1.1 Fluorescein Angiography – Known hypersensitivity to fluorescein derivatives. Some companies specify that beta-blocker treatment is a contraindication. Beta-blockers do not interact with fluorescein directly, but allergic reactions are more difficult to treat. – There are no medically established contraindications in pregnancy. Despite this, the examination is carried out in pregnant women only in exceptional circumstances. – Sodium fluorescein has some limited vasoconstrictive and photosensitizing characteristics (skin irritation by sunlight after injection); however, these are not considered to be serious or significant. – Age-related macular degeneration in unique clinical diagnosis without therapeutic consequences (for example, disciform scarring or geographic atrophy, dry and atrophic scarring after photodynamic therapy). – Diabetic retinopathy for the diagnosis of a clinically significant macular edema or as a “routine check-up.” – Unexplained loss of visual acuity in a clinically normal-appearing macula; no additional information can be expected from angiography. – Choroidal melanoma. Angiography provides little additional information in cases of suspected choroidal melanoma. – Occlusion of retinal vessels. The diagnosis can usually be established without angiography. Reliable conclusions regarding treatment can only be made in cases of vein occlusions, and then usually only months after vessel occlusion. It is debatable whether angiography can determine the necessity of panretinal laser coagulation following retinal vein occlusion. – Hereditary retinal dystrophies. Fundus autofluorescence usually provides sufficient information, even earlier or in more detail than fluorescein angiography, which is only appropriate when exudative complications are expected. Bloom JN, Herman DC, Elin RJ, et al. Intravenous fluorescein interference with clinical laboratory tests. Am J Ophthalmol 1989;108: 375–9. Halperin LS, Olk RJ, Soubrane G, Coscas G. Safety of fluorescein angiography during pregnancy. Am J Ophthalmol 1990;109:563–6. Holz FG, Jorzik J, Schutt F, Flach U, Unnebrink K. Agreement among ophthalmologists in evaluating fluorescein angiograms in patients with neovascular age-related macular degeneration for photodynamic therapy eligibility (FLAP-study). Ophthalmology 2003;110:400–5.
Fluorescence and Fluorescein Angiography
The term “fluorescence” refers to solid, liquid, or gaseous matter that emits absorbed radiated energy in the form of electromagnetic radiation at similar or longer wavelengths.
In fluorescence angiography, a fluorescent substance is administered intravenously; shortly afterwards, it spreads through the choroid via the bloodstream and retinal vessels. Stimulating light enters the eye through a filter and then, as a result of the substance’s fluorescence, produces light emission at varying wavelengths, which are reduced to appropriately defined emission spectra through a second filter; these can then be photographed and/or filmed.
Two different intravenous fluorescent substances are currently in use in ophthalmology: fluorescein and indocyanine green. The two substances differ in their absorption and emission spectra, in their pharmacological characteristics (particularly their binding properties to plasma proteins) and in their elimination and passage through vascular walls in the choroid and retina.
The development of the methodology and the clinical applications of fluorescein angiography are based on the work of Chao and Flocks, Novotny and Alvis, and MacLean and Maumenee. These angiographic methods have been in regular use for retinal diagnosis since the 1960s. In Germany, fluorescein angiography was first introduced, developed, and popularized by Wessing.
Sodium Fluorescein
Sodium fluorescein (C20H10O5Na2, MG 376) is the dye most commonly used for fluorescence angiography throughout the world. After agitation, the fluorescence lasts for 10−9 seconds (there is therefore no “afterglow” as in phosphorescent substances).
Both the absorption and emission spectra of sodium fluorescein depend on various factors; with intravenous administration, they are 465 nm (absorption) and 525 nm (emission).
Approximately 70–80% of the sodium fluorescein binds to plasma proteins; 20–30% does not bind. Injected fluorescein is diluted in the bloodstream by a factor of approximately 600 and disperses throughout the whole body. With the exception of the central nervous system vessels, the retina, and to a limited extent the iris, unbound sodium fluorescein can freely permeate all blood vessels in the body. A few minutes after injection, the skin, mucosa (visible up to 6 h after injection), and urine (visible up to 36 h after injection, or longer if kidney function is limited) show signs of the yellow dye. Some laboratory values can be affected until the dye is completely eliminated from the body. The elimination of sodium fluorescein predominantly takes place through the kidney rather than the liver.
Contraindications:
Prerequisites for Fluorescein Angiography
Despite evidence of very good general tolerance of injected sodium fluorescein, the physician administering or supervising the treatment should be adequately trained in emergency procedures. Medication required during an emergency must be administered reliably and without delay. Immediate notification of the need for emergency care and implementation of it must be ensured (see Chapter 2).
Secure intravenous access is important; if extravascular or intra-arterial injection take place, severe side effects such as skin necrosis or intense yellow discoloring of the surrounding skin can be expected. Intravenous access should be maintained until the examination has been completed.
The following equipment is essential: a fundus camera with automatic film transport, or a digital camera; an electronic flash unit with an exciter filter of 465– 490 nm (the blue–green spectrum); and a recording filter of 520–530 nm (the green–yellow spectrum). Enlargement and editing of images for retinal analysis can be carried out (depending on the type of fundus camera used) using the various sizes of field available. A standard exposure setting of 30° for macular diseases is routinely used; in addition, 20° (the largest enlargement possible with a small section) and 50° or 60° exposures are also used.
Photographic documentation of the filtered, emitted light is recorded on highly sensitive black-and-white film, or in electronic image files with a digital camera system. Newer scanning laser ophthalmoscopes (SLOs) are also usually equipped to handle fluorescein angiography. Digital documentation systems have been increasingly used in practice in recent years. These have the advantages of allowing immediate assessment and editing of the images (with adjustment of contrast and brilliance) and also of providing electronic archiving and data storage, enabling fast computer-networked access to the data from several locations.
At present, however, film documentation still provides higher resolution. In private practices and most clinical centres, digital technology has established itself as the main examination method.
Experience and knowledge on the part of the photographer, along with cooperation by the patient, determine the quality of the images taken for analysis. Other factors that can have a negative effect on the quality of an angiogram are: clouding of the media; insufficient pupil dilation; too slow or insufficient injection of dye; and unreliable or badly configured equipment or faulty processing of the angiograms.
Practical Procedure
Before angiography is started, it is recommended to take a color exposure and a blank black-and-white exposure of the fundus. There should always be clarity about the structures to be examined, any potentially important phase of the angiography to be observed, and the enlargement chosen. Photographic documentation of the findings in the second eye should also be obtained in order to allow comparisons with the eye being treated and for monitoring.
Angiography starts with an intravenous bolus administration of 5 ml 10% sodium fluorescein solution (equivalent to 500 mg active agent; today’s commercially available compounds usually come in ready-made solution vials). For patients with renal insufficiency, a reduced dose of 2.5 ml of the appropriate solution is recommended. A stopwatch should be used during the injection in order to plot the chronology of the exposures.
Inflow of dye into the choroidal vessels can usually be seen after 10–15 s; this is directly followed by the retinal circulation. Experienced photographers can “anticipate” the inflow of the dye and immediately start taking sequential exposures. The fast arterial and arteriovenous phase of the angiography which then follows places very high demands on the photographer, who has to take sequential exposures (starting with approximately one image per second) within a very short period of time, continuing until the maximum fluorescence is reached (this is usually 25–35 s after the start of the angiography). Angiography is concluded with the last exposures being taken 5, 10, or 15 min after the initial injection. The protocols used at different institutions vary depending on the profile of the disease and the institution carrying out the angiography.
After completion of the arteriovenous phase, additional exposures of the fundus periphery (for example, in diabetic retinopathy) or the second eye (for example, in macular degeneration) should be made in accordance with the diagnosed profile of the disease. Parallel complete angiography of both eyes is not possible with standard equipment.
After completion of the angiography, six to eight typical exposures (determined by the analysis technique used) of the individual phases of the angiography should be selected and enlarged—or, where applicable (digital angiography), digitally processed and saved for documentation purposes—and printed out. Storage of all unenlarged negatives—in other words, complete picture data—is recommended in all cases.
In situations in which the resulting images are inadequate for interpretation, the angiography can be repeated in most diseases after 2 h, at which time no fluorescence phenomena can usually be detected in the choroid vessels or in the retina. However, in larger studies, a 48-h interval is generally recommended.
Using the appropriate equipment, fluorescein angiography and indocyanine green angiography can be carried out, either in parallel or shortly after each other. Mutual interference between the fluorescence phenomena displayed does not occur, although some investigators have reported an increase in side effects during simultaneous angiography.
Interpretation
The interpretation of fluorescein angiography is a subjective method that is influenced by the timing of individual exposures, their quality, the actual exposures chosen for analysis, the way in which the picture is viewed, and finally the experience and qualification of the investigator. Interobserver and intraobserver differences in the interpretation of fluorescein angiography can occur.
When in doubt, cases should be discussed with an experienced colleague; with the advent of digital angiography and the internet, this has become a fast and relatively simple procedure.
The analysis and diagnosis of angiographies are carried out in different ways. Technically, the best method is analyzing stereo angiography (see section 1.4). Another alternative is the single enlargement of a 35-mm film angiogram or monitor image, which can also be appropriately printed out for consultation purposes. Selection, modification and printout of single images should be carried out by the investigator or an experienced photographer in a standardized way. In any case, storage of all unedited pictures taken during angiography is advisable.
For interpretation, the following patient information is important: medical history; course of the disease; previous treatment; visual acuity; and concomitant diagnosis.
The clinical diagnosis should be known to the consultant, who should at least receive a color image. Color images help identify structures that may be hard to define with angiography alone.
The availability of previous angiograms is helpful for monitoring purposes—for example, relating to the enlargement or reduction of a choroidal neovascularization after treatment.
During interpretation, the examiner determines whether the angiographic findings are normal and allocates them spatially (anatomical structures) and chronologically (early, middle, or late phase of the angiography). Ideally, diagnosis and the resulting treatment are based on a combination of the clinical findings and angiographic findings.
Avoiding Unnecessary Angiographies
Like all diagnostic procedures, fluorescein angiography is used to diagnose conditions in order to determine the need for therapeutic procedures and to follow up specific diseases.
Despite good tolerance and high levels of safety, fluorescein angiography is still an invasive procedure that can potentially lead to serious side effects. Unnecessary angiography should therefore be avoided.
Angiography should not be performed if a diagnosis can be made without it or if no therapeutic consequences would result from the procedure. Angiography is also unsuitable as a method of routine check-up or for documentation purposes—for example, in the course of a diabetic macular edema or macular degeneration.
In our experience, numerous unnecessary angiographies are often carried out in the following groups of diseases in particular:
Normal Fluorescein Angiography
Blood flow in the choroidal and retinal vessels can be visualized using fluorescein angiography. After injection into a peripheral vein, the sodium fluorescein reaches the vascular system in the eye via the blood circulation system and the central retinal artery. Sodium fluorescein diffuses quickly within the fluid compartments of the eye, but it does not usually pass through the retinal vessels and the retinal pigment epithelium (RPE). Fluorescein angiography therefore examines the integrity of the blood–retina barrier.
Two vessel systems, the choroidal vessel system and the retinal vessel system, with differing degrees of extravasation of fluorescein, fill almost simultaneously. These are separated by the RPE. Due to the presence of tight junctions, fluorescein is virtually incapable of permeating the vascular walls (the internal blood–retina barrier) of normal retinal vessels and large choroidal vessels. The dye freely permeates the fenestrated vessels of the choriocapillaris and spreads very rapidly within the choroid.
As well as acting as an optical barrier to stimulating and emitting light, the RPE also acts as a barrier against fluorescein, because of its zonula occludens. The pigment content of the RPE in patients of African or Asian descent is comparable to that of less pigmented races. The difference between the lightness of the background of the eye is based on the variable pigmentation within the choroid.
The first visible change is that the choriocapillaris of the choroid is filled via the posterior ciliary arteries. The flooding of the fluorescein relates to the lobular structure of the choriocapillaris. Although there is no immediately visible barrier, interindividually variable segment-shaped filling of the choriocapillaris is noticeable. In the periphery, these patches resemble spindle-type segments. The long posterior ciliary arteries fill a nasal and temporal segment. Early choroidal vessel filling is only of diagnostic significance in particular lesions (for example, choroidal hemangioma) or when there is patchy and delayed filling (for example, in anterior ischemic optic neuropathy or ocular ischemic syndrome).
Cilioretinal vessels may fill at the same time as the choriocapillaris and, as a rule, slightly earlier than the actual retinal vessels.
The first signs of the dye in the retinal vessels can be seen 10–15 s after the start of the injection (known as the “arm–retina time” and averaging about 11 s in healthy young individuals). Filling of the retinal vessels takes place 0–2 s after filling of the choroidal vessels. The arteriovenous passage time (the time between the first signs of the dye in the arteries close to the optic nerve head and the first signs in the veins) averages 1.5 s. Another definition of the arteriovenous passage time is the time span between the first signs of the dye in the temporal arteries and complete filling of the large temporal retinal veins (normal value up to 11 s).
The retinal vessels are located in the inner two-thirds of the retina; the outer third, incorporating the photoreceptors, is vessel-free. The arterial branches represent end arteries without anastomoses. The retinal arteries and veins course in the ganglion cell layer; the capillaries form deep, extensive interweaving layers that reach the outer plexiform layer. A distinguished surface capillary layer is visible in the peripapillary area.
After injection, the dye spreads into the central retinal artery and into the retinal arteries, initially with an axial flow. The unbound fluorescein in particular is visible, which in practice represents the plasma flow. Since the column of erythrocytes in the blood vessels is seen in normal fundus images, the vessels appear comparatively wider on fluorescein angiography. Stronger fluorescence is noticeable in anemic patients due to the relatively high plasma content.
All arterial branches are filled within one or two seconds, after which transit into the capillary area, followed by the macula area, can be observed. The orientation and density of the capillary network shows regional variations (macula, optic nerve head, periphery). Due to their high xanthophyll concentration and increased pigmentation resulting from blockage of choroidal fluorescence, the central macular areas appear darker.
Simultaneously with the filling of the arteries in the fundus periphery, filling of the retinal veins also starts in the retina. As the fluorescein arrives in the central venules prior to the peripheral venules in the efferent veins, laminar flow can be seen in the vessels. Approximately 25 s later, the dye is evenly distributed in the arteries and veins; at this point, the perifoveal capillary network can be seen best. After reaching a maximum concentration level, the visible intraluminal fluorescence in the retinal vessel decreases.
In the meantime, the dye leaves the fenestrated vessels of the choriocapillaris within a few seconds and diffuses into the choroidal tissue, resulting in a relatively homogeneous background fluorescence that reaches its climax more or less parallel to the filling of the retinal capillaries. Subsequently, the fluorescein molecules in the choroidal vessel follow a corresponding concentration gradient (by diffusion) back into the vessel. As a result of dilution and elimination, the background fluorescein decreases.
Both the posterior ciliary arteries and numerous retinal capillaries are visible on the optic nerve head, resulting in distinct coloring of the optic disc during the course of the angiography. A low-grade leakage phenomenon can also be encountered in the optic disc area as a result of the fenestrated peripapillary choriocapillaris and staining of the lamina cribrosa in normal angiograms.
The last phenomenon in fluorescein angiography is staining of the inner scleral layers, which is visible as a result of contrast with the choroidal vessels.
There is no standardized pattern for describing the various phases of angiography; individual interpretations and arbitrary classifications are used. In general, however, a distinction is made between an early phase (the filling of the retinal arteries or “arterial phase”); a middle phase or arteriovenous phase (the arteriovenous transition, often further subdivided into early, middle and late arteriovenous phases); and a late phase (in which the fluorescein angiographic phenomena decline). The timing of the late phase is handled in a variety of ways; usually, an exposure is carried out after 5 min. In specific diseases (for example, distinct leakage or staining in exudative macular degeneration), exposure after 10 min is necessary.
Identifying Abnormal Appearances in Fluorescein Angiography
The interpretation of fluorescein angiography is based on the identification of fluorescein phenomena that differ from those in normal angiography. These aspects are placed in a spatial and chronological context and are compared with known changes in specific diseases.
Angiographic phenomena that differ from those of normal angiography can be divided into two groups: hyperfluorescence and hypofluorescence. Hyperfluorescence means added fluorescein detection in comparison with normal angiography. Leakage shows the transfer of fluorescein into the surrounding tissue, usually from permeable vessels. Hypofluorescence, in contrast, means weaker fluorescein detection in comparison with normal angiography.
The causes of hyperfluorescence and hypofluorescence are numerous. Furthermore, both hyperfluorescence and hypofluorescence can occur in various combinations within one and the same angiographic examination.
Hyperfluorescence and hypofluorescence should always be interpreted according to the anatomic structural relationship, the phases of angiography, and the possible changes during the course of angiography. Consequently, early-phase hyperfluorescence can become late-phase hypofluorescence and vice versa.
Hyperfluorescence
Hyperfluorescence can arise in what are known as “window defects,” in newly formed vessels, in vessel-conducting structures, or due to dysfunction of the inner or outer blood–retina barrier.
In window defects, increased visibility of the normal choroidal vessel fluorescence takes place because of a defect in the RPE. An early sharply demarcated hyper-fluorescence that decreases in the subsequent course is typical. Leakage phenomena (with the hyperfluorescence spreading like the smudging of watercolor paint) are not encountered.
In newly formed vessels or vessel-conducting structures, hyperfluorescence arises from their location, also producing additional fluorescence in the corresponding anatomical structures. In addition, newly formed vessels are usually more permeable to unbound fluorescein. Early hyperfluorescence as a result of filling of the newly formed vessels in the arterial phase followed by an increase in the subsequent course as a result of leakage is typical. In contrast to neovascularization, collateral formation typically shows weaker leakage phenomena.
Damage to the external blood–retina barrier caused by functional changes in the RPE is visible mostly in the form of late hyperfluorescence, through an accumulation of the dye in front or behind the RPE (for example, in central serous chorioretinopathy).
Damage to the inner blood–retina barrier mainly results in extravascular and increasing hyperfluorescence with leakage phenomena (for example, in retinal vasculitis).
Hypofluorescence
Hypofluorescence occurs as a result of a decrease in the stimulating or emitting light or the dye-conducting structures.
Classically, hypofluorescence occurs due to the blockage of normal fluorescence (for example, “exclusion” of the stimulating light and occlusion of the choroidal fluorescence as a result of retinal hemorrhage), or as a result of reduced perfusion (for example, in arterial occlusion).
The transition from initial hypofluorescence to subsequent hyperfluorescence can typically be observed in inflammatory changes both to the retina and to the choroidal vessel, and in specific tumor-like changes. The initial hypofluorescence is explained by blockage phenomena (for example, in retinal edema or tumor), followed by a hyperfluorescence as a result of added vessels or more permeable vessels within these structures.
References
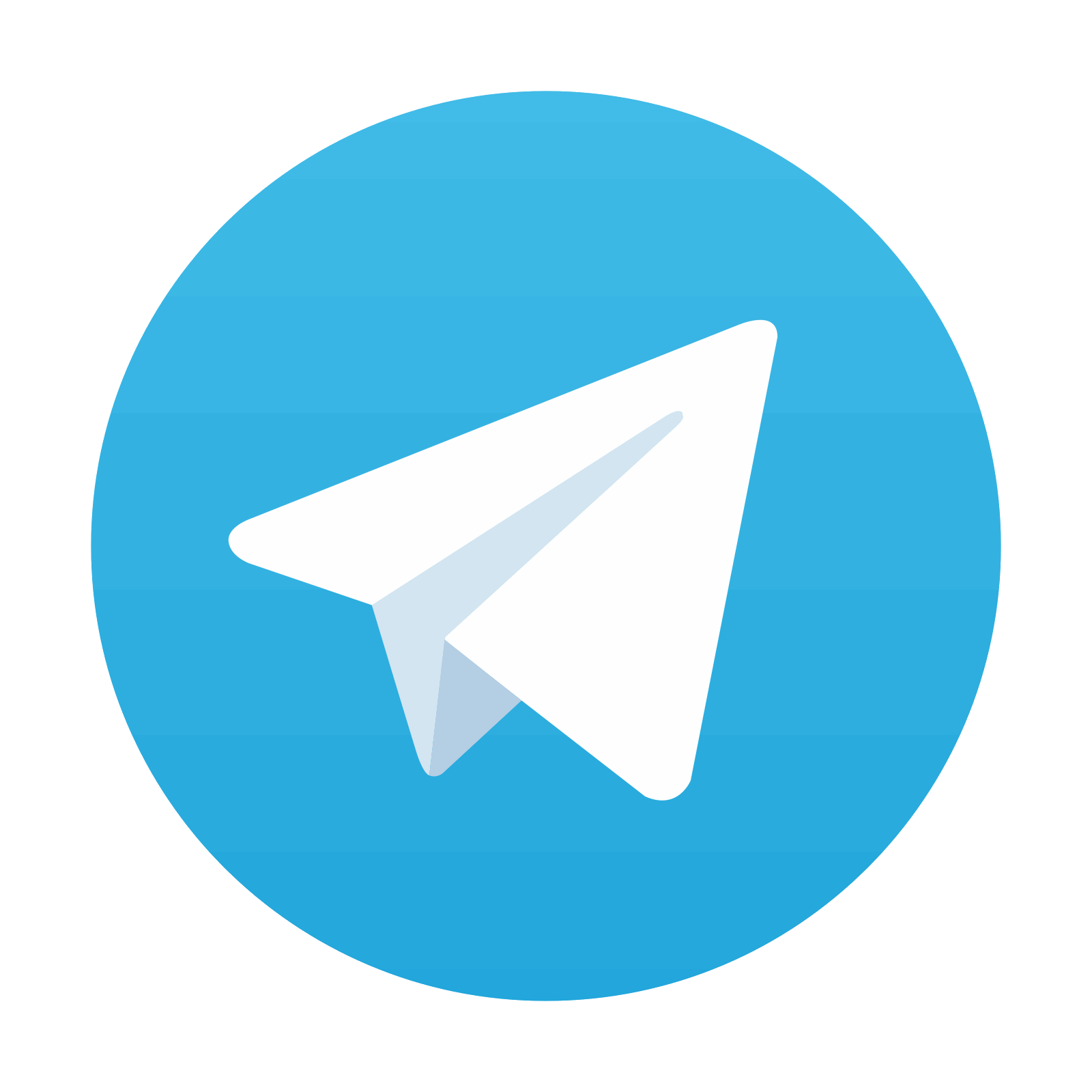
Stay updated, free articles. Join our Telegram channel

Full access? Get Clinical Tree
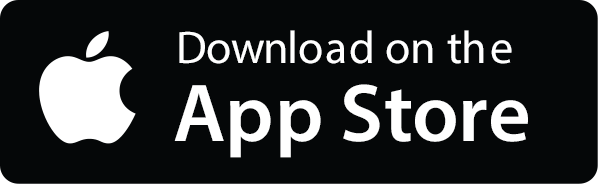
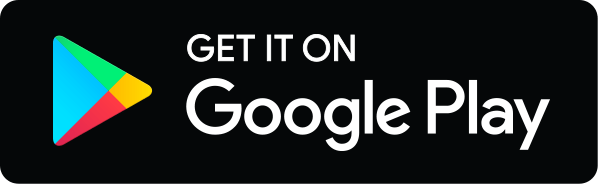