Purpose
To construct a model simulating intraocular lens (IOL) opacification attributable to the formation of calcium phosphate deposits and to investigate the kinetics of deposit formation.
Design
Prospective laboratory investigation.
Methods
setting: Department of Ophthalmology, Medical School and Department of Chemical Engineering, Laboratory of Inorganic and Analytical Chemistry, University of Patras, Greece. study population: Three hydrophilic acrylic IOLs (26% water content) were placed inside a 10-mL double-walled thermostated reactor simulating the anterior chamber. Simulated aqueous humor was injected continuously into the reactor using a pump with variable speed. observation procedures: The observation of IOLs was carried out in situ daily by optical microscopy. Scanning electron microscopy and energy-dispersive radiographic spectroscopy were used for the identification of the morphologic features and the composition of the deposits.
Results
The lenses were removed and inspected 5, 9, and 12 months after the initiation of the experiment. Investigation showed deposits of calcium phosphate crystallites in the interior of opacified IOLs. However, these deposits were not observed on the surface of the IOLs.
Conclusions
In agreement with earlier reports by our group and in the literature, IOL opacification is the result of calcification. It is suggested that the surface hydroxyl groups of the polyacrylic polymeric components of the IOLs are capable of inducing surface nucleation and crystal growth of calcium phosphates. However, most important is the finding that the calcification of IOLs is initiated from their interior through the development of sufficiently high local supersaturation, realized through the diffusion of calcium and phosphate ions.
Small-incision cataract surgery with foldable intraocular lens (IOL) implantation resulted in a new postoperative complication: IOL opacification. Various factors implicated in the phenomenon have been suggested, including inflammation, irrigation solutions, viscosurgical devices, silicone, and fatty acids contamination.
Previous reports have demonstrated that IOL opacification is the result of calcification. The formation of calcific deposits consisting of calcium phosphate salts may be attributed to the fact that the aqueous humor is supersaturated with respect to different calcium phosphate crystalline phases. Modeling in vivo processes by reliable and reproducible in vitro methods is of key importance to understanding the underlying mechanisms. Precise thermodynamic calculations of equilibrium speciation in combination with kinetics measurements at conditions simulating the eye environment are expected to yield mechanistic information concerning the formation of calcium phosphate deposits.
We have developed an experimental model in an effort to investigate in vitro the mechanism of calcification of hydrophilic acrylic IOLs. The nature of the calcium phosphate phases formed in an aqueous medium simulating aqueous humor in relationship to the presence of foreign substrates provided by the IOLs was investigated.
Methods
An experimental model simulating the environment of IOLs into the eye was constructed to investigate the characterization and the kinetics of development of calcified deposits on hydrophilic IOLs.
A double-walled thermostated reactor was constructed, volume totaling 10 mL, made of polyamide. The reactor had glass windows on top and bottom to allow for the direct observation of the IOL specimens in situ using an optical microscope combined with an image analysis system (Quantimet 500; Leica Cambridge, Cambridge, United Kingdom). In the external wall of the reactor, water supplied from a thermostat was circulated to maintain the temperature at 37.0 ± 0.2 C, whereas in the interior of the reactor, constant flow of a simulated aqueous humor solution was ensured with the help of a syringe pump (Fresenius Kabi, Pilot C, Bad Homburg, Germany). The composition of simulated aqueous humor solution, which contained only inorganic components, is identical to that of electrolyte concentrations in normal aqueous humor, where the precise concentrations are as follows: sodium (Na + ) = 146 μmol/mL, potassium (K + ) = 5.25 μmol/mL, calcium (Ca ++ ) = 1.70 μmol/mL, magnesium (Mg ++ ) = 0.8 μmol/mL, chloride (Cl − ) = 109.5 μmol/mL, bicarbonate (HCO 3 − ) = 33.6 μmol/mL, and phosphate (H 2 PO 4 − ) = 0.62 μmol/mL.
The simulated aqueous humor solution was introduced in the reactor in an once-flow through mode at a flow rate of 0.2 mL/hour, simulating the in vivo flow in the anterior chamber, where aqueous humor is fully renewed within 2 hours. Three-piece hydrophilic acrylic IOLs (A, B, and C) in triplicate (SOFTEC III, LH-5000; LENSTEC Inc, St. Petersburg, Florida, USA), 12.75 mm in total length and 6 mm in optic diameter, made of hydroxyl-ethyl-methacrylate with 26% water content were placed in a special holder to maximize the available total surface exposed to the simulated aqueous humor. The observation of IOLs was carried out in situ daily by optical microscopy for the assessment of the opacification progress. A schematic diagram and a photograph of the experimental setup used for the in vitro calcification experiments of the IOLs are shown in Figure 1 .
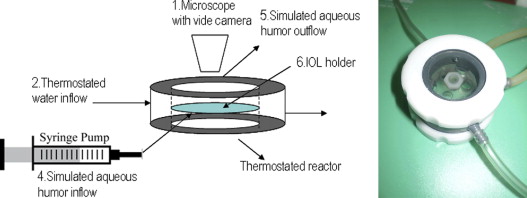
Five months after the initiation of the experiment, lens A was removed to be inspected, both at the surface and in the interior. The morphologic features of the deposits were examined using scanning electron microscopy (LEO SUPRA VP 35; Carl Zeiss, Oberkochen, Germany). The composition of the deposits was identified by microanalysis with energy-dispersive spectroscopy (JEOL 5200 with Oxford ISIS microanalysis unit; Oxford, United Kingdom). Lens B was removed on the ninth month, whereas lens C was inspected 1 year after the onset of the experiment. Similar studies, including scanning electron microscopy and energy-dispersive spectroscopy analysis, were used for the investigation of both of those lenses.
Results
Scanning electron microscopy analysis of the surface of lens A showed granular deposits with an appearance similar to that of calcium phosphate salts; despite this, energy-dispersive spectroscopy analysis of the deposit showed the presence only of calcium, which in combination with the carbon component, suggested either the presence of filler (CaCO 3 ) or the formation of calcium polymer salts ( Figure 2 ). The nature of these formations on the surface of the test specimens needs further investigation.
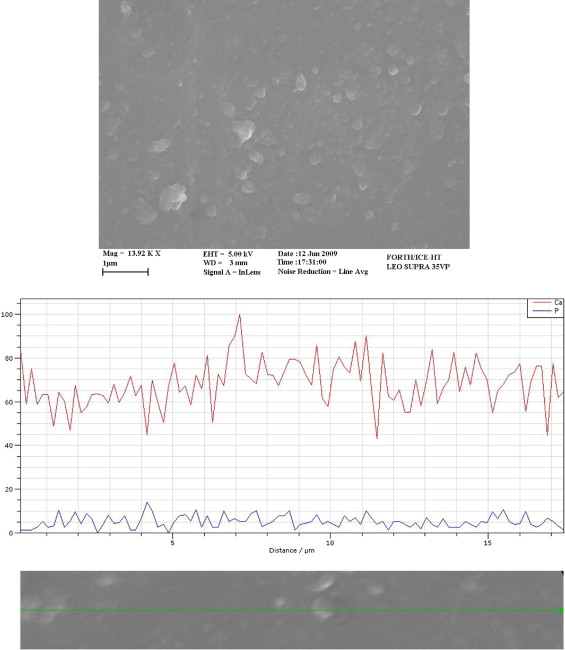
Subsequently, the IOL was cut for sagittal analysis. Scanning electron microscopy investigation showed the development of prismatic nanoparticle deposits in the interior of the IOL, approximately 10 μm from the surface. The deposits consisted of calcium phosphate crystallites. The size (< 100 nm) and the morphologic features of the observed mineral formation were typical of hydroxyapatite in the interior of the IOL. Energy-dispersive spectroscopy microanalysis confirmed the finding yielding a molar ratio of calcium-to-phospate equal to 1.67, corresponding to hydroxyapatite ( Figure 3 ).
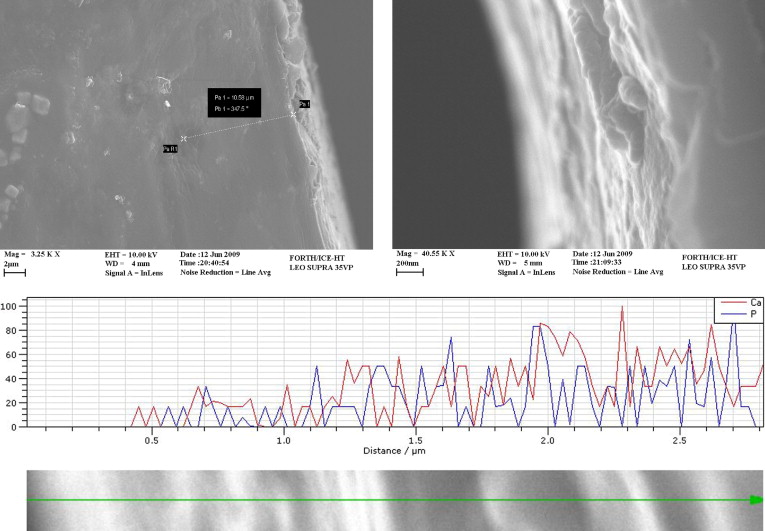
On the ninth month, lens B was removed and the same analytical methodology was applied ( Figure 4 ). The IOL’s surface was free of calcium phosphate deposits, as shown by energy-dispersive spectroscopy analysis, although the granular formations observed by scanning electron microscopy were found to a greater extent in comparison with the previous specimens. Formation of prismatic crystals of hydroxyapatite was found in sections of the lens, reaching depths of 233 μm from the surface ( Figure 5 ). Moreover, the number density of the crystalline deposits was larger in comparison with that of lens A. Lens C was inspected 1 year after the onset of the experiment. No mineral deposits were detected on the IOL’s surface, despite the fact that granular deposition was observed to extend over the entire surface ( Figure 6 ). Section analysis of the IOL by using both scanning electron microscopy and energy-dispersive spectroscopy showed deposits consisting of prismatic hydroxyapatite nanocrystals located at a depth of 249 μm from the surface. The distribution of the deposits was noted to be across a line parallel to the surface separated from it by a clear zone where no evidence of deposition was found ( Figure 7 ).
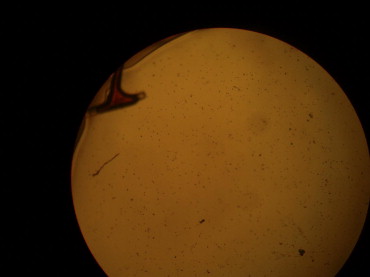
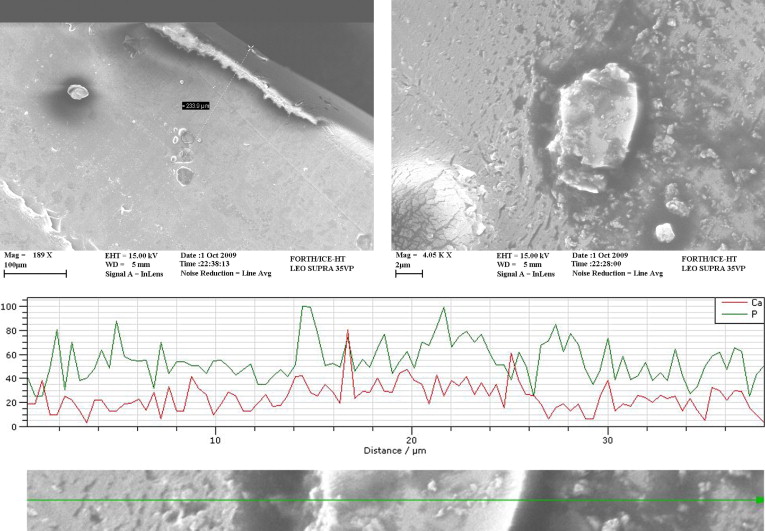
Results
Scanning electron microscopy analysis of the surface of lens A showed granular deposits with an appearance similar to that of calcium phosphate salts; despite this, energy-dispersive spectroscopy analysis of the deposit showed the presence only of calcium, which in combination with the carbon component, suggested either the presence of filler (CaCO 3 ) or the formation of calcium polymer salts ( Figure 2 ). The nature of these formations on the surface of the test specimens needs further investigation.
Subsequently, the IOL was cut for sagittal analysis. Scanning electron microscopy investigation showed the development of prismatic nanoparticle deposits in the interior of the IOL, approximately 10 μm from the surface. The deposits consisted of calcium phosphate crystallites. The size (< 100 nm) and the morphologic features of the observed mineral formation were typical of hydroxyapatite in the interior of the IOL. Energy-dispersive spectroscopy microanalysis confirmed the finding yielding a molar ratio of calcium-to-phospate equal to 1.67, corresponding to hydroxyapatite ( Figure 3 ).
On the ninth month, lens B was removed and the same analytical methodology was applied ( Figure 4 ). The IOL’s surface was free of calcium phosphate deposits, as shown by energy-dispersive spectroscopy analysis, although the granular formations observed by scanning electron microscopy were found to a greater extent in comparison with the previous specimens. Formation of prismatic crystals of hydroxyapatite was found in sections of the lens, reaching depths of 233 μm from the surface ( Figure 5 ). Moreover, the number density of the crystalline deposits was larger in comparison with that of lens A. Lens C was inspected 1 year after the onset of the experiment. No mineral deposits were detected on the IOL’s surface, despite the fact that granular deposition was observed to extend over the entire surface ( Figure 6 ). Section analysis of the IOL by using both scanning electron microscopy and energy-dispersive spectroscopy showed deposits consisting of prismatic hydroxyapatite nanocrystals located at a depth of 249 μm from the surface. The distribution of the deposits was noted to be across a line parallel to the surface separated from it by a clear zone where no evidence of deposition was found ( Figure 7 ).
