Fluorescein Angiography of the Ocular Fundus
Eleonora Lavaque
Virgil D. Alfaro III
John B. Kerrison
INTRODUCTION
Over the past thirty years, fluorescein angiography has been technically refined and continues to be a vital tool in the evaluation, diagnosis, and follow-up of patients with retinal and choroidal disease. The principles of fluorescein angiography were first described in 1960 by Angus McLean and Edward Maumenee. In its initial usage, fluorescein was given by intravenous injection followed by angioscopy at the slit lamp using a cobalt blue filter without photographic documentation (1). Later Novotny and Alvis presented the first human fluorescein angiogram, what they called “a method of photographing fluorescein circulating in blood vessels” (2).
Currently, two intravascular dyes are in use, sodium fluorescein and indocyanine green. They produce a different angiographic pattern because of their physical and chemical properties. By far, fluorescein angiography is more commonly used than indocyanine green angiography because it is inexpensive, easy to use, and diagnostically useful for a wide range of retinal diseases, many of them illustrated in this chapter. Indocyanine green angiography is selected for cases that require visualization of the choroidal vasculature or the imaging of structures blocked by hemorrhage, melanin, xanthophyll pigment, lipid exudates, retinal pigment epithelial detachment, or serosanguinous fluid (3).
PRINCIPLES
Luminescence is the emission of light in the visible spectrum from any source that has been stimulated by electromagnetic radiation. It occurs when energy in the form of electromagnetic radiation is absorbed at a shorter wavelength, shifted, and re-emitted at a longer visible wavelength. The process involves decay from a wavelength of higher energy to a wavelength at a lower energy state. Fluorescence is the luminescence maintained in response to continuous excitation. Therefore, fluorescence is present only if the excitation is present.
Retinal angiography is possible because of the chemical and physical properties of sodium fluorescein. It is a watersoluble, yellow-red hydrocarbon (C20H12O5Na) that is highly fluorescent, nontoxic, and inexpensive. It absorbs blue light energy between 465 and 490 nm and emits yellow-green light at a wavelength of 520 to 530 nm. In other words, if a blue light is projected as an exciting light from a camera into the eye, the sodium fluorescein circulating inside the eye will absorb and discharge a green-yellow re-emission or responder light that will come towards the camera. Eighty percent of intravenously injected sodium fluorescein is bound by serum proteins while 20% is unbound or free. Only the 20% of sodium fluorescein not bound to protein will fluoresce.
Two filters are placed in a camera for angiography: a blue filter and a green-yellow filter. The projector filter is a blue filter that allows only the blue wavelengths of the color spectrum to pass and reach the eye. The receptor filter is a green-yellow filter that allows only re-emitted green-yellow wavelengths to pass back towards the camera without residual blue light reflected. A mismatch of these two filters allows part of the reflected blue light from the fundus to come back through the system. This will make nonfluorescent objects appear to fluoresce. In other words, pseudofluorescence occurs when the two filters are overlapped. For example if a blue filter overlaps the green one, both blue and green light will pass. This phenomenon creates a decrease in contrast, increased background illumination, and loss of photographic detail (4).
Autofluorescence is the emission of fluorescent light from the ocular fundus in the absence of sodium fluorescein. Conditions that cause autofluorescence are optic nerve drusen and astrocytic hamartomata. More recently, other autofluorescent substances in the ocular fundus have been recognized using imaging techniques that give more information than obtained by conventional methods, such as fundus photography and fluorescein angiography (5). These techniques have permitted topographic mapping of lipofuscin distribution in the retinal pigment epithelium (RPE) as well as of other fluorophores occurring in the outer retina and subretinal space. Excessive accumulation of lipofuscin granules in the lysosomal compartment of RPE cells represents a common downstream pathogenetic pathway in various hereditary and complex retinal diseases, including age-related macular degeneration (5).
EQUIPMENT
Immediately after the injection of dye, photographs are taken. Cameras must be able to take rapid sequence images to ensure a high quality angiogram. Photographs may be film based or digital based. Digital fluorescein angiography stores information in an electronic record that allows immediate study of the angiogram and modification of illumination. This allows evaluation and adjustment of the quality of a study while it is still in progress. However, good stereoscopic images are best obtained in film-based photographs.
DYE ADMINISTRATION
Before the angiogram, the patient should be informed about the nature and side effects of the study. After signing a consent form, the patient is seated in front of the camera with one forearm extended. Color and red-free photographs are taken. An antecubital, forearm, or hand vein is cannulated. Prior to injecting a bolus of fluorescein, it is important to administer a small test dose injection in order to ensure that the dye is properly flowing into the vein. Ensuring proper flow will allow one to avoid painful extravasation with bolus injection that may occur if the intravenous cannulation site is infiltrated. Then, 5 to 10 milliliters of fluorescein dye is injected intravenously. The injection is given rapidly in order to ensure quality images.
There are few immediate side effects associated with fluorescein injection, particularly nausea and vomiting. Later effects include temporary tan or yellow skin color, fluorescent urine, and photosensitivity for 24 hours. Severe reactions are rare and include hives, asthmatic symptoms, and laryngeal edema. These can usually be managed by intravenous administration of
cortisone. Syncope, anaphylactic reaction, myocardial infarction, and respiratory or cardiac arrest are very rare events.
cortisone. Syncope, anaphylactic reaction, myocardial infarction, and respiratory or cardiac arrest are very rare events.
Fluorescein is a safe drug. It is advisable to have equipment and plans necessary to manage serious reactions. Such reactions typically appear in the first minutes following the intravenous injection, although it takes 24 hours for the liver and the kidney to eliminate fluorescein completely. Fluorescein angiography will interfere with laboratory testing that utilizes fluorescent-based methods.
ANATOMIC AND PHYSIOLOGIC CONSIDERATIONS
In comparison with ophthalmoscopy or color photography, blood vessels appear larger with fluorescein angiography. With angiography it is possible to see the entire caliber of the vessel, as compared to ophthalmoscopy or color photography, where it is only possible to see the central blood column and not the outer clear plasma layer.
The pattern of fluorescence is influenced by the size of the sodium fluorescein molecules and anatomic considerations. Sodium fluorescein does not cross intact retinal endothelial tight junctions (the inner blood-retinal barrier) or zonula occludens of the RPE (the outer blood-retinal barrier). However, it leaks freely through the porelike fenestrations of the choriocapillaris.
The macula is an oval area measuring 3.5 disc diameters between the vascular arcades and appears dark on fluorescein angiography. It is characterized by a high concentration of cone photoreceptors, the thickest ganglion cell layer, and the tallest RPE cells with high concentrations of lipofuscin and melanin. These characteristics block fluorescence emanating from the choriocapillaris beneath the macula, giving its dark appearance on fluorescein angiography.
The fovea, the center of the macula, is even darker due to the absence of retinal vessels. The fovea contains xanthophyll pigment, a progressive thinning of the inner retinal layers, and no retinal vessels. The foveal avascular zone, referred to as the FAZ, is approximately 400 to 500 um in diameter and is the darkest area at the center of the macula on an angiogram.
In a normal angiogram, fluorescein leaks from the porelike fenestrated choriocapillaris and is absorbed by the sclera. If there is chorioretinal atrophy, the fluorescence of the sclera may be observed. Bruch’s membrane allows the transmission of fluorescent light from the dye in the choriocapillaris as well as passage of the dye itself. As the dye continues to diffuse toward the retina from the choriocapillaris, the close apposition of the RPE cells prevents the dye from diffusing further into the subretinal space and retina.
In a normal angiogram, the optic disc fluoresces primarily due to fluorescein that has diffused into it from the surrounding permeable choriocapillaris.
NORMAL FLUORESCEIN ANGIOGRAM
A normal angiogram must be well understood and interpreted in order to provide a basis for comparison to abnormal angiograms. An angiogram will be interpreted as abnormal in terms of the amount of fluorescence, whether increased or decreased, in a specific area.
Prior to injection, color and red-free photographs are taken (Fig. 5-1). They are useful for correlation with pathologic areas found in an angiogram. No fluorescence should be evident at this stage. If there is fluorescence prior to injection of fluorescein, it is due to suboptimal matching of the two filters, pseudofluorescence, or less commonly a pathologic structure that is autofluorescent, such as optic nerve drusen. The most common autofluorescent structures of the fundus are flecks from fundus flavimaculatus, Best vitelliform lesions, and optic nerve drusen (Fig. 5-2) (6).
A normal angiogram has classically been divided into progressive phases: prearterial, arterial, arteriovenous, venous, and recirculation. The prearterial phase is the first phase, where fluorescein fills the choroid and choriocapillaris, occurring one
second before the retinal arterial circulation. It has a distinct pattern that is patchy and variable even in normal angiograms. The arterial phase progresses and ends when the arteries are completely filled (Fig. 5-3). The arteriovenous phase follows and is a transition phase with filling of the arteries and the veins (Fig. 5-4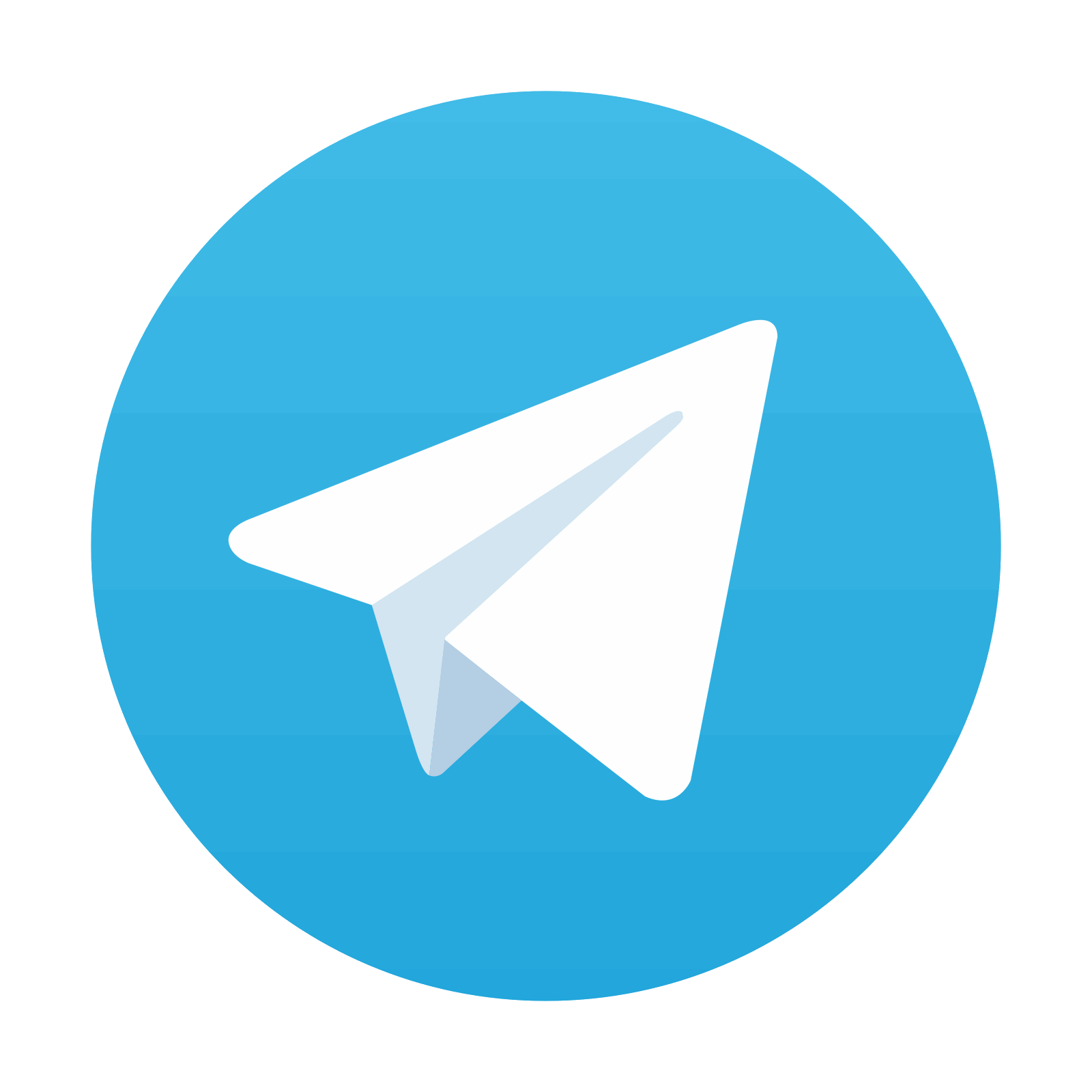
second before the retinal arterial circulation. It has a distinct pattern that is patchy and variable even in normal angiograms. The arterial phase progresses and ends when the arteries are completely filled (Fig. 5-3). The arteriovenous phase follows and is a transition phase with filling of the arteries and the veins (Fig. 5-4
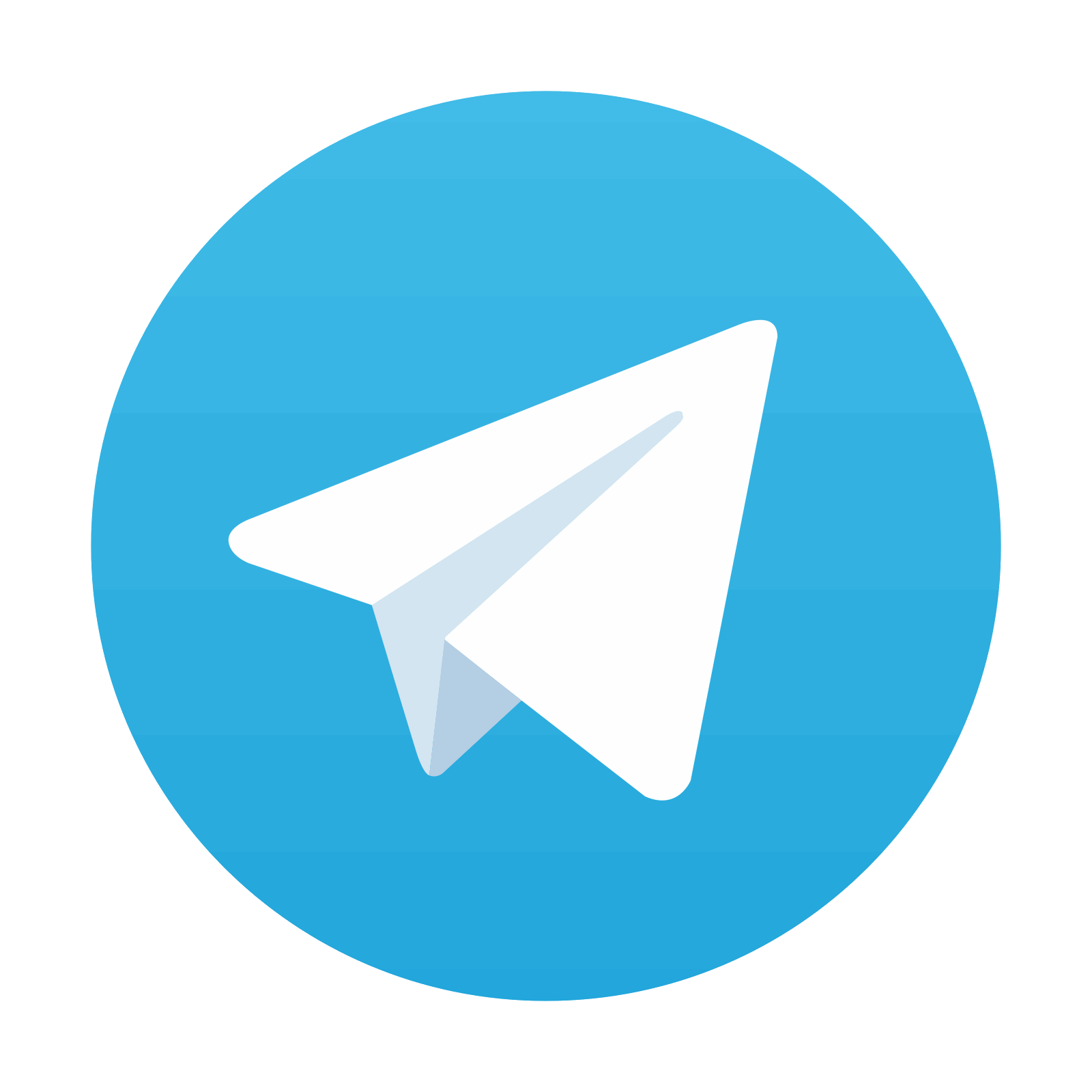
Stay updated, free articles. Join our Telegram channel

Full access? Get Clinical Tree
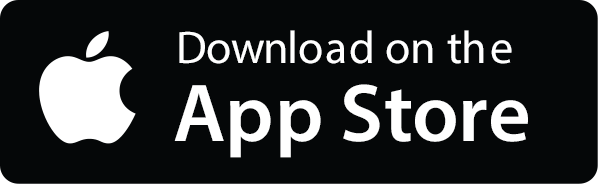
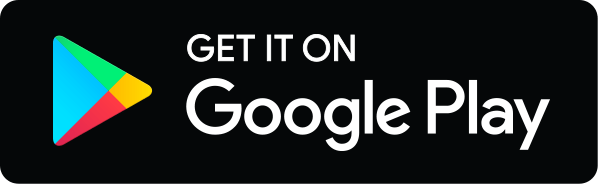