suggest that alterations in this zone precede atrophy development (Stetson et al. 2014). To date it has not been investigated if these changes in minimum intensity are exactly correlated with the decrease in ONL thickness that has been observed to precede RPE loss (Bearelly et al. 2009).
14.4 Perilesional Zone
Heterogeneous changes can be noted in the perilesional zone of GA, which encompass retinal areas with visible abnormalities on the fundus photograph, SLO image, or SD-OCT outside the atrophic patches. In some sections, the retinal layers appear without noticeable alterations either of the inner or the outer retinal layers. Alterations of ellipsoid zone and RPE-BM complex may impede observation of the interdigitation zone (Fig. 14.5b–k), and changes can be so intense that the four outer hyperreflective OCT bands become indistinguishable (Fig. 14.5g). Thinning and thickening of the RPE-BM complex are common (Fig. 14.5b) and highly reflecting, segmented plaques may be observed in this band (Fig. 14.5c). Elongated elevations (not correlated by funduscopically visible drusen) and dome-shaped elevations that correlate with drusen in fundus images (Fig. 14.5d–f) may occur (Fleckenstein et al. 2008). Beneath drusen, a thin line usually remains present on the former level of the RPE-BM complex (Fig. 14.5c–f). These dome-shaped elevations may precede atrophy development (Fleckenstein et al. 2010). A recent study identified pathological changes occurring before the development of drusen-associated atrophy using SD-OCT, which was defined as “nascent” GA (Wu et al. 2014). Unique features in these areas included subsidence of the OPL and INL and development of a hyporeflective wedge-shaped band within the limits of the OPL (Wu et al. 2014). There are several changes that can be observed on top of such a dome-shaped elevation, but may also occur independently (Fleckenstein et al. 2008). Apical extensions of the thickened RPE-BM complex can be observed with and without associated disruptions in the other outer retinal bands. These apical extensions may have clumps above the tips in different retinal layers (Fig. 14.5g–i). Such vertical extensions had spikelike appearances, beginning at the level of the RPE-BM complex, with extension through the overlying bands (Fig. 14.5h) (Fleckenstein et al. 2008). Longitudinal observations show that these findings may be considered as a continuum with clumps on the RPE-BM complex initially not disrupting the ellipsoid zone or ELM migrating toward the inner retinal layers and disrupting the retinal bands between. The clumps may either fade with time or precede atrophy development (Fleckenstein et al. 2010). Hyperreflective clumps may be found also independently of underlying spikelike lesions and can be noted at the level of the OPL. Underneath those clumps, outer retinal layers usually appear disrupted as if a piece of the RPE-BM complex had been torn out and broken through the layers above to finally localize at the assumed OPL level (Fig. 14.5j) (Fleckenstein et al. 2008). In the perilesional zone, a mottled irregular appearance of the RPE-BM complex accompanied by small elevations of the ellipsoid zone, commonly associated with a reticular drusen pattern in the FAF and IR images (Fig. 14.5k), may be present. Occasionally an increased distance between ellipsoid zone and RPE-BM complex can be found (Fig. 14.5l). In one eye of a patient, typically multiple such alterations can be detected.


Fig. 14.5
Alterations of the perilesional zone. (a) SD-OCT scan obtained from a clinically normal appearing retina. (b) SD-OCT showing thickening of the RPE-BM complex. (c) SD-OCT showing elongation and elevation of the RPE-BM complex with plaques beneath. (d) SD-OCT showing dome-shaped elevations with preserved layers above. (e) SD-OCT showing dome-shaped elevation with clumps at the top. (f) SD-OCT showing dome-shaped elevation with backscattering material beneath. (g) SD-OCT showing apical extensions of the RPE-BM complex. (h) SD-OCT showing a spike with a clump at the tip. (i) SD-OCT showing clumps at different retinal levels. (j) SD-OCT showing a clump in the OPL with disrupted bands beneath. (k) SD-OCT showing small elevations of the ellipsoid zone and mottled RPE-BM complex. (l) SD-OCT showing increased distance between both bands (Figure copyright: Fleckenstein et al. 2008)
14.5 Morphology of the Spared Fovea
Typically, atrophic patches initially occur in the parafoveal retina. Over time, several atrophic patches may coalesce, and new atrophic areas may occur. This can result in a horseshoe- and later ringlike configuration of atrophy surrounding the fovea. Thus, the fovea itself may remain uninvolved in the atrophic process until late in the course of the disease, a phenomenon referred to as “foveal sparing” (Sunness et al. 1995; Sarks et al. 1988). When the fovea finally becomes involved, a dramatic loss in visual acuity occurs, and less benefit would be expected from any future therapeutic intervention aiming to slow down or halt the natural course of the disease (Maguire and Vine 1986; Sunness et al. 1999). In SD-OCT scans of a healthy macula, the foveal depression (resulting in the physiological absence of the inner retinal layers in this area) is visualized (Fig. 14.6a). In patients with AMD and GA, the “spared fovea” may exhibit various alterations. Frequently an irregular and broad band of increased reflectivity at the site of the three outer hyperreflective bands can be found making details of these three bands invisible (Schmitz-Valckenberg et al. 2009). However the ELM usually remains visible. Interestingly when the atrophy does not entirely surround the fovea, outer retinal bands may be distinguishable (Wolf-Schnurrbusch et al. 2008). In the spared fovea, the ONL was found to be markedly swollen (Fig. 14.6b) (Schmitz-Valckenberg et al. 2009). Recent results suggest that not the entire ONL but rather the Henle’s fiber layer was thickened. This implies that not a swelling of the photoreceptor nuclei themselves but rather their axons or of the Müller cells is responsible of the observed thickening (Mones et al. 2013). Surrounding atrophy of the outer retinal layers together with this foveal swelling leads to a loss of the usual concave configuration of the foveal pit. Instead the inner retinal layers show a convex shape. The inner retinal layers appear to be distorted and to have a tendency toward perifoveal atrophy. At the site of atrophy, marked retinal thinning and the absence of the characteristic morphology of outer retinal layers are present (Schmitz-Valckenberg et al. 2009). At the edge between atrophy and spared fovea, so-called “wedge” hyperreflective structures ranging from the inner portion of the RPE-BM complex to the inner limit of the outer plexiform layer can be found in 70 % of the cases (Mones et al. 2012).


Fig.14.6
Comparison of the foveal structure as observed in SD-OCT in a clinically healthy retina of a right eye (upper panel) and a left eye with GA and foveal sparing (lower panel). Underneath the SD-OCT images, retinal thickness curves are shown. In GA with foveal sparing, the subfoveal retinal thickness is increased as compared to the surrounding atrophic areas. The inner retinal layers appeared distorted and moved into the perifoveal atrophy (Image obtained from Schmitz-Valckenberg et al. (2009), with permission)
14.6 Evaluation of the Choroid in Geographic Atrophy
Choriocapillary atrophy is a well-described feature of GA in AMD (Green and Key 1977; Sarks et al. 1988), and a more general involvement of the choroid in the process of atrophy development has been hypothesized (Friedman 1997, 2004, 2008). Several recent studies made advantage of the newly developed enhanced depth imaging (EDI) modality in SD-OCT devices that enables the evaluation of structures beyond the Bruch’s membrane, in particular the entire choroid and the choroido-scleral interface (Fig. 14.6a). Thereby reproducible measurement of choroidal thickness (CT) becomes possible (Margolis and Spaide 2009; Spaide et al. 2008). Works on early stages of AMD have revealed controversial results finding the CT decreased or unchanged in these patients (Adhi et al. 2014; Chung et al. 2011; Coscas et al. 2014; Jonas et al. 2014; Jirarattanasopa et al. 2012; Kang et al. 2014; Kim et al. 2011; Koizumi et al. 2011; Lee et al. 2013; Manjunath et al. 2011; Noori et al. 2012; Sigler and Randolph 2013; Wood et al. 2011). In contrast, three recent studies have assessed CT in GA in particular and consistently find that the choroid is thinned in eyes with this late-stage manifestation of AMD (Adhi et al. 2014; Lee et al. 2013; Lindner et al. 2015) (Fig. 14.6b). The extent of thinning exceeds the dimension that could be attributable to choriocapillary thinning alone; thus these findings suggest a more general role of the choroid in GA. Yet the extent of choroidal thinning seems to be different in distinct subtypes of GA (Lindner et al. 2015). Longitudinal data assessing whether GA development precedes choroidal thinning or vice versa are still missing.
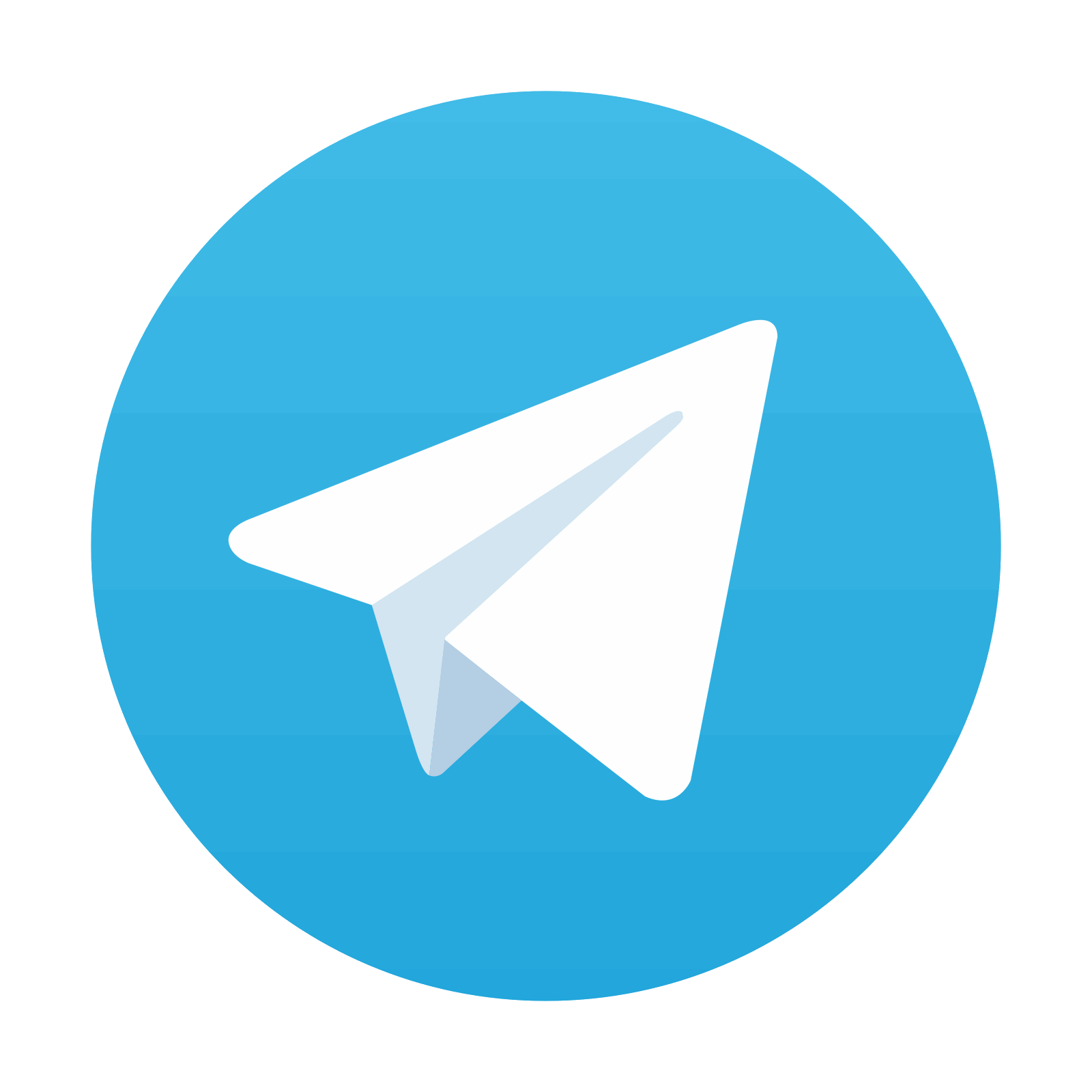
Stay updated, free articles. Join our Telegram channel

Full access? Get Clinical Tree
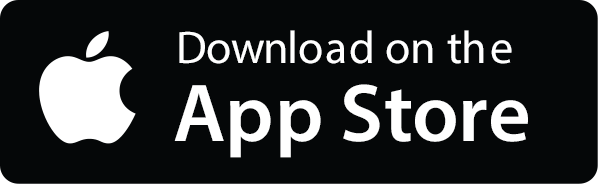
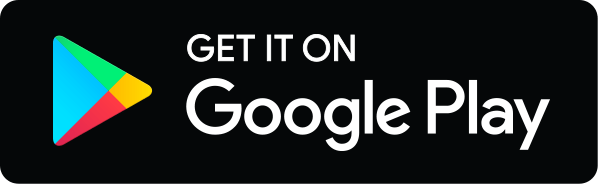