Purpose
To review the current state of molecular genetic testing as it relates to pediatric ophthalmology and to discuss its uses.
Design
Review and evaluation of available molecular genetic testing.
Methods
Literature review and discussion of testing in practice based on the authors’ clinical and laboratory experience.
Results
Fee-for-service testing for many genetic eye diseases now is available. A report is always generated for fee-for-service testing. Detection of DNA variants in genes known to cause eye disease must be interpreted taking into account the variability of the human genome, the presence of benign variants (polymorphisms), and the carrier frequency of recessive alleles. Negative results in genetic testing are helpful in some disorders for which most of the causative genes are known and many disease-causing variants have already been reported, but are less helpful in those that currently have many undiscovered causative genes or novel mutations. Research-based testing also is available, but does not always yield a result. Patients with RPE65 -associated Leber congenital amaurosis may be eligible for the current gene therapy trial. Patients with a variety of disorders may benefit from improved surveillance if their genetic diagnosis is known.
Conclusions
Entry into the genetic testing system often is via the patient’s ophthalmologist. Collaboration with geneticists and genetic counselors, use of web sites to keep up with the ever-changing availability and detection rates, and knowledge of clinical trials, when combined with excellent clinical diagnosis, can improve diagnosis and allow eligible patients to participate in treatment trials.
Types of Genetic Testing Available
Ever since the first genes for genetic eye diseases were discovered, DNA testing for diagnostic purposes has been available to some patients. In 1989, a method was reported for laboratory differentiation between germline and nonheritable retinoblastoma, and soon after, rhodopsin mutations were noted to be the cause of some cases of autosomal dominant retinitis pigmentosa. Seemingly overnight, a new era began in ophthalmology. A specialty that formerly was based largely on clinical diagnosis and often on subtleties of appearance developed into a field where retinopathies that appeared to be identical with the ophthalmoscope were found to be caused by different mutations in the same gene, or even by mutations in different genes altogether. In addition, some ocular disorders that seemed to be completely different from each other clinically have been found to be caused by mutations in the same gene.
Although a clinical diagnosis would have sufficed for most patients a generation ago, the explosion of molecular discoveries during the past 2 decades has fueled many patients’ desires for a rapid and accurate molecular diagnosis of their disease. As a result, clinicians will need to acquire a new level of understanding about how molecular biology is used to understand and ultimately to impact the pathophysiologic features of these diseases. Like much of ophthalmology, this is a constantly changing landscape. This review will discuss why genetic testing is important, and how active clinicians can incorporate it into their practice in a practical and effective manner.
In the past, many dedicated ophthalmologists sent their patients’ blood samples to research laboratories only to find that they rarely received any results from these investigations. Although some patients did receive a genetic diagnosis, the physician often was required to field many requests for updates on the status of their testing with the answer, “I don’t know.” Patients and their doctors had an unspoken fee-for-service expectation of performance from the research labs, whereas in fact the research labs had insufficient personnel, instrumentation, communication strategies, or a combination thereof to deliver test results with this degree of customer service. Fortunately, clinicians and patients are gaining a greater appreciation for the complexity of genetic testing at the same time that research and commercial laboratories are gaining a greater understanding of their collaborators’ and clients’ needs. Thus, the expectation gap is closing for many genetic tests for eye diseases.
All fee-for-service testing, and some research testing, is performed using validated laboratory procedures specified by the Clinical Laboratory Improvement Act (CLIA). Currently available fee-for-service testing for inherited eye disease is very different from the research testing clinicians may have experienced in the past. First, there is a fee for the testing that is payable either by the patient or by their insurance carrier. More importantly, there is a written report, of either positive or negative results, that is generated and sent to the referring physician or patient, or both, in every case. Because many different genes can cause some inherited eye diseases, a report of negative results usually is not nearly as helpful in patient management as one reporting positive results. In addition, because genes are still actively being found and certain alleles are observed only in certain populations, even positive results require more validation and qualification than a more routine laboratory test like a white blood cell count or liver enzymes analysis. This will be discussed more fully below. Here, it is sufficient to emphasize the point that for a test to be offered in a fee-for-service manner, that test must be validated and performed in a laboratory that is certified to meet CLIA standards.
Today when a clinician suspects a genetic eye disease based on examination and testing in the office, an excellent first resource is the web site www.genetests.org , managed by Roberta Pagon, MD, at the University of Washington, Seattle. This site is user friendly and allows the physician to query for testing for various diseases. There are links to laboratories at which fee-for-service testing for each disorder is performed, as well as detailed information on how to send a sample. The site states what type of testing is offered and whether carrier and prenatal testing is offered at each laboratory. Labs offering fee-for-service testing must be CLIA certified. Information on laboratories that offer research-based testing also is available on this site.
For patients with insurance, printing the forms for the laboratory of choice, having the physician fill out the appropriate fields, and taking the forms to a phlebotomy lab, often are all that is required. However, many insurance carriers will not pay for testing without precertification. Patients, physician office staff, or both should call the carrier before having blood drawn to avoid surprise bills. In addition, as with all bills sent for payment to insurance carriers, each insurer has their own policies about which tests will be covered and which denied. In our experience, with appropriate attention to precertification, insurance companies will cover genetic testing for inherited eye disease approximately 80% of the time. However, it still behooves the physician to tell patients that each insurance plan is different, and that it is always safest to get a precertification number even before having the blood drawn. Because of the recent proliferation of fee for service genetic tests for all types of genetic disorders, many certified genetic counselors and genetic nurse specialists have become expert at getting precertification and coverage for genetic testing. For many clinicians, the most efficient way to obtain testing for a given patient is to refer them to a general genetics clinic with their suspected ocular diagnosis and paperwork for a laboratory offering testing in hand. This collaboration between ophthalmologist and geneticist or genetic counselor is ideal because the ophthalmologist can provide the clinical diagnosis, then the genetics clinic can organize DNA testing, interpret the results, and offer genetic counseling. In many academic medical centers, genetic eye disease clinics are being formed that include an ophthalmologist with a specialty in genetic eye disease, in collaboration with a geneticist or genetic counselor. These combination clinics can provide the best of both worlds in just 1 visit for the patient.
Fee-for-service laboratories generally send the results to the ordering physician so he or she can discuss those results with the patient. The ordering physician must have a plan in advance for who will discuss and interpret the results with the patient. Many ophthalmologists prefer to work with a geneticist who will meet with the patient after the results of testing are obtained, because the discussion may be very complex. Recurrence risk in offspring, risk to other family members, reproductive options, and how to interpret both positive and negative results are outside the scope of many ophthalmologists’ realm of practice. It is important to remember that essentially all genetic eye disease is heritable, but not all genetic eye disease is inherited. Spontaneous new mutations are not uncommon. In these patients, there is no family history, but the genetic mutations may be passed on to subsequent generations. Some diseases have protean manifestations that only a very careful family history or examination of additional family members can elicit.
What the ophthalmologist can and should do is identify patients with likely genetic eye disease and make a tentative clinical diagnosis to direct genetic testing. Other members of the team can perform the genetic test, can help explain it to the patient, and can help to counsel the patient and his or her family. Thus, a good working relationship between the patient, ophthalmologist, the laboratory, and the counselor is key to taking maximum advantage of the genetic tests that currently are available.
Why is Molecular Genetic Testing Important in Pediatric Ophthalmology?
Molecular genetic testing is important for children with ocular diseases for a number of reasons. First, it is critical for accurately diagnosing certain diseases. Although a number of ocular diseases in children can be diagnosed based solely on clinical findings, there are certain diseases that cannot be diagnosed with confidence without molecular genetics. For example, in children without a family history of optic atrophy, it is possible to diagnosis Kjer dominant optic atrophy definitely only using molecular genetics. For other diseases, molecular genetic testing can confirm the diagnosis in a situation in which the clinical findings are suggestive of one disease, but there is some overlap with other diseases. For example, a child may have findings suggestive of autosomal dominant familial exudative vitreoretinopathy caused by the frizzled-4 gene, but because of the overlap with other diseases such as persistent fetal vasculature and retinopathy of prematurity, molecular genetics may be necessary to establish the diagnosis definitively. Although positive test results may be diagnostic, negative test results are not helpful in this disorder because there are several different genes and different methods of inheritance; it is estimated that only approximately 20% of index cases of familial exudative vitreoretinopathy are caused by currently detectable frizzled-4 gene mutations, whereas another 20% are caused by mutations in LRP5 , making the genetic diagnosis rate approximately 40% overall. Furthermore, molecular genetic testing often can establish more accurately the risk of the disease occurring in future pregnancies as well as in the offspring of the child than can clinical diagnostics alone.
Obtaining a molecular genetics diagnosis also is important in terms of enrolling patients in clinical trials. Mutations in more than 14 different genes have been shown to cause Leber congenital amaurosis (LCA), and many of these affect retinal function in very different ways. It is not usually possible to distinguish between these different genetic subtypes on the basis of clinical findings alone. Approximately 8% of patients with LCA have mutations in the retinal pigment epithelium-specific 65-kDa protein gene ( RPE65 ). This gene encodes a protein that is required to isomerize all-trans-retinyl esters into 11-cis-retinal. Patients with the RPE65 genotype have been shown to have a modest improvement in retinal function after the subretinal injection of adeno-associated virus carrying wild-type RPE65 complementary DNA. Because there is a treatment available for at least 1 form of LCA, it seems incumbent on ophthalmologists to determine if a patient with LCA has RPE65 -associated disease. For patients whose LCA is not caused by mutations in RPE65 , accurately establishing the correct molecular cause may give them the opportunity to participate in new treatment trials as they become available for other genetic subtypes of the disease.
Molecular genetic testing also can be helpful in predicting future problems. If a child is known to be at risk for certain ocular or systemic diseases, the intervals for screening can be adjusted accordingly. For example, one of the authors recently was referred a 2-year-old child with the 6p deletion syndrome. Because the deletion involved the FOXC1 gene, which is one of the genes that has been shown to be associated with Axenfeld-Rieger anomaly, he was referred for an ocular examination. On examination, he was found to have posterior embryotoxon and subtle corectopia consistent with Axenfeld-Rieger anomaly. Although he did not having any findings suggestive of glaucoma at that time, given that glaucoma develops in as many as 50% of patients with Axenfeld-Rieger anomaly, he will be screened at more frequent intervals.
Molecular genetic testing also can be invaluable in assigning a risk to a patient with an ocular disease for developing certain systemic diseases. An example of this is sporadic aniridia. Autosomal dominant aniridia is caused by inactivation of 1 copy of the PAX6 gene at 11p13. Most patients with aniridia have an intragenic mutation, but as many as 18% of patients with sporadic aniridia have a deletion involving the Wilms tumor suppressor gene, WT1 , which is located near the PAX6 gene. Although Wilms tumor develops in only 1 in 10 000 typical children, the risk of Wilms tumor developing increases 67-fold in patients with sporadic aniridia. Like retinoblastoma and the RB1 tumor suppressor gene, Wilms tumor is believed to arise from a loss of function of both WT1 tumor suppressor genes (i.e., the so-called 2-hit hypothesis). Abnormalities in the WT1 gene can be identified by chromosome analysis of 11p13 using fluorescence in situ hybridization, and by strategic mutation analysis. Therefore, children with sporadic aniridia benefit from referral to a geneticist for DNA testing and should be screened on a regular basis for Wilms tumor if confirmed by genetic testing.
Another example of medical surveillance being guided by a specific genetic subtype is CEP290 -associated LCA. We now know that different mutations of the CEP290 gene can cause isolated LCA, Joubert syndrome, Senior Loken syndrome, or Meckel Gruber syndrome. Children diagnosed with CEP290 -associated LCA therefore should have a screening renal ultrasound and regular urinalysis, and brain magnetic resonance imaging should be considered at diagnosis.
Since the advent of genetic testing, it has become apparent that many diseases exhibit genetic heterogeneity. This means that a single phenotype (clinical presentation) can be caused by many different genes or different mutations in the same gene, or both. Conversely, there can be phenotypic heterogeneity. This means the same gene, and in some cases even the same mutation, can cause different clinical appearances in different people. Genetic heterogeneity initially was a surprise, because ophthalmic diseases had been divided and subdivided by the best means possible at the time: ophthalmoscopic or slit-lamp appearance. However, as our understanding of these diseases grows, it is clear that genetic heterogeneity makes sense. Different gene malfunctions easily can lead to the same final common pathway of photoreceptor cell dysfunction and death, to the characteristic pigmentary changes seen in retinal degeneration, or to the loss of corneal or crystalline lens cell clarity resulting from abnormal cells in these tissues. For example, mutations in the ABCA4 and the RDS genes can cause very similar fundus changes that most clinicians would call retinitis pigmentosa. Yet other mutations in the ABCA4 and RDS genes cause only maculopathy, which clinicians would call Stargardt disease or pattern dystrophy.
Bardet Biedl syndrome is a multisystem disorder characterized by the unusual constellation of extra digits, obesity, renal disorders, reproductive disorders, developmental delays, and a progressive severe early onset retinal dystrophy. With such an unusual combination of features, one might think there would be only one gene in the human body that could cause this. Yet Bardet Biedl syndrome is now known to be caused by at least 12 genes. How can this be? A recent discovery shows that most of these genes contribute to a protein complex called the BBSome, which is important in cilia throughout the body. Now it makes perfect sense: if any one of the very different genes that contribute a piece to this BBSome complex is not working, the result is the same no matter which one of them it is: a cilia disorder. And many organs with cilia—such as brain, retina, gonads, kidney, olfactory receptors, hair cells of the ear—may be affected, linking the seemingly unrelated aspects of the disease. Of interest, CEP290 is also important in cilia.
In cases in which the same mutation causes different clinical presentations, it is likely that other modifying genes are involved that have not yet been discovered, or that there are environmental factors that play a role in how the gene is expressed. Two examples of this are the finding that variations in the Rpe65 gene modulate light damage susceptibility in mice and influence the progression of retinal degeneration in transgenic mice with retinitis pigmentosa, and that at least 2 loci on the X chromosome seem to influence how the 11778 mitochondrial mutation causes Leber hereditary optic neuropathy.
Why is Molecular Genetic Testing Important in Pediatric Ophthalmology?
Molecular genetic testing is important for children with ocular diseases for a number of reasons. First, it is critical for accurately diagnosing certain diseases. Although a number of ocular diseases in children can be diagnosed based solely on clinical findings, there are certain diseases that cannot be diagnosed with confidence without molecular genetics. For example, in children without a family history of optic atrophy, it is possible to diagnosis Kjer dominant optic atrophy definitely only using molecular genetics. For other diseases, molecular genetic testing can confirm the diagnosis in a situation in which the clinical findings are suggestive of one disease, but there is some overlap with other diseases. For example, a child may have findings suggestive of autosomal dominant familial exudative vitreoretinopathy caused by the frizzled-4 gene, but because of the overlap with other diseases such as persistent fetal vasculature and retinopathy of prematurity, molecular genetics may be necessary to establish the diagnosis definitively. Although positive test results may be diagnostic, negative test results are not helpful in this disorder because there are several different genes and different methods of inheritance; it is estimated that only approximately 20% of index cases of familial exudative vitreoretinopathy are caused by currently detectable frizzled-4 gene mutations, whereas another 20% are caused by mutations in LRP5 , making the genetic diagnosis rate approximately 40% overall. Furthermore, molecular genetic testing often can establish more accurately the risk of the disease occurring in future pregnancies as well as in the offspring of the child than can clinical diagnostics alone.
Obtaining a molecular genetics diagnosis also is important in terms of enrolling patients in clinical trials. Mutations in more than 14 different genes have been shown to cause Leber congenital amaurosis (LCA), and many of these affect retinal function in very different ways. It is not usually possible to distinguish between these different genetic subtypes on the basis of clinical findings alone. Approximately 8% of patients with LCA have mutations in the retinal pigment epithelium-specific 65-kDa protein gene ( RPE65 ). This gene encodes a protein that is required to isomerize all-trans-retinyl esters into 11-cis-retinal. Patients with the RPE65 genotype have been shown to have a modest improvement in retinal function after the subretinal injection of adeno-associated virus carrying wild-type RPE65 complementary DNA. Because there is a treatment available for at least 1 form of LCA, it seems incumbent on ophthalmologists to determine if a patient with LCA has RPE65 -associated disease. For patients whose LCA is not caused by mutations in RPE65 , accurately establishing the correct molecular cause may give them the opportunity to participate in new treatment trials as they become available for other genetic subtypes of the disease.
Molecular genetic testing also can be helpful in predicting future problems. If a child is known to be at risk for certain ocular or systemic diseases, the intervals for screening can be adjusted accordingly. For example, one of the authors recently was referred a 2-year-old child with the 6p deletion syndrome. Because the deletion involved the FOXC1 gene, which is one of the genes that has been shown to be associated with Axenfeld-Rieger anomaly, he was referred for an ocular examination. On examination, he was found to have posterior embryotoxon and subtle corectopia consistent with Axenfeld-Rieger anomaly. Although he did not having any findings suggestive of glaucoma at that time, given that glaucoma develops in as many as 50% of patients with Axenfeld-Rieger anomaly, he will be screened at more frequent intervals.
Molecular genetic testing also can be invaluable in assigning a risk to a patient with an ocular disease for developing certain systemic diseases. An example of this is sporadic aniridia. Autosomal dominant aniridia is caused by inactivation of 1 copy of the PAX6 gene at 11p13. Most patients with aniridia have an intragenic mutation, but as many as 18% of patients with sporadic aniridia have a deletion involving the Wilms tumor suppressor gene, WT1 , which is located near the PAX6 gene. Although Wilms tumor develops in only 1 in 10 000 typical children, the risk of Wilms tumor developing increases 67-fold in patients with sporadic aniridia. Like retinoblastoma and the RB1 tumor suppressor gene, Wilms tumor is believed to arise from a loss of function of both WT1 tumor suppressor genes (i.e., the so-called 2-hit hypothesis). Abnormalities in the WT1 gene can be identified by chromosome analysis of 11p13 using fluorescence in situ hybridization, and by strategic mutation analysis. Therefore, children with sporadic aniridia benefit from referral to a geneticist for DNA testing and should be screened on a regular basis for Wilms tumor if confirmed by genetic testing.
Another example of medical surveillance being guided by a specific genetic subtype is CEP290 -associated LCA. We now know that different mutations of the CEP290 gene can cause isolated LCA, Joubert syndrome, Senior Loken syndrome, or Meckel Gruber syndrome. Children diagnosed with CEP290 -associated LCA therefore should have a screening renal ultrasound and regular urinalysis, and brain magnetic resonance imaging should be considered at diagnosis.
Since the advent of genetic testing, it has become apparent that many diseases exhibit genetic heterogeneity. This means that a single phenotype (clinical presentation) can be caused by many different genes or different mutations in the same gene, or both. Conversely, there can be phenotypic heterogeneity. This means the same gene, and in some cases even the same mutation, can cause different clinical appearances in different people. Genetic heterogeneity initially was a surprise, because ophthalmic diseases had been divided and subdivided by the best means possible at the time: ophthalmoscopic or slit-lamp appearance. However, as our understanding of these diseases grows, it is clear that genetic heterogeneity makes sense. Different gene malfunctions easily can lead to the same final common pathway of photoreceptor cell dysfunction and death, to the characteristic pigmentary changes seen in retinal degeneration, or to the loss of corneal or crystalline lens cell clarity resulting from abnormal cells in these tissues. For example, mutations in the ABCA4 and the RDS genes can cause very similar fundus changes that most clinicians would call retinitis pigmentosa. Yet other mutations in the ABCA4 and RDS genes cause only maculopathy, which clinicians would call Stargardt disease or pattern dystrophy.
Bardet Biedl syndrome is a multisystem disorder characterized by the unusual constellation of extra digits, obesity, renal disorders, reproductive disorders, developmental delays, and a progressive severe early onset retinal dystrophy. With such an unusual combination of features, one might think there would be only one gene in the human body that could cause this. Yet Bardet Biedl syndrome is now known to be caused by at least 12 genes. How can this be? A recent discovery shows that most of these genes contribute to a protein complex called the BBSome, which is important in cilia throughout the body. Now it makes perfect sense: if any one of the very different genes that contribute a piece to this BBSome complex is not working, the result is the same no matter which one of them it is: a cilia disorder. And many organs with cilia—such as brain, retina, gonads, kidney, olfactory receptors, hair cells of the ear—may be affected, linking the seemingly unrelated aspects of the disease. Of interest, CEP290 is also important in cilia.
In cases in which the same mutation causes different clinical presentations, it is likely that other modifying genes are involved that have not yet been discovered, or that there are environmental factors that play a role in how the gene is expressed. Two examples of this are the finding that variations in the Rpe65 gene modulate light damage susceptibility in mice and influence the progression of retinal degeneration in transgenic mice with retinitis pigmentosa, and that at least 2 loci on the X chromosome seem to influence how the 11778 mitochondrial mutation causes Leber hereditary optic neuropathy.
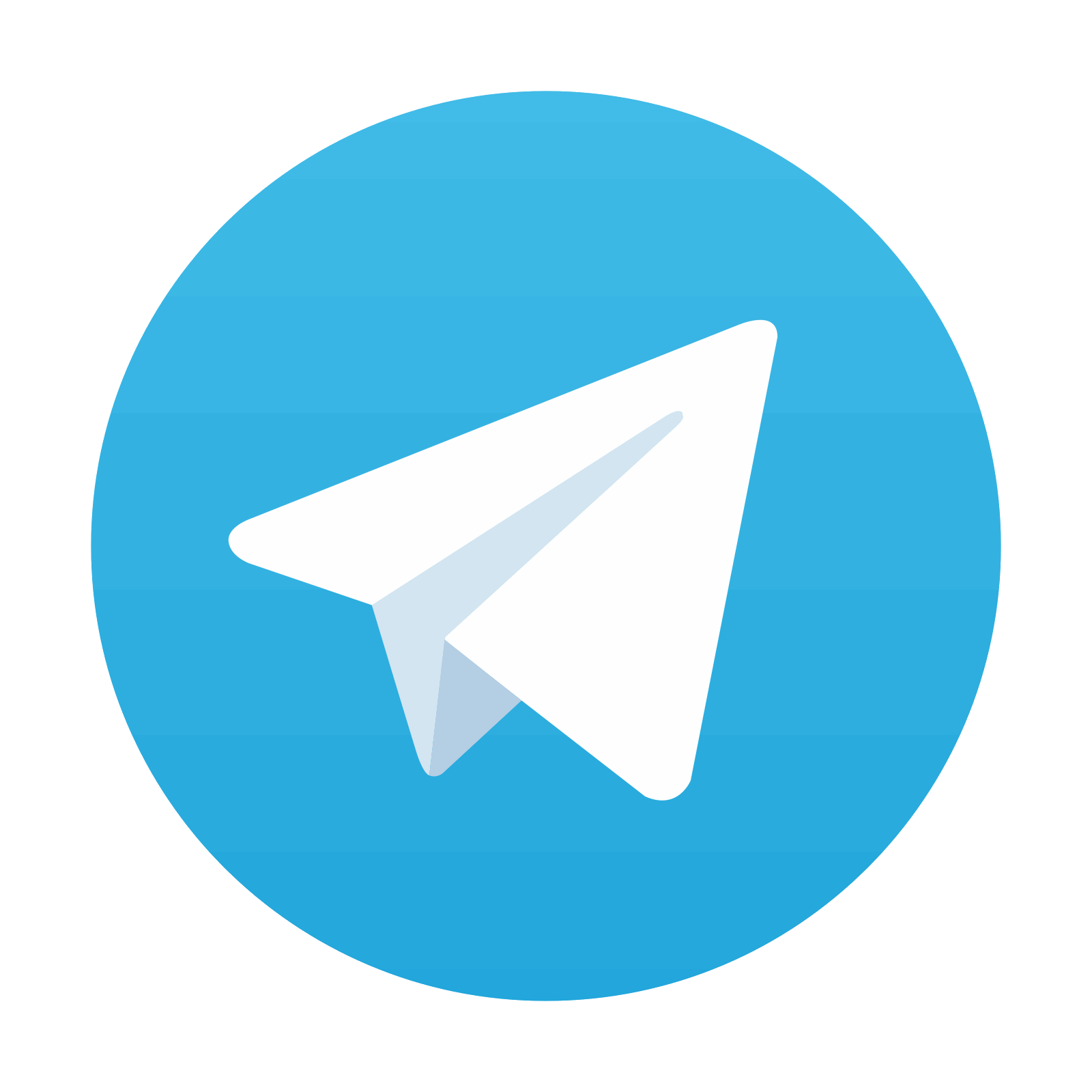
Stay updated, free articles. Join our Telegram channel

Full access? Get Clinical Tree
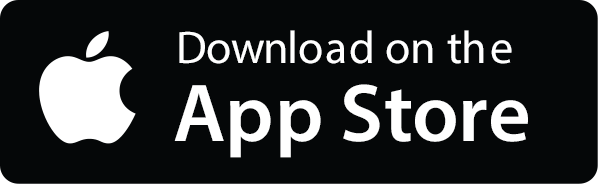
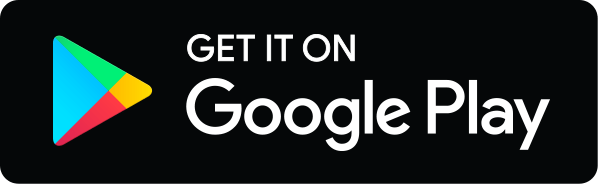