57
Gene Therapy for Retinal Disease
Peter Gehlbach
VECTORS FOR GENE THERAPY
Nonviral Vectors
Nonviral vectors have potential advantages. Most of them are safe and nontoxic to ocular tissues. The major drawback of nonviral vectors is their low transfection rate and relatively short life. The particular characteristics of the target tissue (or cell), the specific therapeutic material to be used, and the expected beneficial outcome, determine the selection of the most appropriate route of delivery. This makes route of delivery an important aspect when discussing nonviral vectors. For the eye, topical instillation, periocular, or intraocular (intravitreal or subretinal) injections are the most widely used. Systemic administration, on the other hand, is seldom considered due to the presence of blood-ocular barriers and the potential secondary side effects.
Topical Instillation
Instillation of an active compound is the first choice method of delivery for ocular therapy. However, due to the innate protective characteristics of the eye against the entry of foreign compounds (tear drainage and corneal epithelium barrier), the bioavailability of an instilled compound is generally low. To overcome this limiting factor, frequent instillations or the use of specific formulations which increase the corneal contact time have been developed. Despite these improvements, after instillation, drug levels within the posterior segment of the eye are, in most instances negligible. Plasmids showed better intracellular delivery after delivery via liposomes as well as via polyplexes. Oligodeoxynucleotides (ODNs) showed very localized expression to the anterior layers of the eye which was not greatly improved by delivery in liposomes.
Intracameral and Intracorneal Injections
Intracameral injections are rarely used in clinic because of the rapid turnover of aqueous humor resulting in very short-time contact time between the active compound and the tissue. On the other hand, injection into the corneal stroma may enhance the transfection of corneal cells because it induce mechanical pressure and allows for a prolonged direct contact of the nucleic acids with the target cells. Naked plasmids delivered intrastromally resulted in sustained corneal expression and did not induce any inflammation. When delivered in liposomes they showed better penetration and a wider distribution, this may be due to their relatively large size, causing their accumulation in the aqueous humor-draining pathways, delaying the outflow and increasing the contact time with ocular tissues. ODN showed low transfection rates but their delivery to the anterior segment was enhanced when delivered in liposomes. Ribonucleic acid (RNA) aptamer were delivered in sustained-release pellets of hydron polymer. Rat cornea neovascularization was inhibited by pellets containing an RNA aptamer using the corneal micropockets system.
Subconjunctival Delivery
Subconjunctival injection is minimally invasive and can be repeated. The subconjunctival space allows for the injection of large volumes and can be used as a reservoir. Thus it may replace the need for repeated instillations. After subconjunctival injections, the intraocular penetration depends on the physicochemical properties of the active compound used. In general, high levels of drugs may be detected in the anterior and the posterior segments of the eye. However, high systemic absorption has also been observed.
Naked plasmids delivered only transfect periocular tissues, and showed enhanced transfection of the corneal cells when delivered in liposomes. Using collagen shield has increased markedly the gene expression within tissues due to the slow release of plasmids in the subconjunctival space. ODNs and siRNAs are smaller and allow for potential targeting of corneal cells after subconjunctival injections.
Intravitreous Injection
Direct injection of active compounds into the vitreous cavity is the simplest way to target intraocular tissues. However, molecules injected into the vitreous are cleared rapidly through diffusion anteriorly or transretinally. Therefore, longstanding vitreous therapeutic levels can be achieved either by repeated injection, the use of high initial concentrations or slow release formulations. Shortly after vitreous injection, initial high peak of local drug levels can be toxic to adjacent ocular tissues. Complications, such as vitreous hemorrhage, endophthalmitis, and/or retinal tear, although rare, may occur after intravitreal injection. These risks are obviously increased when repeated injections are given. Following intravitreous injection of naked plasmids, no significant expression of the reporter gene as observed in the retina (1–3). Liposomes have been used to increase the half-life time of intravitreally injected nucleic acids and reduce their toxicity. Plasmid encapsulation within liposomes allows for the transfection of cells in the anterior segment of the eye, inner retinal layers, and retinal pigmented epithelial (RPE) cells. Liposomes also increase the stability of ODNs in the vitreous and to increase the transfection efficiency of liposomes, strategies such as pegylation have been developed were oligo-aptamers coupled to polyethylene glycol (PEG) were formulated. ODNs have also been delivered in nanoparticles; VP22 is a cationic peptide that form well characterized nanoparticles (vectosomes) when complexed with ODNs. We have observed that after intravitreous injection in the rat eye, vectosomes followed a transretinal migration accumulating in the cytoplasm of RPE cells. The VP22 vectosomes show a specific sensitivity to light. After their internalization by the RPE cells, the vectosomes remain stable within the cytoplasm. However, when the cells are illuminated, the vectosomes are destabilized releasing their ODN. The released ODN then moves from the cytoplasm to the cell nucleus expressing its genetic load. Therefore, they can be used to control the release of ODNs at chosen sites using white light (or laser) beam.
Subretinal Delivery
To increase the local concentration of active compounds in the posterior retinal layers (photoreceptors and RPE cells) and prolong their contact time with the target cells, direct injection in the subretinal space can be performed. Injections can be carried out externally through the sclera or internally through the retina entering into the eye via the pars plana. These types of injections require the use of pressure and fluid or air to detach the retina. The disadvantage of this method is that the area of targeted cells is limited to the locally detached retina. Furthermore, multiple complications may occur: Of these, most notable are lesions of RPE cells, hemorrhages, retinal tears, subretinal or preretinal fibrosis, and uncontrolled retinal detachments. Subretinal delivery of nucleic acids has been used to target RPE and photoreceptors in preclinical studies as a proof of concept. However, due to the expected high rate of complications, particularly if reinjections are needed. and the potential difficulty of detaching a pathological retina where subretinal fibrosis takes place, subretinal injections may remain an investigative tool and not a practical method for application to the human eye.
Viral Vectors
Adenoviral (Ad) vectors are relatively easy to produce, have good capacity, and with an appropriate promoter can mediate good expression levels in many types of cells. The transduction efficiency for a particular cell type may vary depending upon the serotype and other characteristics of the Ad vector (4). There is little or no transduction of neuronal cells in the retina. Subretinal injections result in strong transduction of RPE cells and occasional Muller cells, but little or no transduction of retinal neurons. The major concern with Ad vectors is that they induce an immune response that results in inflammation, mediates destruction of transduced cells reducing transgene expression, and prevents repeated injections; however, excellent safety profiles in two recent clinical trials suggest that clinical grade second generation Ad vectors are well tolerated in the eyes of humans.
Recombinant Ad vectors in which all viral genes have been deleted are generally called encapsidated adenovirus mini-chromosomes or “gutless Ad vectors” and invoke much less immune response, transduce rod photoreceptors, and mediate much longer transgene expression.
Adeno-associated viral (AAV) vectors are substantially more difficult to produce than Ad vectors and have limited capacity of less than 5 kb. However, AAV vectors appear to invoke little or no immune response and therefore have little toxicity and mediate prolonged transgene expression. Intravitreous injections of AAV vectors result in transduction of retinal ganglion cells and subretinal injections result in transduction of RPE cells and photoreceptors.
Lentiviral vectors efficiently transduce a variety of nondividing cells with little or no host response resulting in long-term transgene expression. Subretinal injection of a human immunodeficiency virus (HIV) vector containing the green fluorescent protein (GFP) gene under the control of the cytomegalovirus (CMV) promoter resulted in widespread expression of GFP in photoreceptors and RPE cells, for at least 12 weeks, the longest time point examined. Contamination of HIV vector preparations with replication competent virus is unlikely, but because of its serious consequences it is still a concern. Bovine immunodeficiency virus (BIV) is a lentivirus that is not known to cause human disease. Subretinal injection of a BIV. GFP vector resulted in rapid and prolonged transduction of RPE cells with no evidence of an inflammatory response.
Applications of Gene Therapy in Macular Diseases Macular Edema and Ischemia: Antiapoptosis Gene Therapy and Neuroprotection
Macular ischemia is the result of an insufficient blood supply to neurons due to occlusion of blood vessels As a consequence, retinal cells undergo hypoxia causing a number of pathophysiological processes, including the production of glutamate and free radicals, that ultimately end in damage to retinal neurons and cell death by apoptosis (5–10). The current therapies aim at re-establishing the retinal microcirculation through using laser treatment, ocular massage, and tPA. However, these therapies have had limited success and need to be applied early in the course of ischemia to be effective. Other agents that provide neuroprotection through delaying cell death of ischemic retina have also been tested; however, they do not permanently rescue the retinal cells (11, 12). It appears that in order to rescue retinal cells from apoptosis, a neuroprotectant should affect the apoptotic mechanism itself.
Apoptosis appears to be the final pathway of cell death in retinal cells (13, 14). Pro and antiapoptotic genes have been identified and tested on retinal cells. The transfer of bcl-2 gene (an antiapoptotic gene) to the retinal cells resulted in a delay in retinal degeneration in mice (15). However the transfer of this same gene to the ganglion cells resulted in increased glutamate-induced apoptosis (16). Thus the protective effect of bcl-2 may be tissue specific, or may be mediated by secondary pathways that are differ among different retinal cells.
A proapoptotic protein, caspase-3, when unregulated causes retinal degeneration in transgenic rats (17), and hence inhibition of its expression could inhibit apoptosis (18).
The final pathway in the apoptotic pathway is the processing and activation of caspases that dismantle the cell by breaking up cytoskeletal proteins and repair enzymes (19). Recently, inhibitors of apoptosis (IAPs) have gained attention as because of their impressive ability to inhibit the final caspase cascade. All IAPs contain at least one Baculovirus IAP Repeat (BIR) domain that allows direct binding to and inhibition of caspases (20). XIAP is the most potent IAP and it inhibits apoptosis by directly blocking the activity of caspases 3, 7, and 9 via its three N-terminal BIR domains (21–23). Renwick et al., 2006 reported that XIAP over-expression protected the retina from infarction due to transient ischemia induced by increasing intraocular pressure (24). Their results indicate that, in the presence of XIAP, retinal function was maintained up to 4 weeks postischemia. They showed further that retinal structure, assessed by counting cells in the INL, measuring inner retinal thickness and counting axons in optic nerve cross-sections, was significantly maintained in the presence of XIAP.
EPIRETINAL MEMBRANE AND MACULAR HOLE
Proliferative vitreoretinopathy (PVR) is an acquired, scarring process in the posterior segment of the eye that commonly occurs after retinal detachment and penetrating trauma (25). Although the pathogenesis of PVR is not completely understood, it is characterized by the growth of hypocellular membranes on the retina and within the vitreous gel. Proliferation of several cell types, including RPE cells, fibroblasts, and glia, is accompanied by deposition of extracellular matrix proteins; this is followed by cell-mediated contraction of the collagen fibrils leading to tractional retinal folds and detachment (26). Somatic therapies directed at inhibiting cellular proliferation and the deposition of extracellular matrix have been efficacious in reducing the severity of disease in experimental animal models of PVR.
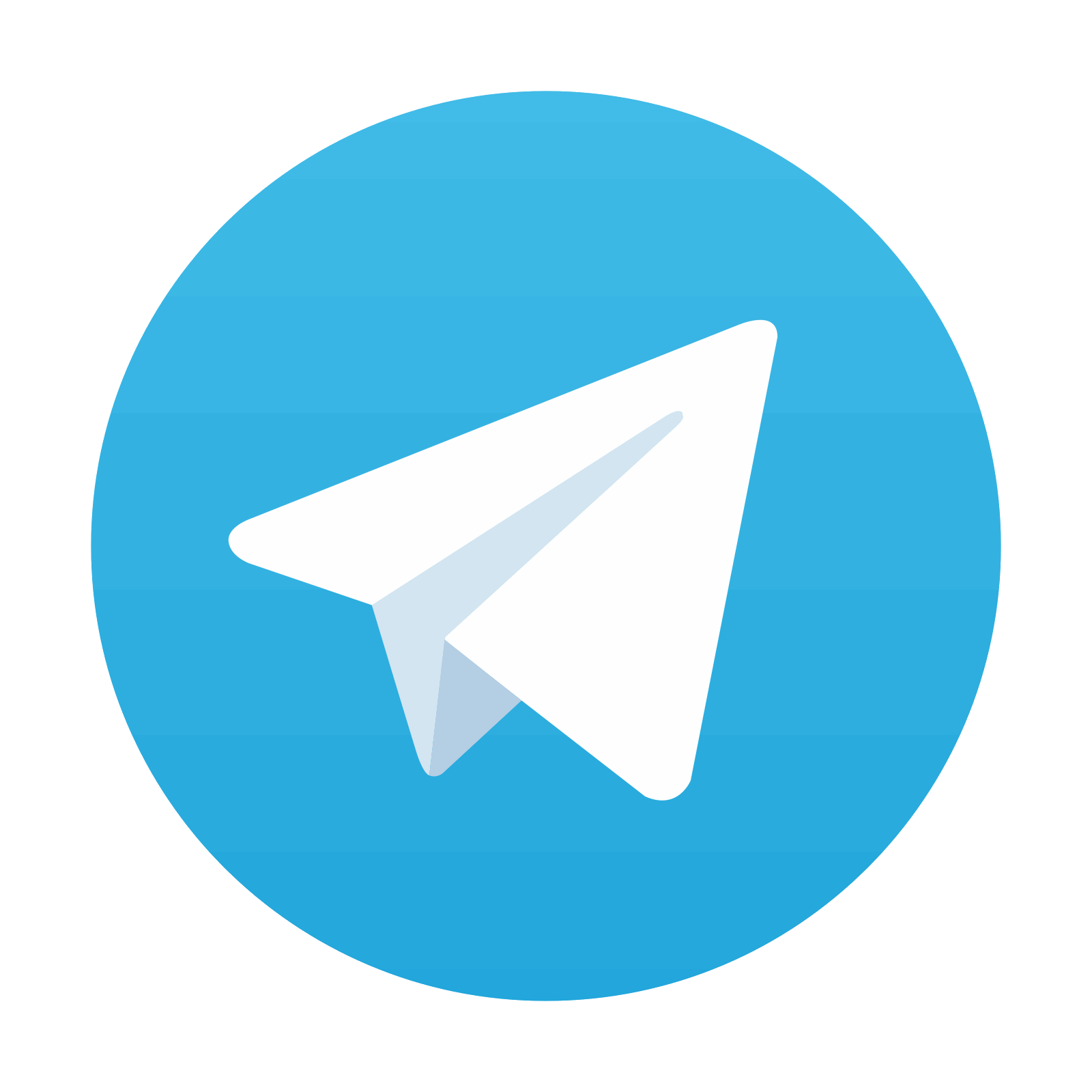
Stay updated, free articles. Join our Telegram channel

Full access? Get Clinical Tree
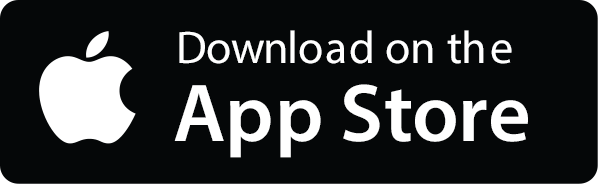
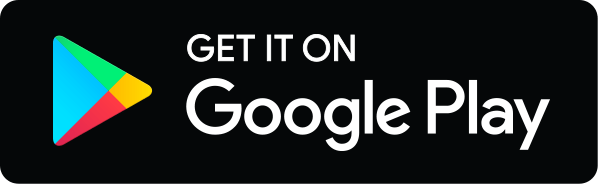