6 Femtosecond Laser–Assisted In Situ Keratomileusis III: Clinical Outcomes
Summary
Femtosecond laser–assisted in situ keratomileusis has been shown to be safe and effective in the treatment of refractive error. This technology offers a high level of precision and customization in flap creation, while facilitating fast visual recovery after surgery. Satisfactory outcomes with femtosecond platforms can be expected, although individual results depend on a variety of other factors, such as the level of refractive error and excimer platform.
Keywords: femtosecond, LASIK, outcomes, refractive error, ametropia, flap, safety, efficacy, thickness, hinge, side cut, excimer, wavefront guided, wavefront optimized, HOAs, visual acuity, contrast, night driving
6.1 Introduction
The application of femtosecond lasers for flap generation in laser-assisted in situ keratomileusis (LASIK) represents a pivotal technological evolution of the procedure. Femtosecond technology provides several important advantages in refractive surgery. These devices selectively produce tissue disruption at programmable focal points within the corneal stroma, which enables a high degree of precision in the generation of dissection planes required for flap creation (▶ Fig. 6.1 and ▶ Fig. 6.2). The precision offered by femtosecond technology allows for more control over flap architecture, allowing procedures to be tailored to meet the individual needs of the patient (▶ Fig. 6.3). Flap thickness, centration, diameter, hinge angle and width, and flap morphology are all customizable parameters, in contrast to microkeratome, with potential to influence visual outcomes. In addition, total procedure time with the newer devices matches or surpasses that of surgeons experienced with microkeratomes, making it a suitable option in higher volume centers. Overall, the most prominent advantages offered by femtosecond lasers are its safety and reproducibility. Femtosecond-assisted procedures consistently generate flaps of predictable, customizable architecture with safe and efficacious visual outcomes. Limitations are few but important: femtosecond LASIK generally requires more resources to establish, both in cost and office space, and intraoperative and postoperative complications can be unique to the platform, placing emphasis on provider experience to achieve optimal results.
(Image courtesy of SC Schallhorn.)
Fig. 6.1 Patient interface. The suction ring has been applied to the eye and docking with the femtosecond laser is about to occur (applanation of the cornea).
(Image courtesy of SC Schallhorn.)
Currently, the femtosecond platforms available in the market fall into one of two major categories based on relative differences in pulse energy and repetition rate: high-energy/low-frequency and low-energy/high-frequency platforms. The high-energy/low-frequency devices produce pulses in the microjoule (µJ) range, and fire at frequencies in the kilohertz (kHz) range. Conversely, the low-energy/high-frequency devices operate in the nanojoule (nJ) and megahertz (MHz) range for pulse energy and repetition rate, respectively. Many other distinctions exist between devices, including the method of corneal applanation, procedure time, and side-cut angle, among others. To a large extent, the advantages offered by femtosecond platforms are considered generalizable to the technology, with limited studies comparing outcomes between devices.
The latest information of devices currently available can be found in ▶ Table 6.1. These data reflect the most up to date specifications from the manufacturer.
Laser device | IntraLase iFS | Femto LDV Z6 | VisuMax | VICTUS | WaveLight FS200 |
Company | Abbott Laboratories Inc., Illinois | Ziemer Ophthalmic Systems AG, Switzerland | Carl Zeiss Meditec Inc., Germany | Bausch & Lomb Inc. MO, USA | Alcon Laboratories TX, USA |
Photo |
|
|
|
|
|
Laser type | Diode-pumped Nd:glass regenerative | Diode-pumped Ytterbium oscillator | Fiber optic amplifier | Diode-pumped solid state laser | Oscillator -amplifier |
Wavelength (nm) | 1,053 | 1,020–1,060 | 1,043 | 1,040 | 1,045 |
Repetition rate | 150 kHz | 20 MHz | 500 kHz | 160 kHz | 200 kHz |
Pulse energy (μJ) | 0.7–2.5 | 0.150 | 0.12–1.0 | 0.65–2.0 | 0.1–1.2 |
Visualization of surgery | Virtual | Visual, virtual | Visual, virtual | Virtual, OCT | Visual, virtual |
Cut pattern | Raster | Raster | Spiral | Spiral | Raster |
Flap diameter (mm) | 5–9.5 | 8.5, 9, 9.5, 10 | 7–9.6 | 6–9.5 | 5–10 |
Flap shape | Circular, elliptical | Circular, elliptical | Circular | Circular | Circular, elliptical |
Side-cut angle (degrees) | 30–150 | 30–150 | 45–135 | 60–120 | 30–150 |
Applanation surface | Planar | Planar | Curved | Curved | Modified planar |
Average firing time (9-mm flap) | 13 s | 15–30 s | 15 s | N/A | 20 s |
Approx. no. of procedures | 6,500,000 | 1,000,000 | N/A | N/A | N/A |
Additional surgical applications | AK, CC, EK, ICRS, LK, PKP, Pocket | AK, CC, ICRS, LK, PKP, Pocket | LK, PKP | AK, CC, FLACS | AK, ICRS, LK, PKP |
Abbreviations: AK, astigmatic keratotomy; CC, clear corneal incisions; EK, endothelial keratoplasty; FLACS, femtosecond laser–assisted cataract surgery; ICRS, intracorneal ring segments; LK, lamellar keratoplasty; PKP, penetrating keratoplasty. |
6.2 Flap Creation
6.2.1 Femtosecond and Microkeratome
The preponderance of peer-reviewed literature has demonstrated that femtosecond lasers create LASIK flaps which are at least as efficacious as, if not superior to, those created by mechanical microkeratome. A wide body of literature has been generated studying the properties of femtosecond flaps, many with direct comparison to flaps produced by mechanical means. These studies have focused on intended versus actual flap cut depth and morphology, flap integrity, and associated stroma changes, among other topics. The philosophy driving flap creation is to create a stromal bed which matches the ablation area, and in this capacity femtosecond lasers offer many advantages, including customizable flap diameter and elliptical flaps (▶ Fig. 6.3). But overall, the most profound advantage is reliable flap generation—a critical step to obtain good refractive outcomes. Results from studies on flap quality publish results that usually fall into one of two categories: those that state the flaps created by femtosecond laser are no different and those that detail specific advantages in precision offered by the technology. These results follow, to some extent, the refinement of femtosecond technology over time. Typically, studies on older generations of femtosecond platforms tend to observe equivalency with microkeratome, while studies of newer devices demonstrate select advantages of the lasers.
6.2.2 Flap Thickness
Accurate and reproducible flap production has been essential to the development and adaptation of femtosecond lasers in LASIK. High reliability in flap generation is necessary for the creation of thin LASIK flaps. One focal point of research into the technology is intended versus actual measured flap thickness. Various factors are known to influence flap thickness when created with a microkeratome, including blade quality, entry angle, cutting mechanism, and cutting rate—factors which are largely nonexistent in femtosecond devices.
Femtosecond lasers as a class will likely yield mean flap thickness closer to target than mechanical microkeratome. In a meta-analysis of studies comparing outcomes of femtosecond LASIK to mechanical microkeratome, Chen et al 1 reported that IntraLase (Abbott Medical Optics, Santa Ana, CA) flaps had significantly lower deviation from the target thickness than microkeratome. This finding extends to other devices beyond the IntraLase platform. In a randomized, prospective, fellow-eye study on 44 patients, Pajic et al 2 compared visual outcomes and flap thickness of the Femto LDV (Ziemer Ophthalmic Systems AG, Port, Switzerland) with microkeratome. The study found a mean deviation from intended flap thickness of 6.5 ±5.2 µm for the laser, versus 16.8 ±10.5 µm for the microkeratome. Precision in flap generation has been evaluated both in mean central flap thickness and peripheral flap thickness. Notably, femtosecond lasers will consistently produce flaps closer to target thickness when measured at multiple points across the cornea, whereas microkeratomes usually form flaps thicker at the periphery. The mean center-periphery disparity in intended thickness ranges from 7.1 to 11.9 µm for femtosecond devices to as high as 55.4 µm for microkeratomes, as reported in a study by Ahn et al 3 comparing measured flaps from three femtosecond platforms with microkeratome. As such, it is generally considered that femtosecond lasers produce flaps of planar configuration, while mechanical microkeratomes are notable for yielding meniscus-shaped flaps. The meniscus shape of flaps created by microkeratome may have an impact on myopic regression after LASIK.
Target flap thickness remains an important ongoing consideration in femtosecond adoption, given that multiple studies have demonstrated that femtosecond lasers may produce flaps of slightly different thickness than intended. Ahn et al 3 found that the IntraLase 60 kHz and VisuMax (Carl Zeiss Meditec, Jena, Germany) platform tended to create flaps slightly thicker than the targeted 110 µm in their study, though the flaps generated by these devices were predictably consistent and planar. In a randomized controlled trial of the fellow eyes of 21 patients comparing femtosecond to microkeratome, Patel et al 4 also demonstrated that the IntraLase 15-kHz platform generated flaps thicker than the goal 120 µm. Maintaining up-to-date systems with proper oversight and experience with an individual platform remains invaluable safeguards of flap quality.
6.2.3 Flap Variability
In association with flap thickness, the working range and intraflap variability for flaps created with femtosecond lasers are improved over microkeratome. The predictable working range offered by bladeless technology is important in maintaining sufficient residual stroma (>250 µm) to avoid postoperative complications, including corneal ectasia. 5 Talamo et al, 6 in a retrospective study on 99 eyes with the IntraLase FS laser and a target flap thickness of 110 µm, reported that the percentage of thick flaps ≥170 µm is low, likely in the range of 0 to 3.7%. By comparison, rates for mechanical microkeratomes were greater, up to 5.8 to 16.8% with the Moria M2 (Moria SA, Antony, France) microkeratome targeting a 130-µm flap. Across the literature available, standard deviations in mean flap thickness range from 4 to 18.4 µm for femtosecond flaps, a reflection of the improvement over the meniscus-shaped flaps produced by microkeratome. 7 To give an estimate of this accuracy, Sutton and Hodge 8 reported on the proportion of flaps found to be within 20 µm of target depth. In a subset of patients undergoing LASIK, they prospectively analyzed flap properties in 260 patients using either the 15- or 30-kHz IntraLase platform, and found that overall 87.3% of flaps created with femtosecond lasers were measured to be within 20 µm of desired thickness. This number improved to 98% when restricting analysis to only flaps made with the 30-kHz laser. These studies underscore how the femtosecond platform provides a higher degree of confidence in flap generation with bladeless techniques.
6.2.4 Flap Integrity
Beyond reliable and customizable generation of flaps for LASIK, an important contributor to good clinical outcomes with femtosecond surgery is the integrity of the flap and associated structural changes. The nature of the flap creation in LASIK significantly alters corneal biomechanics, and the flap usually retains only a small amount of the cornea’s previous tensile strength. Maintaining flap integrity and adhesion after LASIK is essential to avoid complications such as flap displacement. In a rabbit model studying corneal adhesion strength after flap creation, Kim et al 9 found that femtosecond flaps required significantly more force to disrupt in comparison to flaps created by microkeratome. The authors theorized that this finding may be the result of heightened stromal inflammation observed early after femtosecond surgery. There exists limited data on the cellular and tissue responses in corneal stroma after femtosecond flap creation. However, the evidence to date suggests that femtosecond laser ablation does not lead to increased myofibroblast or scarring responses when compared to microkeratome, and it may be associated with reduced keratocyte proliferation and inflammatory cell invasion. 10
6.2.5 Side-Cut Angle and Hinge
Outcomes after femtosecond surgery are also likely influenced by side-cut angle, a parameter customizable by many femtosecond platforms. With a steep or inverted side-cut angle of 90 degrees or more, the edge of the flap is tucked underneath the adjacent tissue, which may promote adhesion and reduce the incidence of flap displacement and epithelial ingrowth (▶ Fig. 6.4). This is in contrast to the shallow, fixed side-cut angles of microkeratomes and select femtosecond platforms. In a large retrospective study by Clare et al, 11 the risk of early flap displacement after LASIK seems to be higher with microkeratome than with femtosecond laser. Planar flap morphology, superior hinge, and greater initial stromal inflammation work in conjunction with steeper side-cut angle to contribute to improved flap adhesion after femtosecond LASIK. In a randomized controlled trial, Kung et al 12 compared 120 fellow eyes randomized to conventional 70-degree side-cut angle with the IntraLase 60 kHz platform versus a 130-degree inverted side-cut angle with the IntraLase 150-kHz platform. They found that eyes that received the inverted side-cut recovered corneal sensation more quickly after surgery, but that finding did not translate into improvement in dry eye symptoms.
Fig. 6.4 Side-cut angle is a parameter uniquely customizable by many femtosecond platforms. The side-cut angle can be inverted (>90 degrees), whereby the flap margin can be tucked under the adjacent tissue, promoting proper alignment and seating.
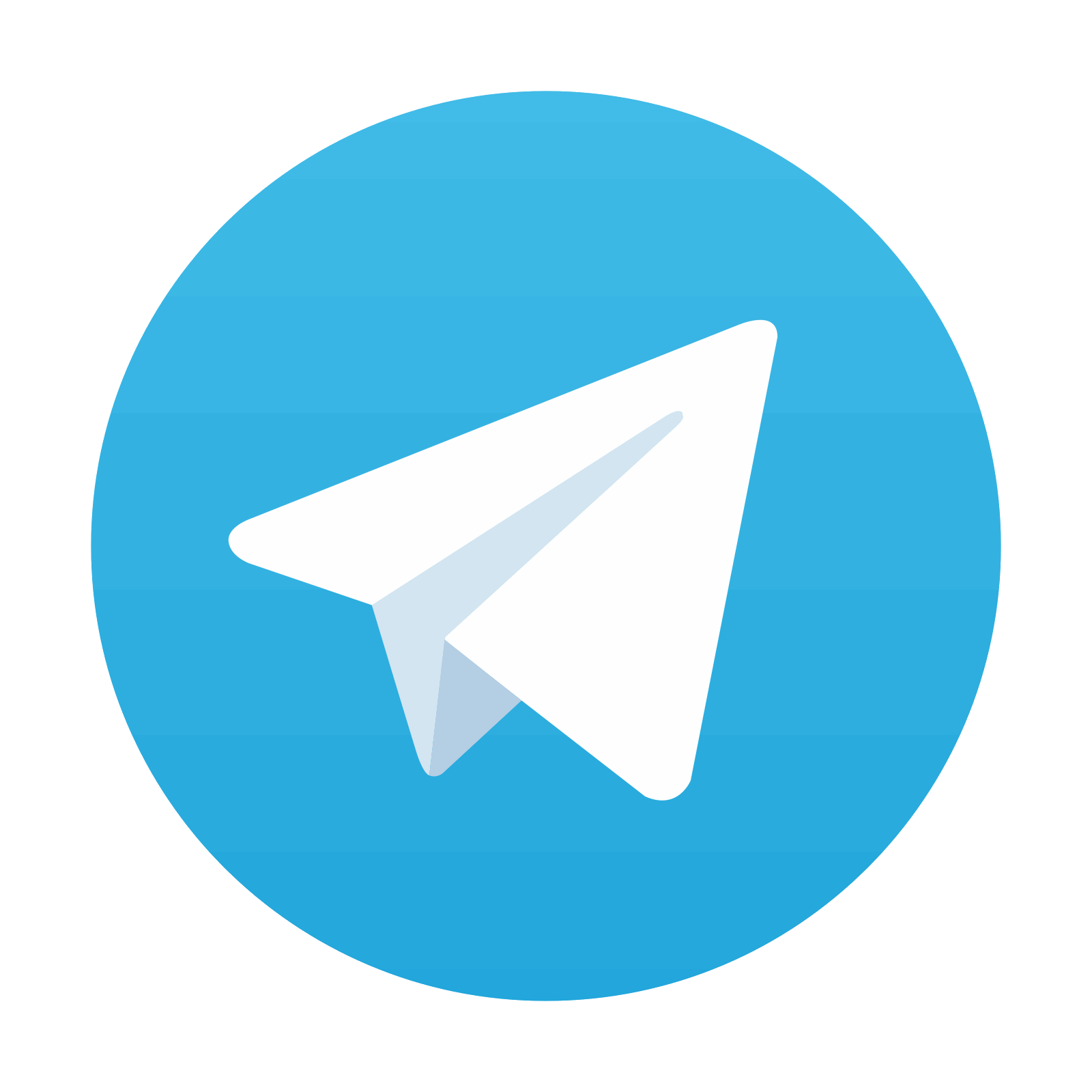
Stay updated, free articles. Join our Telegram channel

Full access? Get Clinical Tree
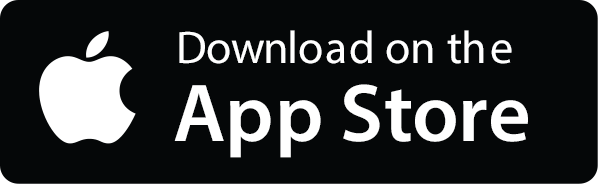
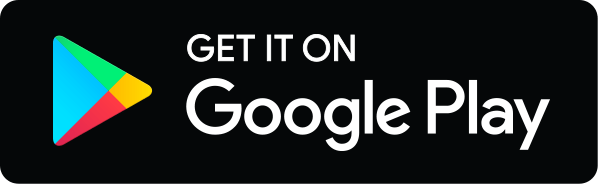