Fig. 1.1
Contour deviation and roughness are useful parameters for characterizing a cut surface. They measure deviations of the intended shape with very low and very high spatial frequency. Deviations with intermediate spatial frequency may be interpreted as “waviness”
The ablation behavior of excimer lasers is characterized, for example, by a particularly low surface roughness. Even state-of-the-art femtosecond laser keratomes now achieve a low roughness with low pulse energy and higher spot density (number of spots per surface area). For a lenticule with a diameter of 7 mm, for example, each of the cut surfaces is prepared at a spot distance of 3 μm by almost 4.3 million laser shots. One should compare this with around 100 shots from an excimer laser, which produce the same surface covering. If one imagines the “filling” of a surface with these shot patterns with very different densities, intuitively one knows that any deviations when working with an excimer laser will show up as waviness. Height errors in the created profile therefore change only slowly with the position (technical term: low spatial frequencies). The characteristic “contour deviation” is used to describe a limit case of the lowest spatial frequencies. A lack of contour accuracy causes in particular an error in the correction of sphere, cylinder, and spherical aberration. The granularity of the scan pattern causes in particular roughness and waviness. With excimer laser ablation, however, there are further possible sources of error. These include the characteristics of the ambient air and the tissue to be ablated. The iterative character of the ablation process of an excimer laser leads to the summation of these errors during the procedure, which, when compounded, therefore increases with the magnitude of the planned refractive correction. The influence of these sources of error accordingly grows with the depth of ablation and the size of the optical zone.
In the SMILE procedure, however, the magnitude of correction has no direct influence on absolute precision. Ultimately, therefore, it makes almost no difference whether the correction magnitude is 1 D or 10 D. At second glance, however, it becomes evident that this procedure can result, in the case of very minor corrections (<1 D), in an interaction between the two incisions, which are very close to each other in a minor correction (cap incision and lenticule incision, Fig. 1.3). If this is to be avoided, minimizing the gas bubbles and thus using as low a pulse energy as possible is beneficial in terms of the quality of the procedure. The comparison of the femtosecond laser keratomes available on the market concerning pulse energy thus also gives an initial indication of their fundamental suitability for lenticule preparation. A first attempt by IntraLase to perform a refractive correction by preparing a stromal tissue disk was reported, however, seemed not pursued any further by consecutive clinical trials [2].
1.1 Why Does the Pulse Energy Used by the Femtosecond Laser Keratomes Vary?
It is not possible to set the pulse energy for a particular femtosecond laser keratome as low as desired. The minimum pulse energy of each of these systems is determined by its ability to generate the necessary radiation intensity for plasma formation in the beam focus (pulse energy per focus area). One can also imagine this intensity as the photon density in the medium, which must be reached so that the tissue that is transparent under normal condition absorbs enough radiation (nonlinear) for all chemical bonds to be broken and plasma to form in the laser focus. The idea is therefore to concentrate as many photons as possible in as small a volume of space as possible at the same time. This is achieved by extreme focusing of ultrashort pulses. The focus volume decreases as the beam cone angle increases, the largest possible beam cone angle favors the achievement of plasma formation at the lowest possible pulse energy. In optical engineering, it is common to use the term “numerical aperture” instead of beam cone angle (angle of aperture) (Fig. 1.2).


Fig. 1.2
The VisuMax® femtosecond laser keratome. It utilizes full-field objective lenses to provide maximum cutting precision, efficiency, and comfort (Source: ZEISS, 2012 at WOC Abu Dhabi)
Today’s femtosecond laser keratomes can be divided into two categories, which are distinguished by the optical systems they use. The first is the class of devices with full-field objective lenses, which include, e.g., IntraLase and VisuMax. The second category includes the devices made by Ziemer, which work with partial-field objective lenses and which can only address a small section of the actual treatment field, without moving the objective. Whereas in the case of the really large full-field objective lenses a high numerical aperture (large beam cone angle) is quite costly, and, in addition, the human anatomy presents certain limitations (nose, cheekbone), a partial-field objective lens is comparatively small and cost-effective. One “gains” this cost advantage with the partial-field objective lens, but with less freedom when it comes to shaping the cutting surfaces. Partial-field objective lens systems therefore tend to be unsuitable for lenticule preparation. In addition to a suitable objective lens, an appropriate beam control is also required in order to also be able to achieve the dome-shaped incision curvature necessary for lenticule preparation with the required precision.
1.2 What Shapes Are the Incisions for a SMILE Lenticule and What Precision Is Required?
The shape of the incisions in lenticule preparation is based equally on the basic principles of the initial pioneering work of José I. Barraquer Moner in 1948 [3], the theoretical work of Munnerlyn et al. in 1988 [4], and early work of Swinger et al. and Lai [5, 6] on intrastromal cutting. It is also based on the work of ZEISS on the specific technical execution, which we have been developing in collaboration with our colleagues since 2002 [7–9]. The experiences with LASIK and PRK played a rather subordinate role in this, because the transferability of empirical knowledge concerning the specific shape of the ablation profiles in these two procedures is not really given for various reasons. Furthermore, there is the clinical methodology, which began with the pioneering work of Sekundo and Blum [10] and was then gradually improved. A great many outstanding refractive surgeons contributed to this, some of whom also appear in this book with their own contribution.
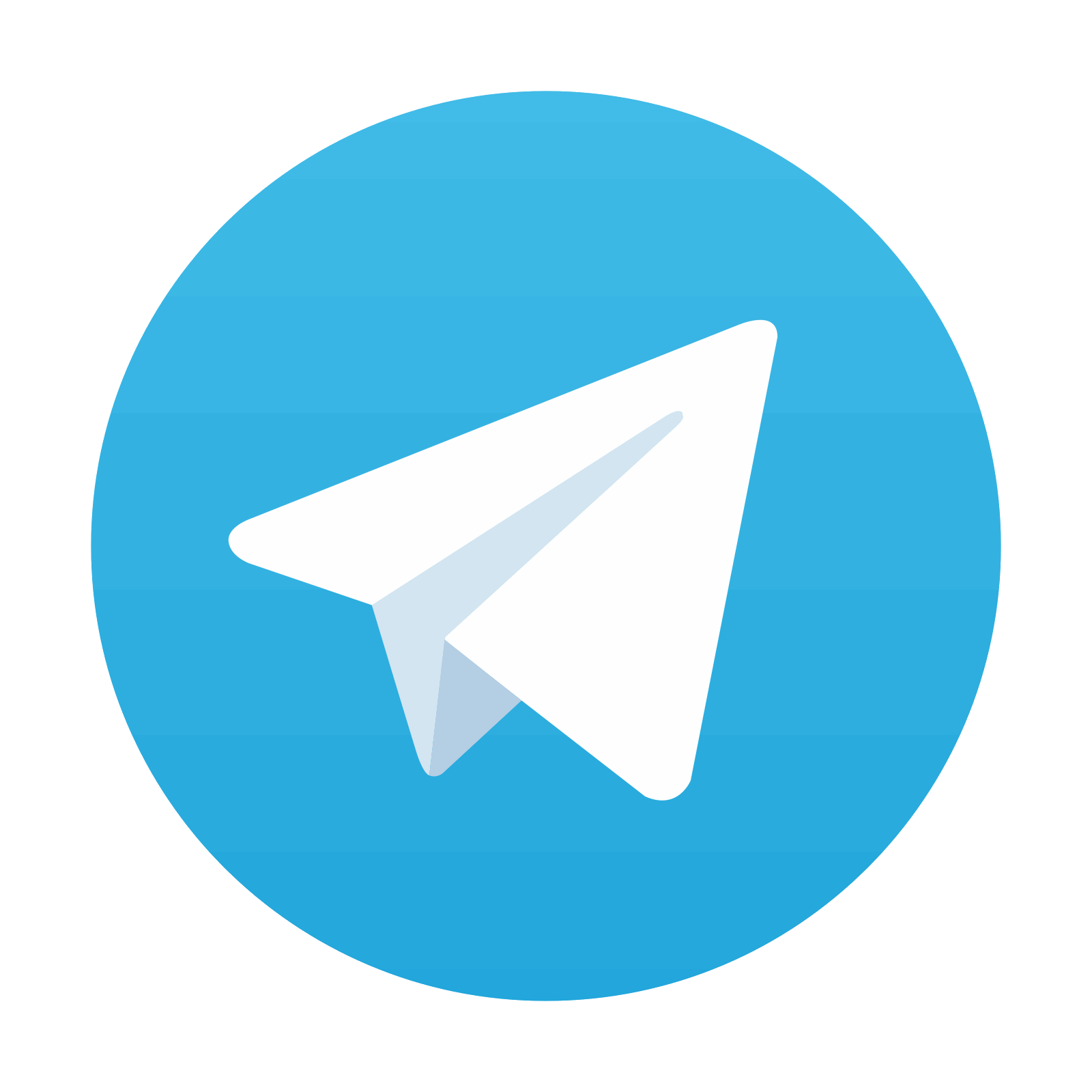
Stay updated, free articles. Join our Telegram channel

Full access? Get Clinical Tree
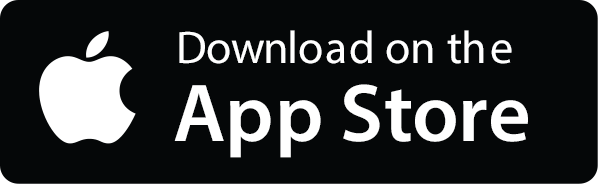
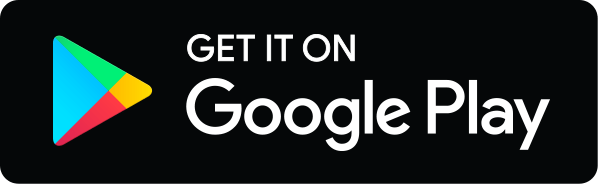