The traditional treatment for sialolithiasis was extirpation of the affected gland. It is now known, however, that salivary gland function can recover completely after stone extraction alone. Since the mid-1980s, much thought has been given to treating sialolithiasis with extracorporeal or intracorporeal shock waves in a manner similar to that used for urinary tract or biliary tract stones, and this has been implemented successfully. This article provides an overview of the various methods of extracorporeal and intracorporeal lithotripsy that have been used or proposed for salivary calculi to date, considering the advantages and disadvantages of each of the techniques.
Although less common than in the urinary or biliary tracts, calculus formation in the major salivary glands can also cause unpleasant symptoms with pain and swelling of the affected organ (salivary colic). Analysis of postmortem specimens led Rauch and Gorlin to the conclusion that some 1.15% of the population has stones in the salivary glands. Escudier and McGurk estimated that the incidence of symptomatic disease is 27 to 56 cases per 1 million inhabitants in the United Kingdom. Assuming that it is valid to extrapolate epidemiologic data, this figure related to the 300 million inhabitants of the United States means that 8100 to 16,800 patients are treated each year. The traditional treatment for sialolithiasis was extirpation of the affected gland. This was performed not just because of the symptoms but also for fear of recurrence and because of the residual loss of function of the affected salivary gland. Since van den Akker and Busemann-Sokole published their findings in 1983, however, it has been known that salivary gland function can recover completely after stone extraction alone. This provided one of the basic principles and prerequisites of the concept of minimally invasive surgery with conservation of function in the treatment of sialolithiasis. A second important aspect is the possibility of complications, such as nerve damage (facial, lingual, and hypoglossal nerves), cosmetically unacceptable scarring, and Frey syndrome, which may occur with complete resection of the gland.
Since the mid-1980s, much thought has been given to treating sialolithiasis with extracorporeal or intracorporeal shock waves in a manner similar to that used for urinary tract or biliary tract stones, and this has been implemented successfully.
This article provides an overview of the various methods of extracorporeal and intracorporeal lithotripsy that have been used or proposed for salivary calculi to date, considering the advantages and disadvantages of each of the techniques.
History
The word “lithotripsy” means “stone fragmentation” and was first proposed in 1813 by Gruithuisen, a Bavarian surgeon. He suggested that a urinary stone could be grasped in the bladder by an instrument introduced by way of the urethra, so that holes could be drilled in the stone to pulverize the stone. The underlying principle was that the small stone fragments produced would pass out of the body through the outflow tracts (ureter-urethra for urinary stones, the bile ducts for biliary calculi) without any adverse effects.
New instruments were developed over the next few years and the first successful lithotripsy was performed in 1824, in public, at the French Academy in Paris. The procedure avoided the need for surgical incisions and replaced lithotomy in five out of every six cases over the following decades. At the same time, the mortality associated with treating the condition fell from 15% to 7%.
Despite these early successes, the development of general anesthesia and the advent of surgical asepsis led to a return to surgical removal of the stones from the affected organs by the late nineteenth century. This could be difficult in the case of kidney stones, but the advent of cholecystectomy for gallstones in 1882 rendered these highly amenable to treatment.
The first reports of attempts to destroy gallstones and bladder calculi by noncontact sonic techniques came from Berlinicke and Schenetten 1951, Mulvaney 1953, and Coats in 1956. Using ultrasound with an intensity of about 5 W/cm 2 and exposure times of up to 30 minutes, slight degradation rates for the stones could be achieved. These attempts were stopped, however, because of serious tissue damage and lack of effectiveness.
The possibility of destroying stones without contact, from a certain distance, arose from advances made in high-speed physics. In 1975, urinary calculi placed in a beaker of water were completely disrupted using focused shock waves, without any direct contact with the appliance; 1980 saw the first successful fragmentation of renal calculi in humans, using shock waves from an external source focused within the body. This revolutionized the treatment concept for renal stones and the first reports of gallstone lithotripsy using a modified kidney lithotripter were published in 1983. At the end of 1985, Sauerbruch and colleagues successfully treated the first patients with gallstones by extracorporeal shock wave lithotripsy. Extracorporeal lithotripsy of biliary tract and pancreatic duct stones followed.
Parallel to the development of extracorporeal methods, intracorporeal techniques were also tested and established in various organ systems. These include intracorporeal electrohydraulic methods, various laser lithotripsy systems, and pneumatic lithotripsy. The development and diversification of the individual techniques in different organ systems is beyond the scope of this article but studies on the lithotripsy of salivary gland stones are mentioned in the relevant sections.
Extracorporeal lithotripsy
Definition and Mechanism of Action of Extracorporeal Shock Waves
Extracorporeal shock waves are compression waves propagated through a medium at supersonic speed. Although the behavior of shock waves is physically similar to that of ultrasound waves, there are great differences in physical characteristics and energy between the two. Although shock waves come from a single pressure pulse rising steeply to a peak and then decaying slowly, ultrasound waves are characterized by sinusoidal pressure variation, with successive compressive and tensile phases ( Fig. 1 ). When passing through biologic tissue, high-frequency waves lose energy to a much greater extent than low-frequency waves, so that shock waves with lower-frequency components are much less attenuated and penetrate to greater depths than ultrasound waves.
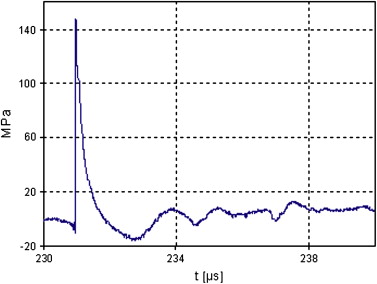
If when passing through biologic tissue a shock wave strikes a body of different impedance (eg, a stone), it is reflected as a compressive and tensile wave, depending on the acoustic properties of the interface. Mechanical damage occurs when the pressure exceeds the compressive or tensile strength of the material. Fragmentation of the stone can be explained as follows: an externally induced shock wave penetrates the body and is propagated uninterruptedly because there is basically no difference between the impedance of water and tissue. At the tissue-stone interface (ie, the anterior border of the stone) partial reflection of the shock wave causes compression forces to develop and act on the stone here. That part of the shock wave that travels through the stone is again reflected, this time from the posterior wall, creating a further compressive and tensile wave. The stone breaks up when the pressure exceeds the compressive or tensile strength of its material. The center of the stone is initially not affected, so it must be exposed to repeated shock waves. Every effort is made to focus the shock wave as narrowly as possible, to minimize the burden on the tissues as the waves pass through. In this way, maximum pressure amplitude is achieved in the focal area with only slight compressive stress distant from the focus. The shock waves have to be transmitted into the body from a medium with acoustic impedance similar to that of biologic tissue. Because biologic tissue is composed of about 70% water, water is used as the coupling medium. This prevents troublesome reflections and resultant stress or damage at the body surface. To be more specific, three different systems have been developed to date, and are now discussed ( Fig. 2 ).
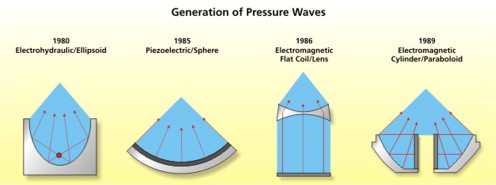
Generation of Extracorporeal Shockwaves
Electrohydraulic principle
The shock wave originates from the discharge of an underwater electrical spark. First, energy stored in a capacitor is released within a very short time. An arc forms between the electrodes; within about a microsecond, this explosively vaporizes the fluid surrounding the arc path, generating plasma-like conditions. The rapid vaporization and expansion generates a shock wave in the surrounding fluid, with spherical propagation of the wave. The shock wave is focused by generating it at the focal point of a reflector with rotational symmetry; it is reflected at the ellipsoid wall and the reflections produced convene at a second focal point (see Fig. 2 ).
Piezoelectric principle
The main component of a piezoelectric shock wave generator is a piezoelectric acoustic radiator consisting of a large number of ceramic elements arranged as a mosaic on the concave surface of a spherical recess. The structure is self-focusing. A high-frequency, high-voltage impulse from a pulse generator stimulates all of the individual ceramic elements simultaneously and causes their sudden expansion (piezoelectric principle). Water is used as the coupling medium to transmit the targeted shock wave. The hollow spherical structure allows a reflection-free, self-focusing system without aberrant acoustic wave components (see Fig. 2 ).
Electromagnetic principle
An electrical impulse from a generator is conducted to a flat coil. This induces changes in an adjacent metallic membrane and a shock wave of increasing intensity is propagated in a water container connected upstream. The shock wave is focused through an acoustic lens. A cylinder coil together with a paraboloid construction can also be used for focusing the shock waves (see Fig. 2 ).
Lithotripters Used and Basic Investigations
The first report of the in vitro use of an electrohydraulic lithotripter (Dornier MedTech Europe GmbH, Wessling, Germany) to treat a salivary gland calculus was in 1986. The study used a large sialolith (1.5 × 0.5 cm), which had been removed from the hilum of a submandibular gland. The stone was put in a plastic bag filled with water and immersed in the water-bath of a renal lithotripter. Under radiograph guidance, the stone was placed at the focus and a series of 50 shock waves applied. The stone fragmented. Nevertheless, the authors went on to say that the existing lithotripter would need to be modified to facilitate treatment in patients. They were aware of possible complications and wanted to ensure precise focusing and keeping the mandible out of the focus.
A further in vitro case report used an electromagnetic lithotripter (Siemens AG Company, Medical Solutions, Erlangen, Germany). The aim of the experiment was to determine whether it was possible to position a patient so as to allow fragmentation of a stone without damaging the surrounding structures. Although the eyes, brain, and larynx could be protected, it was not possible to protect the teeth. In vitro, a stone disintegrated very quickly (200 shocks at 16 kV). The application of shock waves to an extracted tooth resulted in the fracture of the amalgam filling after 200 impulses and significant damage to the tooth enamel. The authors concluded that a special device with a small focus volume would have to be created for lithotripsy of submandibular stones.
This led to in vitro and in vivo experiments with the piezoelectric lithotripter from Wolf (Knittlingen, Germany). In comparison with electrohydraulic and electromagnetic systems, this system has the highest focusing gain “G” because of the generator aperture ( Table 1 ). “G” is defined as the ratio of the maximum pressure at the focus to the maximum pressure at the source. With G less than 20, it is usually necessary to give the patient an anesthetic, because a relatively large part of the shock wave energy is released on the surface. The focus dimensions of the piezoelectric system are considerably smaller and the probability of expected collateral damage is less ( Table 2 ). Because of the prerequisites, appropriate in vitro and in vivo testing was performed before using salivary lithotripsy in humans to establish whether it was possible to use shock wave therapy for salivary gland stones. Of 100 salivary gland stones treated in vitro by piezoelectric shock waves, 80% disintegrated completely. Complete disintegration was defined as the maximum diameter of the fragments being less than 1.5 mm.
System | Gain of Focus (G) |
---|---|
Electrohydraulic (eg, Dornier HM 3) | 5 |
Electromagnetic (eg, Siemens Lithostar plus) | 19 |
Piezoelectric (eg, Wolf Piezolith 2300) | 250 |
System | Dimension of Focus | |
---|---|---|
Axial (in mm) | Lateral (in mm) | |
Electrohydraulic (eg, Dornier HM 3) | 90 | 15 |
Electromagnetic (eg, Siemens Lithostar plus) | 40 | 11 |
Piezoelectric (eg, Wolf Piezolith 2300) | 5 | 3 |
It was assumed that stone fragments of this magnitude would pass out of the body spontaneously through the normal outflow channels. Later anatomic investigations confirmed this hypothesis. Neither the mineral composition nor the size, weight, or volume of the stone had any effect on whether the stone could be fragmented. The greater the size of the stone, the greater the mean number of shock waves required to fragment the stone completely. With respect to the fragmentation rate and treatment time, about 3000 shock waves seemed to be worthwhile for later use in patients.
Further in vitro studies on human tissue and in vivo studies in 30 rabbits (shock waves applied to the parotid area) were intended to identify acute and chronic damage to the tissues. They showed that bleeding into the tissues was caused by the opening of only the smallest blood vessels, irrespective of the impulse intensity of the shock wave in the experiment ( Fig. 3 ). No permanent tissue damage was observed. Nevertheless, shock waves applied directly to the eye with conduction across the superior orbital fissure led to detectable clinical and morphologic changes.
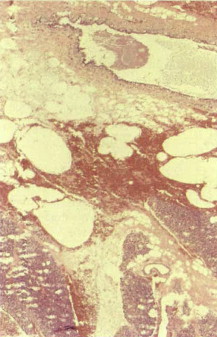
On the basis of these results, using shock wave systems with correspondingly small focus volumes and the physical properties mentioned previously, and assuming the precise positioning of the patient and reliable ultrasound (or alternatively radiograph) localization of the stone, serious adverse effects are not to be expected with salivary stone lithotripsy.
Based on these results, two systems proved to be suitable for clinical use: the Piezolith 2300 model and its successors, the Piezolith 2500 and 2501 ( Fig. 4 ) (Richard Wolf, Knittlingen, Germany); and the Modulith and Minilith electromagnetic systems ( Fig. 5 ) (Storz Medical, AG, Tägerwilen, Switzerland). Both systems have the corresponding protocols and presettings for the treatment of salivary calculi.
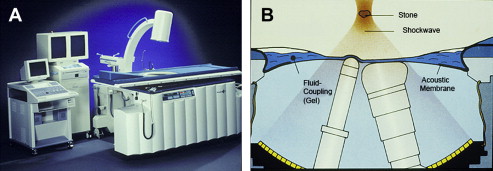
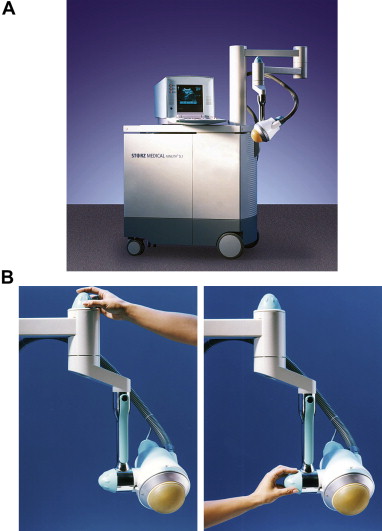
In the piezoelectric system, the water path is closed tightly with a latex membrane. This membrane can be adjusted by changing the pressure, so that during treatment the patient can be positioned comfortably on this device, which is like a soft cushion.
The shock wave generator can be moved in three planes to find the stone, so that the shock waves can be focused precisely on the sialolith under ultrasound guidance. The pulse frequency of the shock waves can be increased in three steps from 1 to 2.5 Hz; the maximum pressure development in the focus volume for salivary calculi lithotripsy is approximately 80 MPa.
The Minilith electromagnetic system consists of a miniature generator, which is fixed to a hinged bracket that can be moved though all spatial planes. This appliance has an integrated ultrasound localization system and a small water path. The system is mobile. The construction of the transducer with a rotary arm also allows lithotripsy to be performed with the patient in various positions. A comparative study of the two lithotripters found no significant differences between the in vitro effects or clinical results. Animal studies on the use of shock waves around the face, however, are available only for the piezoelectric system.
Patient Preparation
Although no dangerous adverse effects have occurred to date, patients should be informed of the following risks before they are given shock waves to the head and neck: bleeding; infection; abscess formation; subsequent removal of the gland; hearing loss or tinnitus; and potential damage to the teeth and injury to the eye (with incorrect application). Absolute contraindications are patients with cardiac pacemakers, the presence of coagulation disorders and acute infections of the gland.
Routine ultrasound B-scans are performed on the affected gland 1 hour before and 1 day after shock wave lithotripsy. In addition, all patients should have an audiogram performed before treatment and 24 hours afterward, to determine any possible iatrogenic hearing loss. Administration of oral analgesics before treatment is not usually necessary unless the patient has a very low pain threshold. Children under the age of 10 years are usually given a general anesthetic.
Before the stone is positioned in the focus, an ear plug is inserted into the external auditory meatus to protect the inner ear from injury caused by the acoustic shock when the shock wave is generated.
After setting the appliance under ultrasound guidance, shock waves are initially applied at the lowest intensity. Under continuous ultrasound monitoring, the intensity of the waves is increased and treatment continued until the maximum number of shock waves has been given (piezoelectric, 3000 pulses ; electromagnetic, 1300–7500 pulses). Treatment is stopped earlier if the stone has fragmented completely and can no longer be located with ultrasound, or if shock wave application is no longer safe because the patient is not cooperative.
If the shock waves fragment the salivary stone, it must be ensured that the fragments are flushed out through the natural outflow ducts of the gland. This can be encouraged by supportive measures performed by the patient or the treating physician.
Sialagogues and gland massage ensure a continuous flow of saliva. In addition, excretion of the stone can be facilitated by bouginage of the natural ostium, the narrowest part of the outflow tract. If individual fragments are palpable in the distal ducts near the ostium, or can be identified on ultrasound, these fragments can be extracted using a Dormia basket. Prophylactic oral antibiotics and anti-inflammatory drugs are given to all patients on the day of treatment and for 2 days afterward.
If symptoms persist or residual stones are identified on ultrasound scanning, a second treatment is given 2 months after the first treatment session, with a third session after 4 months if necessary.
Extracorporeal lithotripsy
Definition and Mechanism of Action of Extracorporeal Shock Waves
Extracorporeal shock waves are compression waves propagated through a medium at supersonic speed. Although the behavior of shock waves is physically similar to that of ultrasound waves, there are great differences in physical characteristics and energy between the two. Although shock waves come from a single pressure pulse rising steeply to a peak and then decaying slowly, ultrasound waves are characterized by sinusoidal pressure variation, with successive compressive and tensile phases ( Fig. 1 ). When passing through biologic tissue, high-frequency waves lose energy to a much greater extent than low-frequency waves, so that shock waves with lower-frequency components are much less attenuated and penetrate to greater depths than ultrasound waves.
If when passing through biologic tissue a shock wave strikes a body of different impedance (eg, a stone), it is reflected as a compressive and tensile wave, depending on the acoustic properties of the interface. Mechanical damage occurs when the pressure exceeds the compressive or tensile strength of the material. Fragmentation of the stone can be explained as follows: an externally induced shock wave penetrates the body and is propagated uninterruptedly because there is basically no difference between the impedance of water and tissue. At the tissue-stone interface (ie, the anterior border of the stone) partial reflection of the shock wave causes compression forces to develop and act on the stone here. That part of the shock wave that travels through the stone is again reflected, this time from the posterior wall, creating a further compressive and tensile wave. The stone breaks up when the pressure exceeds the compressive or tensile strength of its material. The center of the stone is initially not affected, so it must be exposed to repeated shock waves. Every effort is made to focus the shock wave as narrowly as possible, to minimize the burden on the tissues as the waves pass through. In this way, maximum pressure amplitude is achieved in the focal area with only slight compressive stress distant from the focus. The shock waves have to be transmitted into the body from a medium with acoustic impedance similar to that of biologic tissue. Because biologic tissue is composed of about 70% water, water is used as the coupling medium. This prevents troublesome reflections and resultant stress or damage at the body surface. To be more specific, three different systems have been developed to date, and are now discussed ( Fig. 2 ).
Generation of Extracorporeal Shockwaves
Electrohydraulic principle
The shock wave originates from the discharge of an underwater electrical spark. First, energy stored in a capacitor is released within a very short time. An arc forms between the electrodes; within about a microsecond, this explosively vaporizes the fluid surrounding the arc path, generating plasma-like conditions. The rapid vaporization and expansion generates a shock wave in the surrounding fluid, with spherical propagation of the wave. The shock wave is focused by generating it at the focal point of a reflector with rotational symmetry; it is reflected at the ellipsoid wall and the reflections produced convene at a second focal point (see Fig. 2 ).
Piezoelectric principle
The main component of a piezoelectric shock wave generator is a piezoelectric acoustic radiator consisting of a large number of ceramic elements arranged as a mosaic on the concave surface of a spherical recess. The structure is self-focusing. A high-frequency, high-voltage impulse from a pulse generator stimulates all of the individual ceramic elements simultaneously and causes their sudden expansion (piezoelectric principle). Water is used as the coupling medium to transmit the targeted shock wave. The hollow spherical structure allows a reflection-free, self-focusing system without aberrant acoustic wave components (see Fig. 2 ).
Electromagnetic principle
An electrical impulse from a generator is conducted to a flat coil. This induces changes in an adjacent metallic membrane and a shock wave of increasing intensity is propagated in a water container connected upstream. The shock wave is focused through an acoustic lens. A cylinder coil together with a paraboloid construction can also be used for focusing the shock waves (see Fig. 2 ).
Lithotripters Used and Basic Investigations
The first report of the in vitro use of an electrohydraulic lithotripter (Dornier MedTech Europe GmbH, Wessling, Germany) to treat a salivary gland calculus was in 1986. The study used a large sialolith (1.5 × 0.5 cm), which had been removed from the hilum of a submandibular gland. The stone was put in a plastic bag filled with water and immersed in the water-bath of a renal lithotripter. Under radiograph guidance, the stone was placed at the focus and a series of 50 shock waves applied. The stone fragmented. Nevertheless, the authors went on to say that the existing lithotripter would need to be modified to facilitate treatment in patients. They were aware of possible complications and wanted to ensure precise focusing and keeping the mandible out of the focus.
A further in vitro case report used an electromagnetic lithotripter (Siemens AG Company, Medical Solutions, Erlangen, Germany). The aim of the experiment was to determine whether it was possible to position a patient so as to allow fragmentation of a stone without damaging the surrounding structures. Although the eyes, brain, and larynx could be protected, it was not possible to protect the teeth. In vitro, a stone disintegrated very quickly (200 shocks at 16 kV). The application of shock waves to an extracted tooth resulted in the fracture of the amalgam filling after 200 impulses and significant damage to the tooth enamel. The authors concluded that a special device with a small focus volume would have to be created for lithotripsy of submandibular stones.
This led to in vitro and in vivo experiments with the piezoelectric lithotripter from Wolf (Knittlingen, Germany). In comparison with electrohydraulic and electromagnetic systems, this system has the highest focusing gain “G” because of the generator aperture ( Table 1 ). “G” is defined as the ratio of the maximum pressure at the focus to the maximum pressure at the source. With G less than 20, it is usually necessary to give the patient an anesthetic, because a relatively large part of the shock wave energy is released on the surface. The focus dimensions of the piezoelectric system are considerably smaller and the probability of expected collateral damage is less ( Table 2 ). Because of the prerequisites, appropriate in vitro and in vivo testing was performed before using salivary lithotripsy in humans to establish whether it was possible to use shock wave therapy for salivary gland stones. Of 100 salivary gland stones treated in vitro by piezoelectric shock waves, 80% disintegrated completely. Complete disintegration was defined as the maximum diameter of the fragments being less than 1.5 mm.
