Genetics of Congenital Cataracts
Lucy T. Xu
Elias I. Traboulsi
THE DEVELOPING LENS
Embryology of the Lens
The eye starts developing on the 22nd day of gestation.1 Shallow grooves first form on the opposite sides of the forebrain, eventually developing into outpockets called optic vesicles.1 The vesicle comes into contact with the surface ectoderm and induces the differentiation of ectodermal cells into the lens placode.1 The optic vesicle then invaginates and forms the optic cup and stalk.1 The lens placodes invaginate as well to form the lens vesicles on both sides.1 By the 5th week, the lens vesicle loses contact with the surface ectoderm and is located in the front part of the optic cup (Fig. 1.1).1,2
Within the lens vesicle, the anterior cells give rise to a sheet of cuboidal epithelial cells.1 The posterior vesicle cells elongate to reach the anterior wall by the end of week 7.1 These long cells form the primary lens fibers filled with lens-specific proteins such as crystallins.1,3
New secondary fibers are continuously added from the germinative zone—the section of epithelium just above the equator.4 Proliferation occurs rapidly here, with daughter cells migrating below the equator where they differentiate and elongate into secondary fiber cells.4 These new crescent-shaped cells organize in concentric circles over older cells while synthesizing crystallins.4 As these secondary fiber cells migrate through successive zones, they develop membrane undulations and eventually lose their cellular organelles to become compacted containers of protein solution.4 Eventually, the mature secondary fiber cells overlap in specific arc segments known as sutures.4 At birth, the suture pattern consists of three branches shaped as a Y.4 As the lens grows, the sutures become more complex, eventually forming six, nine, and then twelve branch sutures.4 The mature lens consists of an ordered organization of epithelial and fiber cells, with peripheral fiber cells meeting at the sutures.5
Regulation of Lens Development
Lens differentiation is regulated by many transcription factors. Fibroblast growth factor (FGF) induces cell proliferation in low doses, whereas it induces cell migration and fiber cell differentiation at higher doses.6 Thus, the polarity of the lens may be due to the varying concentrations of FGF in the ocular media, with more FGF found in the vitreous than the aqueous.6 Bone morphogenetic protein (BMP) has also been shown to interact with FGF during early lens induction.6 For proper lens induction to occur, the canonical Wnt/β-catenin pathway must be down-regulated, while the antagonistic noncanonical Wnt pathway must be up-regulated.6 The Wnt/β-catenin pathway has also been shown to play a role in beta crys-tallin production.6 Finally, paired box 6 (PAX6) is crucial for the formation of the lens placode and the expression of crystallin genes.7 Crystallins are essential components of the lens, and mutations in crystallin genes account for many of the inherited nonsyndromic congenital cataracts.
CRYSTALLINS
Crystallins are major protein components of the lens. They constitute over 90% of the proteins in each fiber cell.8 They are crucial in maintaining lens transparency.9 Mutations in crystallin genes represent a large portion
of autosomal dominant congenital cataracts.10 Mutations have been identified in αA-crystallin (CRYAA), αB-crystallin (CRYAB), βA1/A3-crystallin (CRYBA1/A3), βB1-crystallin (CRYBB1), βB2-crystallin (CRYBB2), γC-crystallin (CRYGC), and γD-crystallin (CRYGD).10 The β- and γ-crystallins form a superfamily called the βγ-crystallins that share a common polypeptide chain fold.11 The α-crystallins form a separate family due to their relation to small heat shock proteins.11
of autosomal dominant congenital cataracts.10 Mutations have been identified in αA-crystallin (CRYAA), αB-crystallin (CRYAB), βA1/A3-crystallin (CRYBA1/A3), βB1-crystallin (CRYBB1), βB2-crystallin (CRYBB2), γC-crystallin (CRYGC), and γD-crystallin (CRYGD).10 The β- and γ-crystallins form a superfamily called the βγ-crystallins that share a common polypeptide chain fold.11 The α-crystallins form a separate family due to their relation to small heat shock proteins.11
![]() Figure 1.1. In the development of the eye, grooves in the forebrain develop into optic vesicles. The vesicle comes into contact with the surface ectoderm and induces the differentiation of ectodermal cells into the lens placode. This process is regulated by Pax6 and Sox2. The lens placode invaginates to form the lens pit as regulated by Mab21 and L-Maf. The lens pit will eventually detach from the surface ectoderm to produce the lens vesicle. The formation of primary lens fibers (PLF) and the embryonic nucleus is regulated by FoxE3 and α-crystallin genes. The role of the genes is in part derived from animal experiments.2 |
α-Crystallins
α-Crystallins constitute approximately 35% of the lens.12 They are small heat shock proteins that contribute to the refractive power of the lens, and act as a molecular chaperone.12 Specifically, α-crystallins bind unfolded and denatured proteins in the lens to prevent aggregation.12 Aggregated proteins will interfere with the refractive properties of the lens, leading to the formation of cataracts.12
Two types of α-crystallins exist in the lens: αA-crystallins and αB-crystallins. The αA-crystallin gene (CRYAA) is on chromosome 21 and encodes a 173 amino acid protein.12 The αB-crystallin gene (CRYAB) is found on chromosome 11 and encodes a 175 amino acid protein.12 The two α-crystallins share a 57% homology.12 αA-crystallin is expressed solely in the lens while αB-crystallin is expressed systemically. The ratio of αA-crystallin to αB-crystallin in the lens is 3:1.12
Although α-crystallins are generally stable enough to tolerate many substitutions in its primary structure, certain mutations have been implicated in the formation of congenital cataracts.12 These mutations alter the tertiary and quaternary structure of the protein, impinging on its ability to bind substrate proteins.10 Abnormal oligomerization of mutated α-crystallins have been found in congenital cataracts.10 These changes decrease the stability of the protein and reduce its chaperone ability.10 Mutations have also affected posttranslational modifications that lead to misfolded proteins that form aggregates and scatter light.13
An amino acid substitution mutation in the CRYAB gene leads to congenital cataracts but also systemic manifestations, since αB-crystallin is expressed ubiquitously.14 An A>G transition at nucleotide 3787 in the genomic sequence translates to the replacement of arginine at position 120 by glycine.14 The p.R120G mutation causes desmin-related myopathy, which is an autosomal dominant disease characterized by muscle weakness, cardiomyopathy, and congenital cataracts.14 Muscle biopsies show aggregates of desmin possibly due to altered interactions between desmin and the mutated αB-crystallin.14
A deletion in exon 3 (450delA) of the CRYAB gene leads to a frameshift mutation that manifests clinically as an isolated congenital cataract, inherited in an autosomal dominant pattern.13 The cataracts are described as bilateral posterior polar.13 Because the opacity is in the back of the lens and thus close to the optical center of the eye, visual acuity is severely affected.13 The opacity was either present at birth or developed within the first few months after birth but did not progress to any other parts of the lens.13
β-Crystallins
β-Crystallins are the most abundant water-soluble proteins in the lens, and their specific, closely packed arrangements play an important role in maintaining lens transparency.9 β- and γ-crystallins are very similar in structure. They both have two domains connected by an 8-10-amino acid peptide.11 Each domain is composed of an identical polypeptide chain fold in a Greek key motif: a β-sandwich of two antiparallel β-sheets. Normally, βA3- and βB2-crystallins associate reversibly into homo- or heterodimers.11 These dimers may either dissociate into monomers or further associate into multimeric complexes.11 It is thought that the multimeric complexes are essential in maintaining lens transparency.11 Mutations that disrupt the protein structure or change its ability to associate can cause nonspecific aggregates of these crystallins that precipitate cataract formation.
Padma et al. described a family with isolated congenital cataracts due to a mutation in the CRYBA1 gene that encodes βA3/A1-crystallin.15 Like most isolated congenital cataracts, it is inherited in an autosomal dominant fashion.15 All affected members exhibited both zonular and sutural cataracts.15 The fetal and embryonic nuclei in the zonular cataract were clear, while the sutural cataract involved an anterior erect Y-shape and a posterior inverted Y-shape.15
A chain termination mutation in the CRYBB2 gene that encodes for the β-2-crystallin protein has been implicated in a cerulean-type cataract.16,17 The cataracts manifest as blue flakes and spoke-like central opacities with only mild reductions in visual acuity.16
An autosomal recessive mutation in the CRYBB3 gene has been shown to cause a nuclear cataract with cortical riders.9 While crystallin-causing cataracts often follow an autosomal dominant inheritance pattern due to the structural function of the proteins, this autosomal recessive inheritance suggests that βB3-crystallin may play a role beyond that of structure, such as an enzyme or developmental protein.9
γ-Crystallins
The γ-crystallins are part of the βγ-crystallin superfamily and like the β-crystallins, they form a Greek key motif with four β-sheets. It is thought that the γ-crystallins also contribute to the maintenance of lens transparency in a similar way to that of the β-crystallins. The γ-crystallins gene cluster, (CRYGA-F) is located in the loci 2q33-35.18 Mutations that cause cataracts have been identified mainly in the CRYGC and CRYGD genes, which also happen to the most expressed γ-crystallin genes in humans.18 The γS-crystallin gene, formerly known as βS-crystallin, is distinct from other γ-crystallins.19 γS-crystallin is found on chromosome 3, and the protein is structurally similar to the other γ-crystallins except in that it has an additional α-helix between the third and fourth β-sheets.11
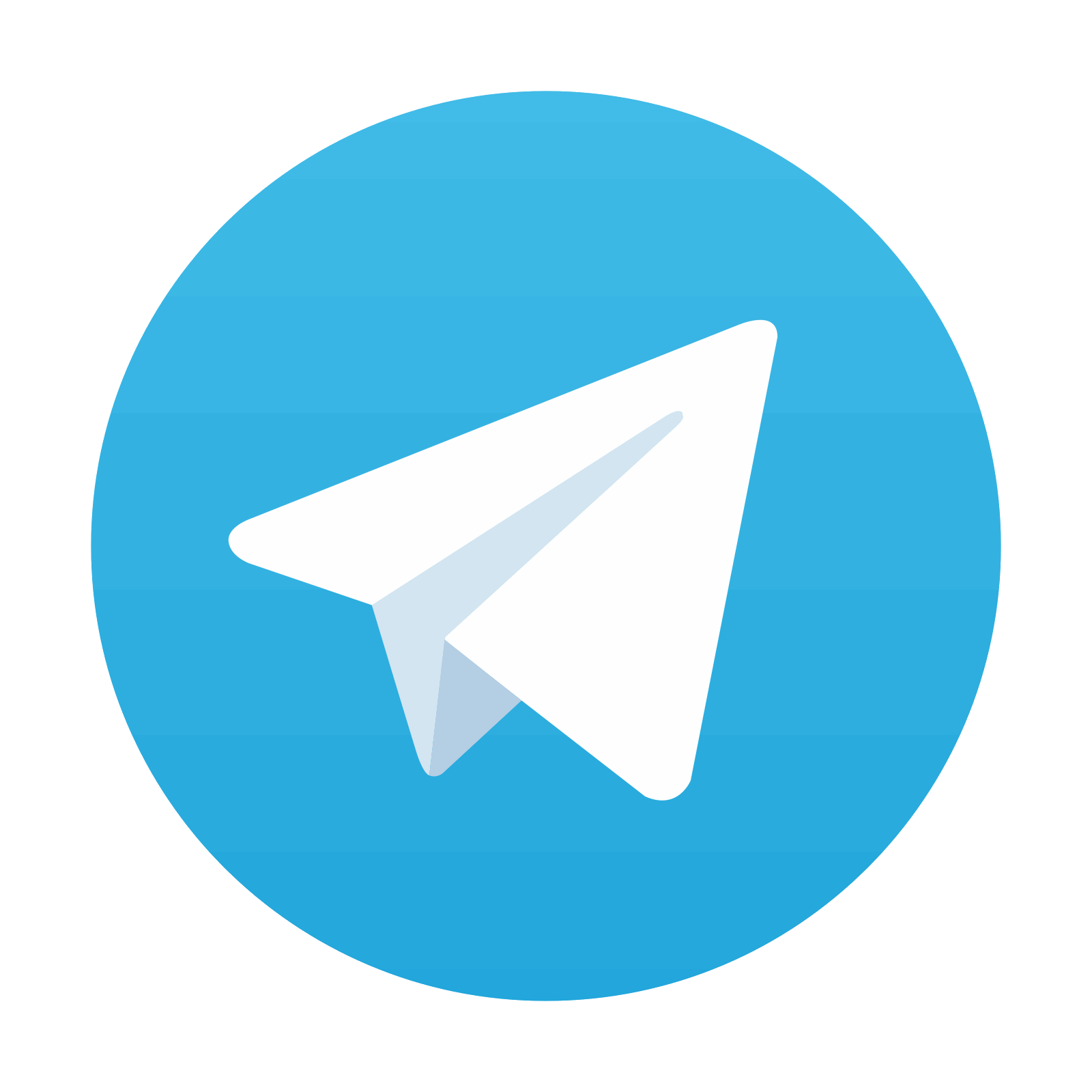
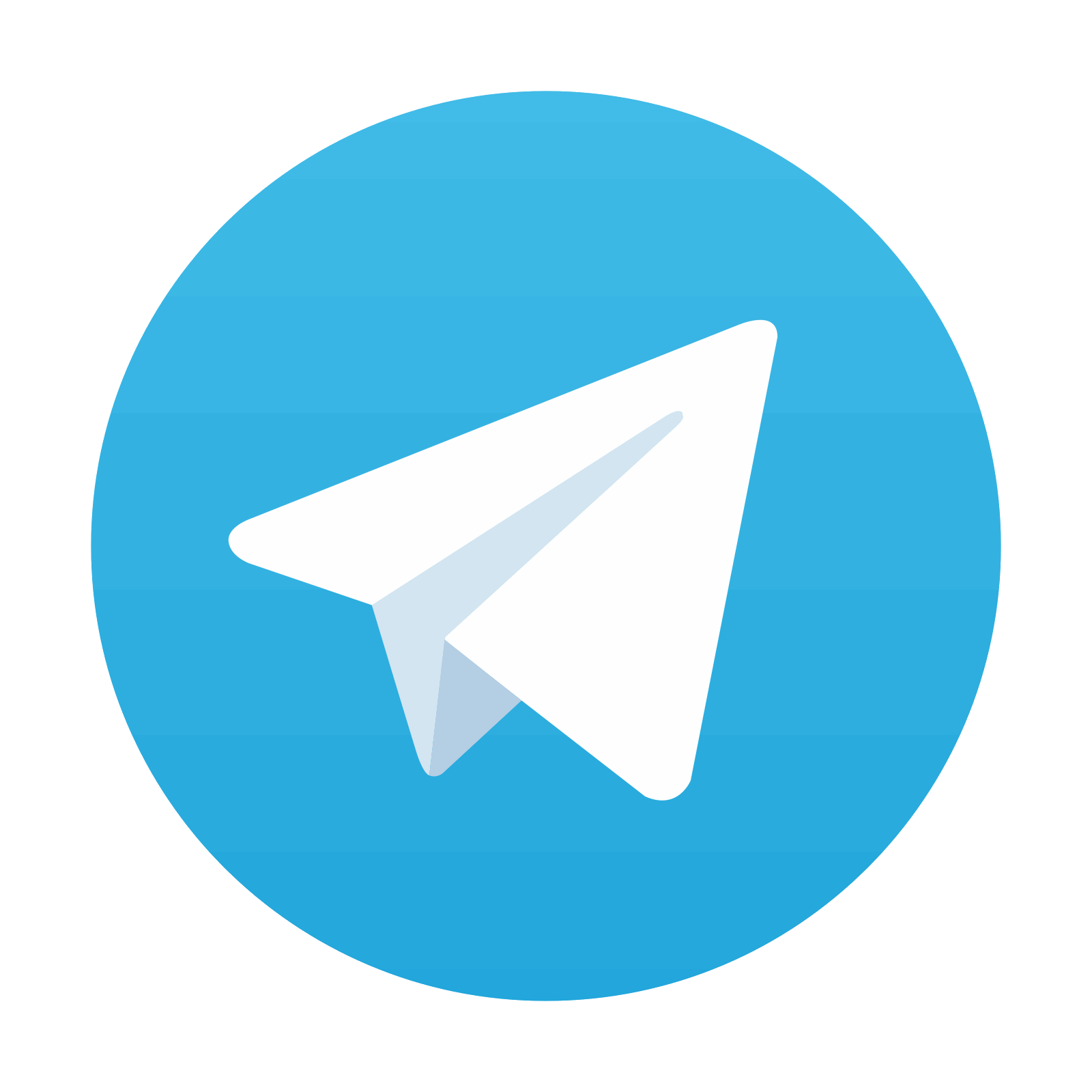
Stay updated, free articles. Join our Telegram channel

Full access? Get Clinical Tree
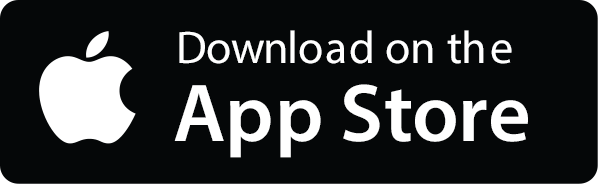
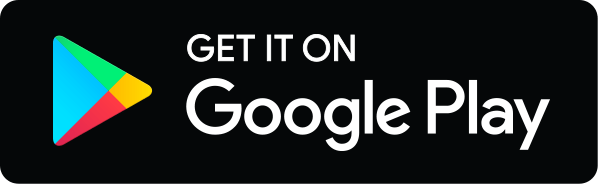
