42 Experimental Approaches to Auditory Neuroscience
The past decade has seen major advances in our understanding of the physiology of the inner ear, the genetics of hearing loss, and the pathophysiology of disease states, and injury to the inner ear. Major technological developments including those in microscopy, genetic manipulation, electrophysiology, cell culture techniques, computer processing, and others have paved the way for neuroscientists, geneticists, physiologists, molecular biologists, and others to explore and deepen our knowledge of the intricate, exquisite structure and function of the inner ear. This chapter, divided into sections on genetics, hair cell regeneration, and otoprotection strategies, is not meant to be an exhaustive discussion of all techniques used in sensory neuroscience; rather, it is intended as an overview to give the reader a basic understanding, with key references for outside reading, of the exciting techniques neuroscientists use to understand the structure and function of the inner ear.
Identifying Deafness Genes and Determining Their Functional Relevance in the Inner Ear
The field of auditory neuroscience has benefited from genetic investigations combined with state of the art cellular, molecular, and physiological studies. Numerous genes responsible for nonsyndromic forms of hearing loss, defined as hereditary hearing loss manifesting as the only clinical disorder1 and syndromic forms of hearing loss, where hearing loss accompanies other characteristic physical features or disorders, have been identified and located to specific regions of the human genome (see hereditary hearing loss homepage, http://hereditaryhearingloss.org/). Nonsyndromic deafness loci are named with the root “DFN” and then are classified based on modes of inheritance. Autosomal dominant mutations are termed “DFNA,” autosomal recessive mutations “DFNB,” and sex-linked mutations “DFNX.” Each mutation is then numbered in succession of discovery. Subsequently, numerous mouse models have been generated to study the implications of these mutations identified either in patients or through mutagenesis experiments (accessible on the Jackson Laboratory Web site: http://hearingimpairment.jax.org/index.html). The role and importance of numerous other genes essential for hearing and balance have also been revealed by in vitro and in vivo manipulations. Many of the genes identified in these studies are expressed in inner ear tissues, and their corresponding protein products have been discovered. The following sections detail experimental approaches used to identify specific deafness genes and highlight some of the techniques commonly used in a research laboratory to explore the structural and physiological role played by these genes in the inner ear.
Identifying Deafness Genes
The process of identifying deafness causing genes typically begins with developing pedigrees of families segregating deafness. Linkage analysis is first performed to establish a candidate chromosomal region where the deafness causing mutation resides. Linkage analyses use polymorphic genetic markers such as single nucleotide polymorphisms (SNPs) or short tandem repeats that are found throughout the genome at known locations. During meiosis, homologous chromosomes cross over and alleles and SNPs are segregated at a frequency correlative of their genetic distances. SNPs located closer together tend to be recombined less frequently, so linkage analyses investigate, which SNPs tend to be commonly linked with a deafness causing mutation.
For example, the deafness locus DFNB28, an autosomal recessive mutation, was mapped in this manner in a Palestinian kindred2 and in kindreds from India and Pakistan.3 DFNB28 was found to be linked with and located in proximity to the genetic marker D22S423, which lies on chromosome 22q13.1.
Linkage intervals (the smallest chromosomal length found to be linked with the phenotype) tend to be quite large and encompass multiple gene loci. This region can be further refined through techniques such as homozygosity mapping and haplotype analysis. Haplotypes are regions of alleles or SNPs that are transmitted together. Haplotype analysis looks for the smallest hereditary hearing impairment-containing haplotype among affected individuals. From this linkage interval, positional cloning is performed where all genes are sequenced to discover the disease causing mutation. In this manner, mutations in the TrioBP gene were shown to cause DFNB28. Animal studies then provide information on the function of this gene in hair cells and why its mutation causes deafness.
Antibodies generated against TRIOBP, a cytoskeleton-associated protein, determined that the protein localizes to the rootlets of stereocilia in mice. To determine how TRIOBP contributes to proper hair cell function from stereocilia rootlets, mutant mice were created which have mutations in TRIOBP and are incapable of making the functional protein.4 The stereocilia in these mice were more flexible and lacked rigidity, which led to the conclusion that TRIOBP, was required to anchor and provide durability and mechanical rigidity for stereocilia (Fig. 42.1). The combination of these tools, the identification of deafness causing genes and the generation of mutant mouse models to determine their function, have successfully determined the required role of several key proteins in the hair cell and its stereocilia bundle.
Forward genetics, a technique used to discover genes that can cause specific phenotypes, has also been employed successfully to discover hearing impairment genes.5 Random mutations are introduced in an organism through radiation or chemical mutagenesis such as N-ethyl-N-nitrosourea. These individuals are bred through several generations to generate a mutant population, which is then screened for balance and hearing impairments. Once an interesting phenotype is revealed, the inner ear is further studied for structural and functional defects, and the mutated genes are identified through mapping and positional cloning. This approach has been successful in discovering genes required for hearing in mice. Zebrafish are also a common organism used in these screens as they have short generation times and have been used, for example, to identify genes required for sensory cell regeneration.6
Assessing Inner Ear Structural Defects
Once a new animal model possessing an interesting hearing and/or balance phenotype is identified, the investigator typically assesses the integrity, morphology, and function of the inner ear structures. When malformations of the inner ear are observed at birth, prenatal work may be performed to assess morphogenesis during the development of the inner ear. Various technologies are commonly used to analyze the overall morphology of the inner ear such as the paint fill method developed by Dr. Donna Fekete,7,8 transmission electron microscopy (Fig. 42.1), scanning electron microscopy, or immunostaining using specific molecular markers on fixed tissues. Such approaches can reveal major morphological abnormalities, such as a collapsed or shortened cochlear duct, or more subtle defects such as misoriented hair bundles (i.e., defects in planar polarity), shorter stereocilia, or absence of interciliary links.
Electrophysiology of the Inner Ear: A Functional Assay at the Cellular Level
As the sensory receptors of the inner ear, hair cells are the first actors in a chain of events that leads to sound perception. Much effort has focused on identifying and characterizing proteins that are essential for hair cell structure and function, mechanotransduction, the conversion of mechanical energy to electrical energy. Voltage dependent and mechanosensitive channels suspected to be expressed in hair cells can be studied in vitro using the patch-clamp technique.9,10 The sensory cells can either be isolated following a mechanical and/or enzymatic dissociation, or the entire sensory epithelium can be acutely dissected out and pinned down in a recording chamber (Fig. 42.2). Placed under the microscope, hair cells are bathed in an extracellular sodium-enriched solution and approached by an electrode filled with a potassium-enriched solution that mimics the intracellular media. Using the whole cell tight seal configuration, voltage and mechanosensitive channels can be studied either under voltage clamp (cells are clamped to a chosen voltage and the resulting currents are recorded) or current clamp (current is injected into the cells and the resulting voltage is recorded). The stimulus can be a voltage step, a current step or a mechanical stimulus which in this case would be a small displacement of the apical hair bundle (Fig. 42.2). Current and voltage responses are precisely analyzed in terms of their biophysical characteristics and compared with responses obtained in mutant models. This knowledge can also be used to identify hair cell precursors which may be derived from pools of stem cells.11 Similar work can be performed at the neuronal level, both analyzing the transmission from the sensory cells to the afferent fibers and characterizing electrophysiologically the afferent and efferent fibers.
Figure 42.1 Ultrastructural examination of stereocilia rootlets in transmission electron micrographs of 16 day-old wild type and Triobp mutant mice (Triobp⋄ex8/⋄ex8), which fail to produce functional TRIOBP protein. Stereocilia of Triobp mutant mice do not form rootlets, which normally insert into the cuticular plate.
Inner Ear Gene Transfection
While mouse models are extremely informative for identifying and studying vital components of hair cell mechanotransduction machinery, the making of knockouts (an endogenous gene is made inoperative), knockins (an exogenous coding deoxyribonucleic acid (DNA) sequence is inserted in a precise locus in the genome) or transgenic models (exogenous coding DNA sequences are randomly integrated into the genome) remains costly and time consuming. Transfection, the introduction of a specific gene into the cells of the inner ear, whether in vitro, in vivo or even in utero, has become a unique and powerful tool for quickly studying the role of a specific gene. Both overexpression and knockdown approaches can be used to reveal the normal function of a candidate gene. Various gene transfer techniques have been successfully applied:
Figure 42.2 Three types of electrophysiologically relevant information can be recorded when studying a sensory hair cell using the whole cell patch clamp configuration. (A) Voltage-dependent currents: under voltage clamp configuration, the voltage is stepped from a holding voltage of −60 mV to voltages ranging from −110 to +100 mV (⋄ Vm). The resulting currents which are recorded through the same electrode reflect the activation and opening of voltage dependent channels. Recorded from acute postnatal P6 apical outer hair cell (OHC). (B) Transduction currents: under voltage clamp configuration, while the voltage is maintained constant at −60 mV, hair bundles are stimulated by a glass fiber which is displaced rapidly in the excitatory direction (toward the longest stereocilia) and inhibitory direction (toward the shortest stereocilia). The currents which are recorded result from entry of ions thought mechanosensitive channels present at the tip of the stereocilia. Recorded from acute postnatal P3 basal OHC. (C) Receptor potential: under the current clamp configuration, a current that mimics a transduction current induced by a 10 Hz wave stimulus is injected into the cell and the resulting change in voltage is recorded. This voltage response, the so-called “receptor potential,” mimics the true in vivo response. Recorded from acute postnatal P3 OHC.
• Gene Gun-mediated transfection: Exogenous DNA can be delivered into cells in vitro or in vivo by bombardment of a targeted cellular surface by micron-or submicron-sized DNA-coated gold particles. Various constructs such as messenger ribonucleic acid (mRNA), siRNA (small interfering RNA–double stranded RNA molecules designed to interfere with (“knock down”) the expression of a specific gene), or DNA can be delivered using this technique.12
• In vitro electroporation: This technique can be used to transfer genes in inner ear explants extracted from embryonic or early postnatal ears.13 Electrical square waves are applied to the explants to introduce DNA in individual cells within the cochlear duct. A video presentation of this technique can be viewed online.14
• In utero electroporation: A plasmid encoding a gene of interest is microinjected through the uterine wall into the lumen of the otocyst and electroporated into otic epithelial progenitor cells.15
• Use of viral vectors: Several viral vectors have been shown to transfect the varying cell types of the mammalian inner ear including hair cells and supporting cells: adeno-associated virus, adenovirus, and lentivirus.16 Numerous laboratories use these viral vectors routinely to introduce exogenous genes in hair cells to disrupt or repair endogenous genes and functionally assess their effects. Such vectors have been show to successfully transfect human hair cells in vitro.16 Several routes of delivery have also been identified for introduction of vectors to the inner ear in vivo while minimizing injury to existing structures and at the same time ensuring widespread distribution of the agent throughout the cochlea and the rest of the inner ear. These studies raise the possibility that gene transfer may be developed as a potential strategy for treating inner ear dysfunction in humans.
Imaging
The advent of confocal microscopy and more recently multiphoton imaging has made possible observing cells in real time with high resolution. These fluorescence miscroscopy-based methods minimize out of focus light, creating an optical sectioning effect analogous to MRI. This eliminates much of the noise and light scatter that reduces contrast in thick specimens such as inner ear organs. Faster systems can easily make time lapse recordings of cells in real time. Since they are fluorescence-based techniques, different cell types can be labeled with vital dyes, or they can be genetically modified to express fluorescent proteins that emit light at various wavelengths in the visible spectrum. For example, transgenic mice have been generated in which haircells or supporting cells express a fluorescent protein under the control of a promoter that is specific to those cell types.17
FM1 43, a styryl dye that is known to permeate hair cell transduction channels when applied for a very brief period18,19 is also commonly used to identify hair-cell populations and assess their mechanosensitivity (Fig. 42.3). Using this technique,20 were able to demonstrate a progressive maturation of the transduction apparatus along the developing organ of Corti over the first postnatal week (Fig. 42.3). While this technique is only qualitative and cannot replace electrophysiological recordings of transduction, it allows researchers to image an entire population of sensory cells at once and therefore gives a quick functional diagnosis which will guide the design of further investigations. It should also be noted that these imaging techniques are not limited to electrophysiology studies and are invaluable to all areas of inner ear research.
Regenerating Hair Cells: From Bench to Bedside
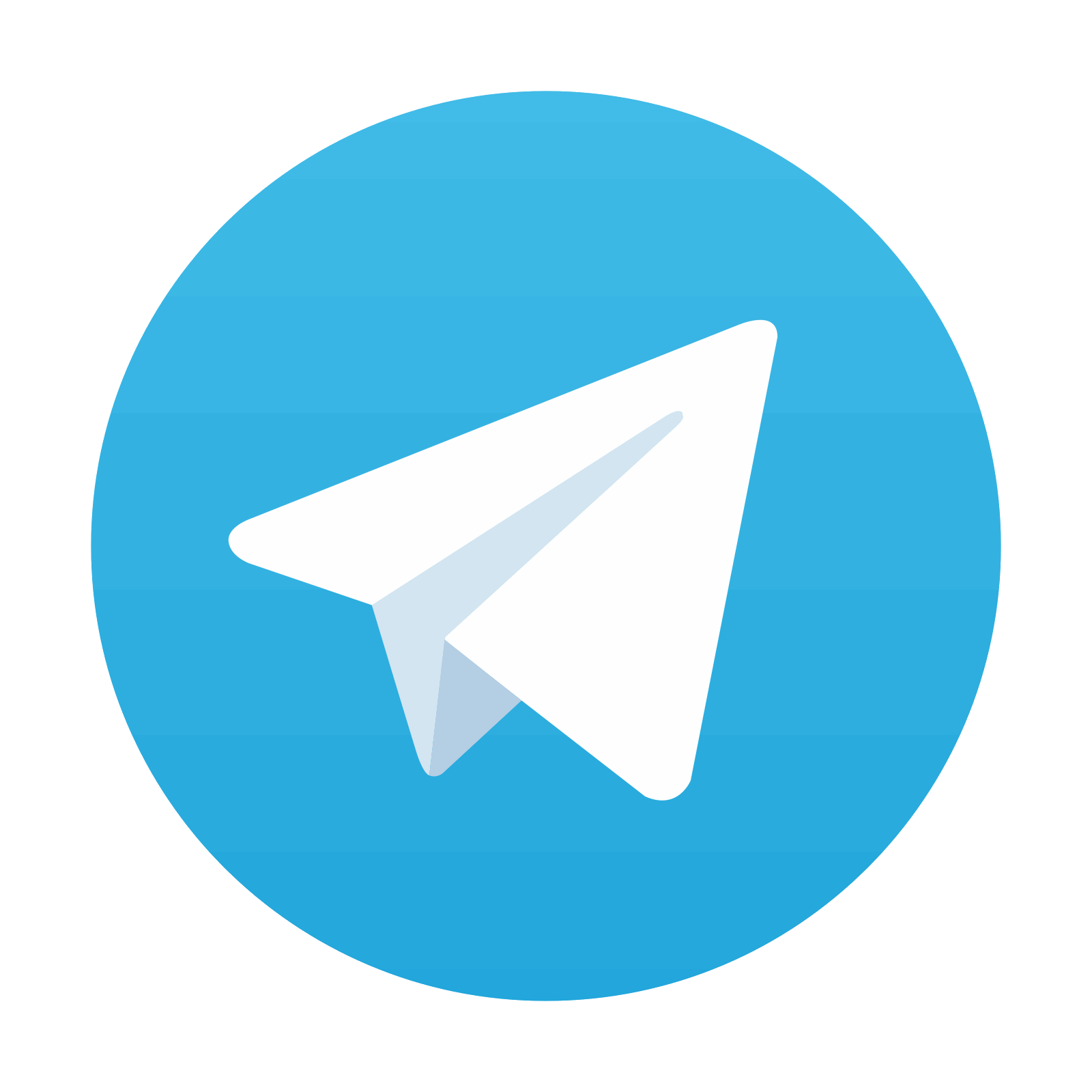
Stay updated, free articles. Join our Telegram channel

Full access? Get Clinical Tree
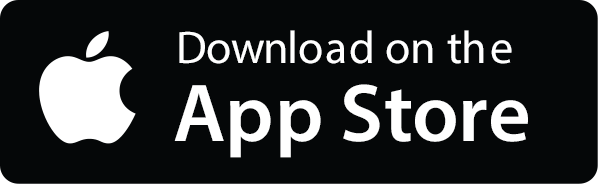
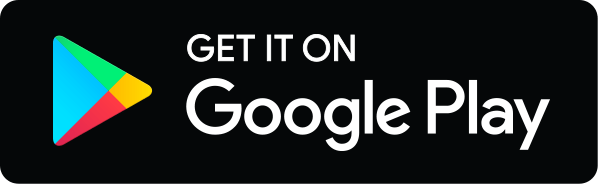