Fig. 5.1
Classification system of inner ear malformation which was relevant to the varying stages of inner ear organogenesis
5.2 Nonsyndromic Hearing Loss
5.2.1 DFNB4/Pendred Syndrome
DFNB4 is characterized by a congenital severe-to-profound sensorineural hearing loss (SNHL) . The hearing loss is described as bilateral, progressive, and fluctuant. There are episodes of sudden deterioration as well as vertigo. DFNB4 occurs as a recessively inherited disorder, genetically homogeneous, and is caused by biallelic mutations in the SLC26A4 [8]. SLC26A4 encodes a putative transmembrane protein designated pendrin, which functions as an anion transporter of chloride and iodide [9]. The SLC26A4 consists of 21 exons and is located on chromosome 7 [10]. Studies in the mouse inner ear have shown that pendrin is expressed in epithelial cells that participate in regulating the composition of inner ear fluids and plays a role in development of the inner ear and in maintenance of normal homeostasis [11]. Radiological studies have shown that an enlargement of the endolymphatic sac and duct in association with a dilated vestibular aqueduct was found in the majority of cases [12], as well as a Mondini-type hypoplasia of the cochlea [13]. Mondini cochlea is a shortened cochlea, rudimentary modiolus, missing interscalar septum, and partial agenesis of the organ of Corti and cochlear neurons [14]. Association of symptoms such as goiter and a partial defect in iodide organification which develops in early puberty or adulthood is defined as Pendred syndrome .
5.2.2 DFN3
DFN3 is characterized by a mixed conductive-sensorineural moderate-to-profound hearing loss and occurrence of a perilymph gusher upon attempted fenestration of the stapes. DFN3 accounts for about half of all cases of X-linked hearing loss. The sensorineural hearing loss may be progressive, and the conductive component of hearing loss is characterized by an air-bone gap in the lower frequencies, often with preservation of the stapedial reflex [15]. The causative gene for DFN3 is POU3F4 [16] which encodes a transcription factor POU3F4. POU3F4 belongs to the POU domain family and includes two functional domains, a POU-specific domain and a homeodomain [17]. POU3F4 is expressed in the mesenchymal cells surrounding the otic vesicle during development [18]. The endocochlear potential was decreased in mutant mice [19], which is thought to be the cause of hearing loss. Radiological studies typically demonstrate dilatation of the internal auditory canal (IAC), often with deficiency of the bone between the IAC and the basal turn of the cochlea [15, 20]. Moreover, deficiency of the bone between the IAC and the vestibule, enlargement of the vestibular aqueduct, the absence of cochlear bony modioli, enlarged labyrinthine facial nerve canals, and dilated singular nerve canals [21] have been suggested. Sennaroglu et al. proposed a new classification for this type of inner ear malformation, namely, incomplete partition type III [7].
5.3 Syndromic Hearing Loss
5.3.1 Waardenburg Syndrome
Waardenburg syndrome was reported to occur in 1 in 42,000 of the population or 1.43 % of the congenitally deaf [22]. The characteristic features were (1) dystopia canthorum (lateral displacement of the medial canthi and lacrimal puncta), (2) broad nasal root, (3) confluence of the medial portions of the eyebrows, (4) partial or total heterochromia iridis, (5) circumscribed albinism of the frontal head hair (white forelock), and (6) sensorineural hearing loss (bilateral or unilateral). Waardenburg syndrome has been divided into four types, depending on the phenotype and presence of additional features. Types I and II are distinguished from each other by the presence of dystopia canthorum (type I) or by its absence (type II). Type III is characterized by the presence of limb defects and is also referred to as Klein-Waardenburg syndrome. Type IV accompanies Hirschsprung disease, which is known as the Shah-Waardenburg syndrome. Types I and II are more common. Waardenburg syndrome is genetically heterogeneous, with mutations reported in a number of different genes, which generally encode for transcription factors. These particular transcription factors appear to be critically involved in the differentiation, migration, and function of melanocytes. Melanocytes are widely distributed within the cochlea and vestibular sense organs. The types I and III are caused by mutations in the PAX3 gene, which maps to 2q35. Inheritance is autosomal dominant [23]. The type II can be due to a mutation in the MITF gene, which maps to 3p14.1-p12.3, or in the SNAI2 gene, which maps to 8q11 [24]. Inheritance is autosomal dominant in mutations involving MITF and autosomal recessive for SNAI2. The type IV can be caused by mutations in the EDNRB gene [25], the EDN3 gene [26], or the SOX10 gene [27]. The type IV may be either autosomal recessive or dominant in its inheritance. Recent reports indicated that the EDNRB, the EDN3, and the SOX10 gene mutations could be involved in type II, although they were not a major cause of type II [28].
Recent reports have suggested that the frequency of hearing loss in type I is 58–75 % and in type II, 78–91 % [29–31]. The extents of loss and audiogram shapes are quite variable, ranging from no measurable clinical loss to severe congenital unilateral or bilateral sensorineural loss [30, 32]. Bilateral loss is more common. The hearing loss in type II has been found to be progressive in 70 % [32]. Abnormalities of the vestibular system are also common and may be seen in individuals who have normal hearing. Whereas the commonest pathological defect is of the Scheibe or cochleosaccular type, more major defects affecting the vestibular apparatus may occasionally be found.
5.3.2 BOR Syndrome
BOR syndrome is characterized by hearing loss, malformations of the external ear, branchial arch anomalies, and renal abnormalities. A mixed hearing loss is the most common type and the hearing loss is usually severe but can vary from mild to profound. Age of onset varies from early childhood to young adulthood. It is stable in majority of patients, although progressive hearing loss and fluctuant hearing loss have been described [33]. Malformations of the external ear include various types of abnormalities of the pinnae, stenosis of atresia of the external auditory canals, and the presence of helical or preauricular pits [34]. Mutations in the EYA1 or SIX1 have been identified to be the causative genes of BOR syndrome. EYA1 is the most frequent causative gene which was first reported by Abdelhak et al. EYA1 is located on chromosome 8q13.3 and acts as a protein phosphatase and transcriptional coactivator [35]. SIX1 is another causative gene located on chromosome 14q23.1 [36]. SIX1 interacts with EYA1 in transcriptional regulation and involves in the development of the mammalian ear and kidney [37–39]. Radiological studies show a wide variety of middle ear and inner ear abnormalities including malformations or absence of the oval window, enlargement of the vestibular aqueduct, and Mondini anomalies [40].
5.3.3 CHARGE Syndrome
The CHARGE syndrome was described with the diagnosis based on patients having at least four of the following six abnormalities: (1) coloboma, (2) heart anomalies, (3) atresia choanae, (4) retarded physical and central nervous system growth, (5) genital hypoplasia, and (6) ear anomalies with hearing loss. The ear is almost always affected. Most CHARGE ears are short and wide. The most detailed study [41] reveals hearing loss in approximately 85 % of patients. Although several studies documented mixed hearing loss due to ossicular anomalies and/or middle ear effusion, many authors reported predominantly or exclusively sensorineural hearing loss [42]. The sensorineural component ranged from mild to severe or profound and was suspected congenital. The majority of patients had sloping sensorineural losses. Guyot et al. [43] pointed out a specific form of unusual dysplasia of the labyrinth characterized by severe dysplasia or agenesis of the pars superior (utricle and canals) and Mondini anomaly of the pars inferior (cochlea and saccule). However, there appear to be exceptions to this rule .
Most cases with the CHARGE syndrome are sporadic, but there is evidence of familial transmission supporting autosomal dominant and autosomal recessive inheritance. CHD7 mutations occur in 32–64 % of patients with CHARGE syndrome features [44]. The most common mutations are nonsense and frameshift, but missense mutations can also occur. These various mutations cause haploinsufficiency of CHD7. In general, those with missense mutations tend to have milder and more variable phenotype than did those with truncating mutations [45]. The CHD7 protein appears to bind mostly to the DNA distal to transcriptional start sites of specific gene targets, enhancing their transcription either positively or negatively [46]. Abnormalities in the development, migration, or interaction of the cell of the neural crest may contribute to the pathogenesis of the CHARGE syndrome [47]. There has also been one report of a child with a clinical diagnosis of CHARGE syndrome who was found to have a mutation in semaphorin 3E (SEMA3E) [48].
5.3.4 Okihiro Syndrome
In 1977, Okihiro et al. [49] described a family of Duane syndrome (bilateral absence of adduction with widening on attempted abduction), most of whom had upper limb malformation and congenital severe sensorineural hearing loss. Inheritance is clearly autosomal dominant. Pathogenic mutations have been identified in the human SALL4 gene at 20q13 in affected individuals [50]. Reporting nonsense and frameshift mutations in five of eight families studied, Kohlhase et al. [50] drew attention to the clinical overlap with Holt-Oram syndrome, acro-renal-ocular syndrome, and cases mistakenly diagnosed as representing thalidomide embryopathy .
5.3.5 Distal Renal Tubular Acidosis (DRTA)
DRTA is characterized by dehydration, growth impairment, metabolic acidosis with alkaline urine, and hearing loss. Mild-to-profound SNHL, mainly at higher frequencies, is seen in childhood. There are several reports with the progressive hearing loss [51, 52]. The inherence pattern of DRTA is autosomal recessive, and mutations in two genes, ATP6V1B1 on chromosome 2p13 and ATP6V0A4 on chromosome 7p33-34, are responsible [53, 54]. ATP6V1B1 and ATP6V0A4 code for subunits of vacuolar H+-ATPase pump which serves to stabilize pH in both the kidney and inner ear [55]. Early SNHL occurs in most patients with ATP6V1B1 mutations, whereas late-onset SNHL is seen with ATP6V0A4 mutations [56]. High-resolution magnetic resonance imaging (MRI) performed in the patients demonstrated enlarged vestibular aqueducts, which can be unilateral or bilateral [55, 56].
5.4 Chromosomal Disorders Associated with Hearing Loss
5.4.1 Trisomy 21 (Down Syndrome)
Down syndrome, with an incidence of 1/600 live births, is the most common chromosome defect in humans. Ninety-five percent of Down syndrome is caused by an extra copy of a normal chromosome 21, such that the individual has a total of 47 chromosomes with three 21s (47,+21) in all cells. Two percent to four percent of cases are mosaic for the extra chromosome 21; that is, not all cells have trisomy 21. This can be the result of either meiotic or mitotic nondisjunction. In <5 % of Down syndrome cases, the additional 21 is caused by the presence of a chromosome rearrangement that results in the additional 21 being translocated to another chromosome. Most often, this occurs as a Robertsonian translocation, where the short arm of the 21 is translocated to the short arm of one of the other acrocentric chromosomes. These cases are significant in that the translocation can be inherited from a phenotypically normal parent, who is a balanced translocation carrier. Since 21 is the smallest chromosome with the fewest number of genes, the gene dosage imbalance resultant from the extra chromosome is one of the few autosomal trisomies tolerated during development, although only about 30 % of Down syndrome fetuses survive to the term [57].
The clinical manifestations are varied and include brachycephaly, upslanting palpebral fissures, epicanthic folds, short neck, flat nasal bridge, abnormalities of the pinnae, narrow palate, transverse palmar crease, mental retardation, congenital heart disease, short stature, duodenal atresia or stenosis, and cataracts [34]. The external ears tend to be small, apparently low set, and slightly posteriorly rotated. Temporal bones of Down syndrome patients show both middle and inner ear defects. Cochlear defects include Mondini dysplasia and overall hypoplasia in inner ear structures, including vestibular malformations and narrowing of the cochlear nerve canal [58]. Stapes malformations, residual mesenchyme obstructing the round window, and otitis media account for most middle ear structural abnormalities. Hearing loss is reported in over 80 % of children with Down syndrome [59]. Hearing loss can be conductive, sensorineural, or mixed .
5.4.2 Trisomy 18 (Edwards Syndrome)
The incidence is 1 in 5000 births. There is marked preponderance of females (3:1). Full trisomy 18 is the norm. A handful of mosaic cases have been reported [60]. Half of trisomy 18 newborns die within the first week; 90 % die within the first year of life [61]. Mosaicism for trisomy 18 may lead to partial expression of the phenotype, from mild to almost full expression. Among the constellations of abnormalities are hypoplasia of skeletal muscle; polyhydramnios; prominent occiput; narrow palpebral fissures; microstomia and micrognathia; microcephaly; clenched fist with the index finger overlapping the third and fourth fingers; shortened big toe; defects of the heart, lungs, and kidneys; hernias; cleft lip and/or palate; choanal atresia; slanting eyes; microphthalmia; low-set ears; and aural atresia [34]. Ears are low set, posteriorly rotated, and malformed and can have atresia of the external auditory canal. Temporal bone studies show abnormalities of the middle and inner ear, including failed ossification of the malleus, incus, and stapes, and retarded development of the cochlea [62]. It is likely that most babies with trisomy 18 are deaf or severely hearing impaired; however, audiometric analysis has not been reported .
5.4.3 Trisomy 13 (Patau Syndrome )
The incidence of trisomy 13 at birth is about 1 in 12,000. Babies born with trisomy 13 rarely survive more than a few days, and only 5 % survive to 6 months of age [57]. The majority of trisomy 13 cases are full free-lying trisomies, i.e., 47 chromosomes. Robertsonian translocation of a 13 onto another acrocentric chromosome has also been reported [63]. Among the anomalies are microcephaly and severe mental retardation, wide sagittal sutures and fontanels, gross anatomic defects of the brain, myelomeningocele, microphthalmia, iris colobomas, cleft lip and palate, antimongoloid slant eyes, simian palmar crease, polydactyly, rocker bottom feet, cardiac defects, low-set ears, and hearing loss [34]. Temporal bone studies show multiple abnormalities of the cochlea and vestibular systems, including semicircular canal and utricular, saccular, and macular anomalies; shortened cochlear length; widened cochlear aqueduct; and abnormalities of the modiolus and defects of the cochlear and vestibular nerves. Middle ear anomalies were also occasionally present [64]. Although hearing ability is often not evaluated because of the combined clinical and neurological impairments, one report noted at least two cases of documented hearing loss in mosaic trisomy 13 cases [63].
5.4.4 22q11.2 Deletion Syndrome (DiGeorge Syndrome)
The incidence of 22q11.2 deletion syndrome is 1 in 4000 [57]. DiGeorge syndrome is characterized by the agenesis of the thymus and parathyroid glands in association with other developmental anomalies of the third and fourth pharyngeal clefts, the cardiovascular and renal systems, and the craniofacial structures [34]. Eighty-five percent to Ninety percent of 22q11.2 deletion syndrome cases have a common deletion of 3 Mb at 22q11.2. Eight percent to ten percent of cases have a 1.5–2 Mb deletion at the same band [65]. The deletions are the result of chromosome 22-specific low-copy repeats that cause nonallelic homologous recombination. Studies in mice and humans have suggested that mutations in the TBX1 gene which maps to the center of the DiGeorge syndrome chromosomal region on 22q11.2 may be responsible for this phenotype [66]. Deletions at the second DiGeorge syndrome locus, 10p13, are larger and often seen on routine cytogenetics, but occur 50 times less frequently than the 22q11.2 deletions [67].
Patients with DiGeorge syndrome can have both external and inner ear defects. Auricular anomalies, one or more of which may be present in 80 % of cases, include small, low-set, or rotated ears; cupped or protruding ears; and helical anomalies [68]. More recent studies find 40 to 65 % of patients with hearing loss of greater than 25 dB in at least one ear [69, 70]; however, hearing deficits of more than 40 dB are relatively rare. The vast majority of hearing loss is conductive (70 to 90 %) and due to chronic otitis media. DiGeorge syndrome patients with a deletion at 10p13 compared to the classical 22q11.1 deletion appear to have a higher percentage of sensorineural hearing loss (41 % of patients). The hearing loss tends to be bilateral and progressive, ranging in a loss from 40 dB to profound loss [71]. Temporal bone studies note a variety of defects in some 22q11.2 deletion syndrome patients, including Mondini dysplasia, shortened cochlea, and defects of the outer and middle ear such as atresia of the external auditory canals and ossicular defects [70].
5.5 Perspective
Since the next-generation sequencing was introduced for the genetic analysis of hearing loss [72, 73], the discovery of novel deafness genes and genetic diagnosis of hearing loss have been greatly facilitated. Although causative genes are identified for only a part of inner ear malformation and cochlear nerve deficiency at present, the situation is likely to change rapidly. With accumulation of genotype-phenotype information for these auditory disorders, explanation for the causes and mechanisms of hearing loss will become more widely available, planning of medical care will be more effective, and genetic counseling will get more precise. Even without genetic testing, such information would contribute to physicians in understanding and predicting the causes and clinical characteristics of each type of the anomaly, which would benefit the medical intervention for patients who do not want genetic tests or prior to genetic tests.
References
1.
Merchant SN. Anomalies of the inner ear. In: Merchant SN, Nadol JB, editors. Schuknecht’s pathology of the ear. 3rd ed. Shelton: People’s Medical Publishing House-USA; 2010. p. 262–77.
2.
Chan KH, Eelkema EA, Furman JM, Kamerer DB. Familial sensorineural hearing loss: a correlative study of audiologic, radiographic, and vestibular findings. Ann Otol Rhinol Laryngol. 1991;100(8):620–5.CrossRefPubMed
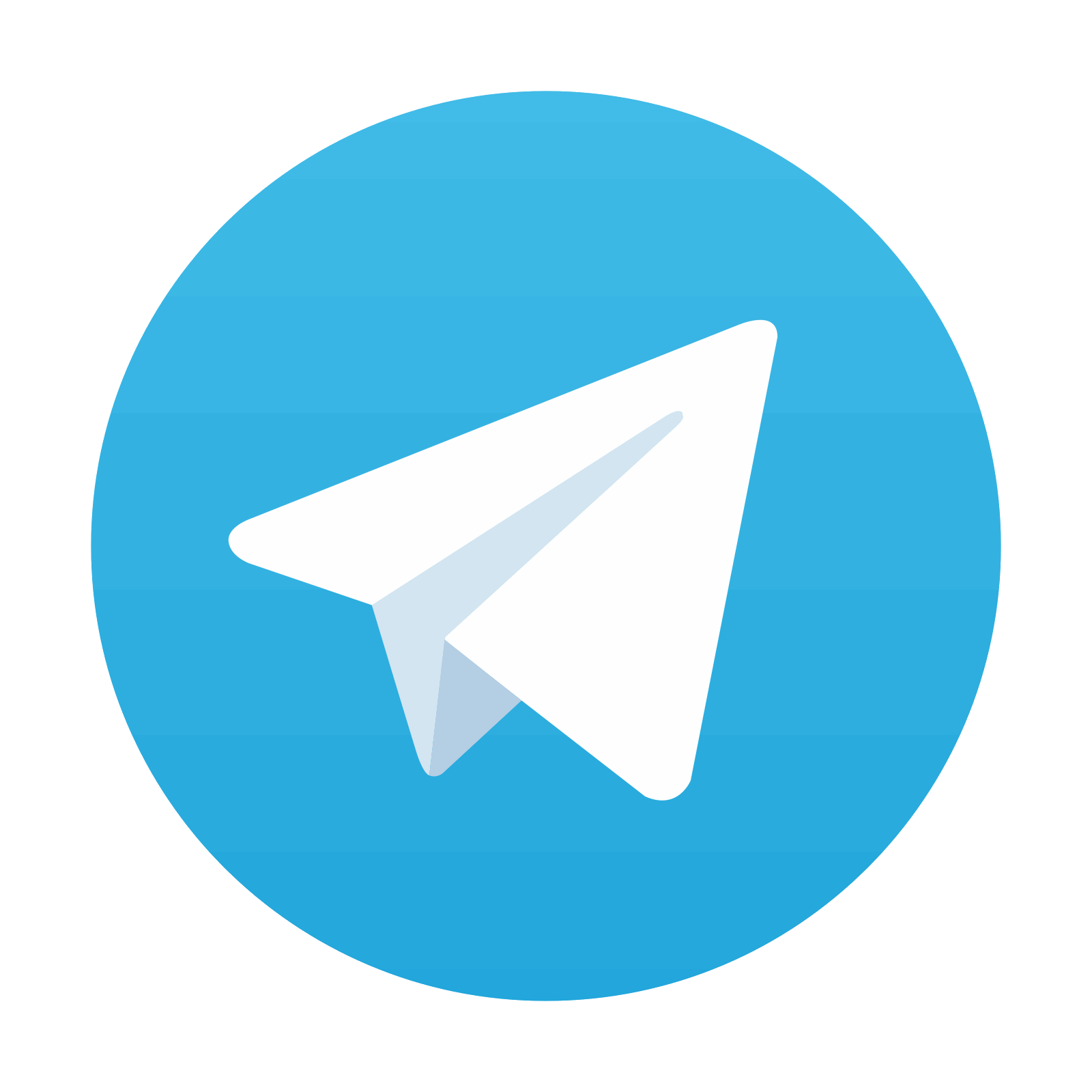
Stay updated, free articles. Join our Telegram channel

Full access? Get Clinical Tree
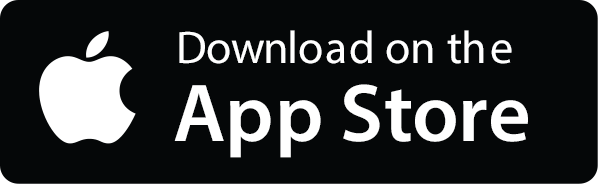
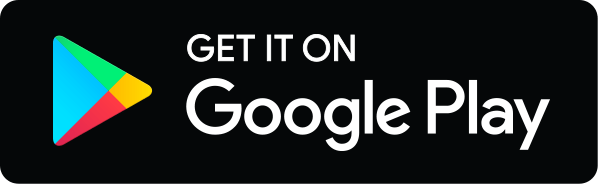