Molecular genetic testing is one of the cornerstones for the care of patients with genetic ocular disease and their families. The results of these tests provide many benefits, including confirmation or ruling out of an underlying genetic disorder or helping to determine the risk of inheriting a genetic disease. With many options for studying the genetic etiology of a disorder, one should consider the target and goal of testing, cost, availability, possible unintended outcomes, risks, limitations, and benefits.
Molecular tests can range from the search for a specific mutation in a single gene to sequencing of the entire genome. Chromosomal tests analyze whole chromosomes or specific lengths of deoxyribonucleic acid (DNA) to identify deletions, duplications, or rearrangements of chromosomal material. Biochemical tests study the quantity or functional level of proteins, which can suggest causative DNA changes that result in a specific disorder.
Genetic testing is voluntary. Because these tests have benefits as well as risks, patients should always be well informed prior to the initiation of testing. A clinical/ocular geneticist or genetic counselor can provide valuable information about the limitations, advantages and disadvantages of a particular test, risks (e.g., discovery of unreported nonpaternity, identification of a health risk unrelated to the indication for testing), and the potential social, insurance, and emotional implications of genetic testing. Counseling should also include information on the nature, inheritance, and implications of the genetic disorder for which testing is being ordered. Similar counseling should be performed after the results of the test become available.
3.1.1 Karyotype
Karyotype is a test performed with a light microscope, and describes the number and appearance of chromosomes in the nucleus of a eukaryotic cell (▶ Fig. 3.1). Chromosomes are removed from the nucleus at a specified stage of the cell cycle to allow for a desired length of chromosome and then stained with a variety of chemicals to create a characteristic banding pattern, which allows for identification of specific regions on each chromosome. Variables analyzed include large gains or losses of chromosomal material, chromosome number, alteration in chromosomal position or configuration, and the presence of satellite/marker material. To identify possible mosaicism, more than one cell is analyzed. A karyotype is requested when there is no specific recognizable single gene syndrome and more than two involved organ systems, when there is a phenotype that is specific for a chromosomal aberration (e.g., trisomy 21), or the family history suggests a chromosomal basis (e.g., multiple miscarriages due to unbalanced translocation).
Fig. 3.1 Human female karyotype.
(This image is provided courtesy of Silvia Castillo, MD.)
3.1.2 Chromosomal Microarray
Chromosomal microarray (CMA) should be considered when a karyotype is normal, but the clinical picture is still highly suggestive of a chromosomal aberration. CMA is a microchip-based testing platform that allows high-volume, automated analysis of many segments of DNA at once. The entire nuclear genome can be screened for submicroscopic copy number variations (CNV): deletions or duplications. These changes are too small to be seen under a light microscope, but may contain multiple genes, and therefore lead to a disease phenotype. Internet databases allow the coordinates of an identified CNV to be entered and affected genes in the particular region are displayed (see section “Mutation and Copy Number Variation Databases”). Associated known phenotypes are also available in the medical literature. The physician then correlates these findings with the phenotype of an affected individual. Genes within the CNV may not be clearly related to the patient’s condition, thus requiring further investigation. CMA may also detect regions of homozygosity, which may be suggestive of risk for recessive disease or imprinting disorders. CMA does not detect balanced translocations, sequence variations within a single gene, or low-level mosaicism (usually below 20%). There may also be intragenic deletions or duplications that are smaller than the resolution of CMA. Deletion/duplication analysis (del/dup) for intragenic changes too small for detection by CMA may be found using probe amplification methods. Deletion and duplication analysis should be considered when sequencing is negative but the clinical picture is very suggestive of a particular syndrome caused by mutations in that gene or when a mutation is found on only one allele for a presumed autosomal recessive disease.
3.1.3 Mutation and Copy Number Variation Databases (Accessed June 2016)
LOVD: Leiden Open Variation Database:
http://www.lovd.nl/3.0/home.
RetNet:
https://sph.uth.edu/retnet/.
CentoMD:
https://info.centomd.com/.
Retina International Mutation Database:
http://www.retina-international.org/sci-news/databases/mutation-database/.
RetinoGenetics:
http://www.retinogenetics.org.
Keio Mutation Databases: KmeyeDB:
http://mutview.dmb.med.keio.ac.jp/MutationView/jsp/index.jsp.
University of California, Santa Cruz (UCSC) Genome Bioinformatics:
https://genome.ucsc.edu/.
DECIPHER:
http://decipher.sanger.ac.uk.
3.1.4 Fluorescein In Situ Hybridization
Fluorescein in situ hybridization (FISH) involves the labeling of metaphase chromosomes with fluorescent probes that bind to specific target regions and thus identify if these regions are deleted or duplicated. The selection of FISH requires a known target region for examination as seen for conditions such as Prader–Willi/Angelman syndrome (del15q11-q13), 22q11 deletion syndrome (del22q11.2), Cri-du-chat syndrome (del5pter), retinoblastoma with systemic malformations (del13q14.1), or Wilms tumor, Anirida, Genitourinary anomalies, Retardation of growth and development, del(11)(p13) (WAGR) syndrome (del11p13). FISH has the advantage of being able to find abnormal cells more easily than standard cytogenetic methods, not requiring living cells and its ability to be quantified automatically. However, a trained technologist is required to distinguish subtle abnormalities.
3.1.5 Gene Sequencing
Gene sequencing is the test of choice when monogenic disease is suspected. Sequencing is the process of defining the precise order of nucleotides within the gene DNA. Sanger sequencing has been the “gold standard” method for many years. It is based on the selective incorporation of chain-terminating dideoxynucleotides by DNA polymerase during in vitro DNA replication. More recently, next-generation sequencing (NGS) methods have allowed for large-scale, automated gene studies including multigene panels where numerous genes can be sequenced in parallel. The Sanger method is still widely used for validation of next-generation results.
3.1.6 Whole Exome Sequencing
Whole exome sequencing (WES) is a technique for simultaneous sequencing of all known coding genes in the nuclear genome, using the NGS technology. This test could render a molecular diagnosis in the setting of a nonspecific phenotype or when standard diagnostic testing has been exhausted. It may also be cost efficient as compared to multiple specific tests. WES is only able to identify those mutations found in coding regions (although some protocols will involve some exon–intron boundaries or deeper intronic sequencing). Approximately 99% of the human genome is not covered using WES.
With WES and whole genome sequencing (WGS; see section “Whole Genome Sequencing”), there is potential for the recognition of incidental findings unrelated to the indication of the test, but which may or may not be valuable for the patient. The American College of Medical Genetics (ACMG) published a policy statement on clinical WES that emphasizes the importance of disclosing the possibility of such results in pretest patient counseling. A gene list of “medically actionable” genes requiring mandatory disclosure if mutated is provided (because of the potential for important preventative interventions). WES has the potential to uncover changes in many other genes as well, which may be unrelated to the patient’s phenotype and not actionable. Therefore, most testing laboratories conduct a “filter” of the generated data such that results given to the patient, which are not on the ACMG mandatory disclosure list, are only for those genes felt to be potentially relevant to the phenotype. Another option offered by some laboratories for managing the risk of excessive and unwanted information is to create customized panels using exome testing that restrict result reporting to specific genes, a process very similar to the next-generation array sequencing panels.
3.1.7 Mitochondrial Genome Sequencing
Mitochondrial diseases are a heterogeneous group of disorders that arise as a result of dysfunction of the mitochondrial respiratory chain. They are associated with mutations of genes encoded by either nuclear DNA or mitochondrial DNA (mtDNA). There are different options for studying mitochondrial DNA, including specific panels (e.g., targeted sequencing for Leber hereditary optic neuropathy [LHON]), sequencing of the entire mtDNA, or whole mitome sequencing, which includes all mtDNA and nuclear genes that encode mitochondrial proteins.
3.1.8 Whole Genome Sequencing
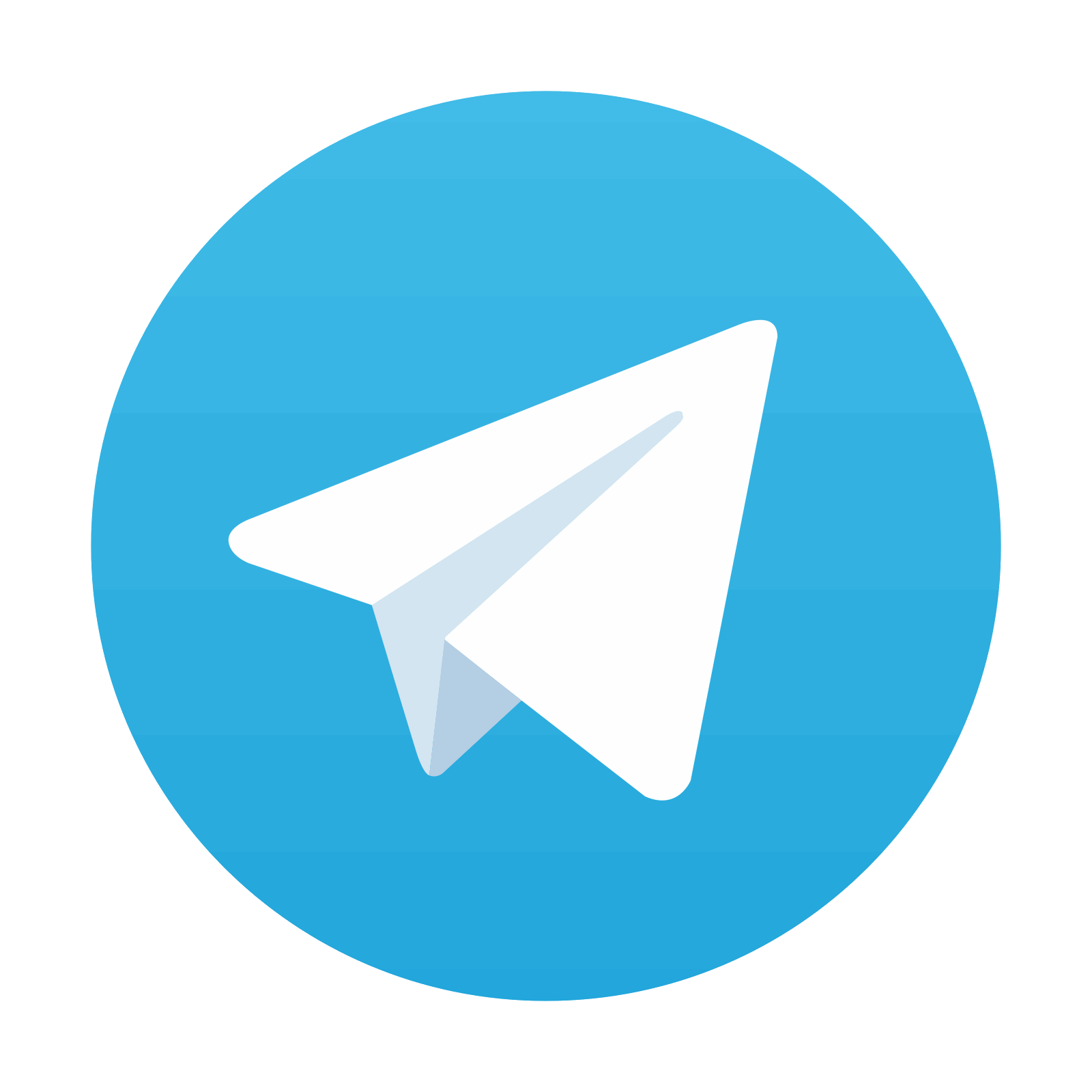
Stay updated, free articles. Join our Telegram channel

Full access? Get Clinical Tree
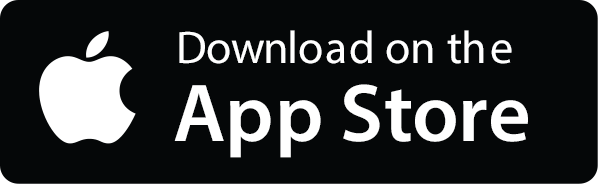
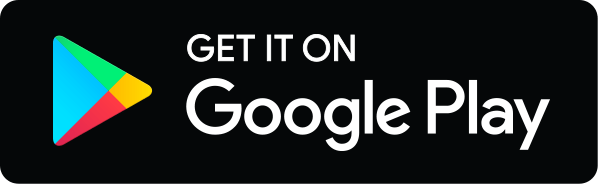