© Springer Science+Business Media Singapore 2017
Kimitaka Kaga (ed.)Cochlear Implantation in Children with Inner Ear Malformation and Cochlear Nerve DeficiencyModern Otology and Neurotology10.1007/978-981-10-1400-0_33. Embryology of Cochlear Nerve and Its Deficiency
(1)
St. Thomas’ Hospital, Westminster Bridge Road, London, SE1 7EH, UK
Abstract
Most studies on development of the human auditory system and hearing loss have to date focused on the sensory apparatus, the cochlea. With advancements in magnetic resonance imaging (MRI), there has recently been increasing interest in the subject of cochlear nerve deficiency (CND) and dysfunction. Cochlear nerve deficiency (CND) amongst individuals with congenital sensorineural hearing loss (SNHL) is not as rare as previously thought, with prevalence as high as 18–21 % reported amongst cochlear implant recipients. Cochlear nerve (CN) morphogenesis is a complex process involving cell populations from two disparate progenitors of the otic placode and neural crest cells. In the first trimester, the basic foundation of the auditory pathway is laid down, with the vestibulocochlear ganglion cells delaminating from the otocyst and establishing peripheral and central connections with the developing cochlea and brainstem, respectively. The second trimester is a period of proliferation, growth and myelination. As the number of axons is pruned back closer to the adult level, myelination begins in the intra-cochlear portion of CN and extends proximally. In the third trimester, further maturation of the neuronal connections in conjunction with paralleled development of the cochlea and brainstem leads to emergence of foetal responses to auditory stimuli. Based on the currently available knowledge of the embryological development of CN, various phenotypes of CND are discussed. It is hoped that better understanding of CN ontogenesis will not only lead to further refinement of auditory implant candidacy but also open doors to potential regeneration therapies such as stem cell therapy in the future.
Keywords
EmbryologyDevelopmentCochlearNerveDeficiency3.1 Introduction
Hearing loss is the commonest congenital sensory deficit, affecting approximately 1 in 1000 live births in most developed countries. In certain communities, the incidence may be up to three to four times higher. In the majority of cases of congenital sensorineural hearing loss (SNHL) , the location of malfunction is within the inner ear. It is therefore not surprising that most studies on development of the human auditory system have to date focused on the sensory apparatus, the cochlea.
More recently, there has been increasing interest in the subject of cochlear nerve deficiency and dysfunction. Advancements in magnetic resonance imaging (MRI) techniques mean that it is now possible to clearly visualise the cochlear nerve (CN) separate from the facial nerve and the superior and inferior branches of the vestibular nerve within the lateral portion of the internal auditory meatus. As access to MRI continues to improve around the world, it has become the imaging modality of choice in many centres for aetiological investigation of SNHL and as part of cochlear implant assessment. As a result, abnormalities of the cochlear nerve are coming under increasing attention, although their clinical implications are not always clear. Cochlear nerve deficiency (CND) amongst individuals with congenital SNHL is not as rare as previously thought, with prevalence as high as 18–21 % reported amongst cochlear implant recipients in recent studies [1–3]. Furthermore, as outcomes of cochlear implantation improve overall and implant candidacy continues to expand, there is closer scrutiny of potential prognostic factors, including congenital anomalies of CN as well as those of the inner ear, which may negatively impact on the implant outcome. Such is particularly the case when there is a significant doubt as to the presence of a functional connection between the cochlea and the brainstem, and an auditory brain stem implant may need to be considered as a potentially better option.
This chapter provides an overview of the current knowledge of the embryological development of CN and discusses its clinical relevance.
3.2 Embryology
The vestibulocochlear nerve (VCN) , like all peripheral nerves, consists of neurons and supporting glial cells [4]. Peripheral glial cells include Schwann cells, which surround and myelinate neuronal axons and neurites, and satellite cells, which support ganglionic neuronal cell bodies. The glial cell component of VCN arises from neural crest cell (NCC) progenitors of the embryonic ectoderm [5–7]. It is commonly held that the neurons of VCN originate almost exclusively from the otic placode [7–10]. However, it has recently been suggested that a significant proportion of VCN neurons may be traced back to NCC or another type of migratory neuroepithelial cells [11]. Throughout all stages of VCN morphogenesis, there is extensive interaction between the two cell populations from the otic placode and NCC progenitors, developing very much in tandem [12].
3.2.1 First Trimester
In the human embryo, the otic placode begins to develop around 3 weeks of gestation (WG) as a thickening of the epidermis on either side of the head. It soon invaginates and separates from the surface ectoderm to form a spherical epithelial structure, referred to as the otic vesicle or otocyst [13–15]. By 4 WG, a group of cells have delaminated from the otocyst and become stationed between the developing inner ear and hindbrain. After undergoing cell proliferation and differentiation, these neuroblasts give rise to the vestibulocochlear ganglion [16–18].
The ganglion cells that will develop into the cochlear division of the nerve project back to the developing cochlea and wind around the modiolus, forming the spiral ganglia. The immature neurons begin to establish polarity by extending their processes in two directions, peripherally towards the inner ear and centrally towards the brainstem [18, 19]. The central processes, the axons, are the first to reach their destination, arriving in the brainstem by the fifth to sixth week of foetal development. Within the brainstem, all of the auditory centres and pathways are identifiable by 7–8 WG, with clusters of neurons recognisable as the cochlear nuclei [16]. The dendritic processes directed peripherally penetrate the undifferentiated, primordial organ of Corti at 9 WG. By 10–12 WG, they form rounded synaptic terminals, making contact with the bases of the developing hair cells [18, 20]. It has been shown that the migration, growth and survival of the vestibulocochlear ganglion cells are likely to be dependent on a number of neurotrophic factors, in particular brain-derived neurotrophic factor and neurotrophin-3, expressed by the otocyst [21–23].
Another event of significant clinical relevance around this stage in development is the change in the mesenchyme in association with the early sensory apparatus and neural connections. At 9 WG, the mesenchyme surrounding the otocyst begins to form a cartilaginous matrix, which eventually ossifies and turns into the otic capsule. This chondrogenesis occurs in synchrony with the development of VCN, whose presence inhibits cartilage formation at the medial aspect of the otocyst, eventually leading to the formation of the internal auditory canal (IAC) . As with the early development of the vestibulocochlear ganglion cells, which requires various neurotrophic factors produced by the otocyst, the induction of chondrogenesis involves close and reciprocal epithelial-mesenchymal tissue interactions between the otocyst and periotic mesenchyme [24]. The formation of IAC is completed by 5 months of gestational age, and it has been hypothesised that the calibre of the IAC is related to the volume of the migrating VCN fibres [25].
3.2.2 Second Trimester
CN undergoes rapid proliferation and maturation during the second trimester. Around 14 WG, the dendrites extending from the spiral ganglia begin to form synaptic contacts with the inner and outer hair cells of the cochlea. This process of synaptogenesis has been shown to progress along a base-to-apex gradient [26]. The mechanisms by which CN ganglion cells differentiate into type I and type II ganglion cells, establish their connections with the correct, corresponding hair cell types and match the tonotopic development of the cochlea are poorly understood.
During this period, CN not only grows in its diameter, increasing almost threefold in cross-sectional area between 22 and 28 WG, but also begins to develop a distinct fascicular arrangement of axons surrounded by increasingly compact connective tissue. Ray et al. observed in their study of the development of the human foetal nerve that the number of axons continued to increase until 20 WG and subsequently reduced to the adult level at 22 WG [27].
One of the most significant events during the mid-gestation period is myelination, which is crucial for rapid synchronised conduction. Developing Schwann cells are detected in foetal embryos as early as 12 WG. At 15 WG, clusters of Schwann cells are seen along the axons in the spiral lamina and modiolus, and by 20–22 WG, myelination has begun within the spiral lamina fibres, with a well-defined, close association between the axons and Schwann cells [26–28]. By the 24th week of foetal development, myelin sheaths can be seen to extend along the nerve up to the glial junction at the point where the nerve exits from the temporal bone. The proximal segment of CN beyond this point is supported by oligodendrocytes, but myelination does not begin until later [28]. One of the interesting findings in the study of human foetal CN by Ray et el. was the maturational asymmetry between the left and right sides, with myelination of the right cochlear nerve consistently lagging behind the left until 38 WG [27]. The clinical implications of this observation have yet to be elucidated.
3.2.3 Third Trimester
As both the cochlear nerve and the central neural connections continue to mature functionally as well as structurally, foetal responses to auditory stimuli start to emerge. Using vibroacoustic stimulation during ultrasound imaging, blink-startle responses could be observed in some foetuses as early as 24–25 WG, and the responses were present consistently after 28 WG [29, 30]. Studies in preterm infants show that auditory brainstem responses (ABRs) can be elicited from around 26 weeks of conceptional age and become more consistent from 29 weeks, although they require stronger intensity stimuli at slower rates. The ABR thresholds appear to stabilise approximately at adult values around 35 WG, and the latencies of the various potential components continue to decrease to term [31–33].
3.2.4 Summary
The foundation of CN morphogenesis is laid down in the first trimester, with the otocyst giving rise to and sustaining the vestibulocochlear ganglion cells which migrate and form connections with the early cochlea and brainstem. The second trimester is a period of proliferation and maturation. Synaptogenesis begins between the dendrites of the spiral ganglia and inner and outer hair cells and progresses in a base-to-apex direction. The number of axons reaches its peak around 20 WG and then decreases to the adult level at 22 WG. As this pruning process occurs, myelination begins in the intra-cochlear portion of CN and extends proximally. By early third trimester, the foetus starts to show physiological responses to vibroacoustic stimuli, and by 29 WG ABRs can be elicited consistently albeit with stronger and slower stimuli.
3.3 Cochlear Nerve Deficiency
There are two possible pathophysiological mechanisms that may lead to CND: one is complete (aplasia) or partial (hypoplasia) failure in development and the other is post-developmental degeneration. Based on the current understanding of CN morphogenesis, associated factors that may provide some insight include the presence or absence of concomitant inner ear anomalies and/or IAC malformations.
Individuals with congenital CND are significantly more likely to have other labyrinthine anomalies than those without CND [3, 25, 34, 35]. This is not surprising, given that the otocyst gives rise to the cochlea, vestibule, semi-circular canals and endolymphatic sac as well as the vestibulocochlear ganglion. It is therefore plausible that developmental arrest occurring early in the embryonic life would likely lead to CND associated with other congenital inner ear malformations. As has been alluded to earlier, migration, growth and survival of vestibulocochlear ganglion cells require expression of various neurotrophic factors by the otocyst [22, 23, 36], which could explain the high prevalence of inner ear dysplasia in CND. In contrast, it appears that the inner ear development is not dependent on neuronal stimulus or trophic effect [37–39]. It is therefore possible that a disturbance in the trophic effect the cochlea exerts on CN neurons may lead to CND as an isolated finding in the presence of a normally developed cochlea [34, 40].
Another potential indicator to be considered is the size of IAC, which is related to migrating VCN neurons and chondrogenesis in the surrounding mesenchyme. IAC stenosis accounts for about 12 % of all congenital temporal bone malformations [19]. Until it became possible to actually visualise CN on MRI, the finding of IAC stenosis on computed tomography (CT) was thought to indicate VCN aplasia and therefore considered an absolute contraindication to cochlear implantation [41, 42]. Subsequent studies, however, have demonstrated that a stenotic IAC may contain a hypoplastic CN or functional CN fibres travelling with other nerves within IAC [1, 34, 43]. Conversely, CND in association with normal calibre IAC has also been reported [1, 35, 44]; as the development of IAC commences at 9 WG and is completed by 5 months of gestation, such cases may represent either isolated CN agenesis (vascular insufficiency, uncontrolled apoptosis regulation) or post-developmental degeneration of the nerve (perinatal, neurotrophic viral infections ) [35].
A subject that merits discussion in this chapter is auditory neuropathy spectrum disorder (ANSD) . ANSD is a heterogeneous group of conditions characterised by marked discrepancy between cochlear and neural functions in the auditory system. The most widely accepted diagnostic criterion is absent or abnormal ABR in association with normal outer hair cell function as evidenced by present cochlear microphonics and/or otoacoustic emissions. It has been estimated that ANSD may account for up to 10–15 % of newly diagnosed cases of hearing loss in children [45, 46]. The prevalence of CND in ANSD is reported to be as high as 18–28 %, compared to 6–16 % in children with SNHL not diagnosed as ANSD [35, 47–49]. Conversely, over 70 % of children with CND have been found to have the audiometric features of ANSD [35, 50]. Although the lesion responsible for dysynchrony may be within the inner hair cells (presynaptic) or neural elements (postsynaptic), it appears that, in some children, the ANSD phenotype may result from an absent or hypoplastic CN, especially when there are no medical or familial risk factors identified [35].
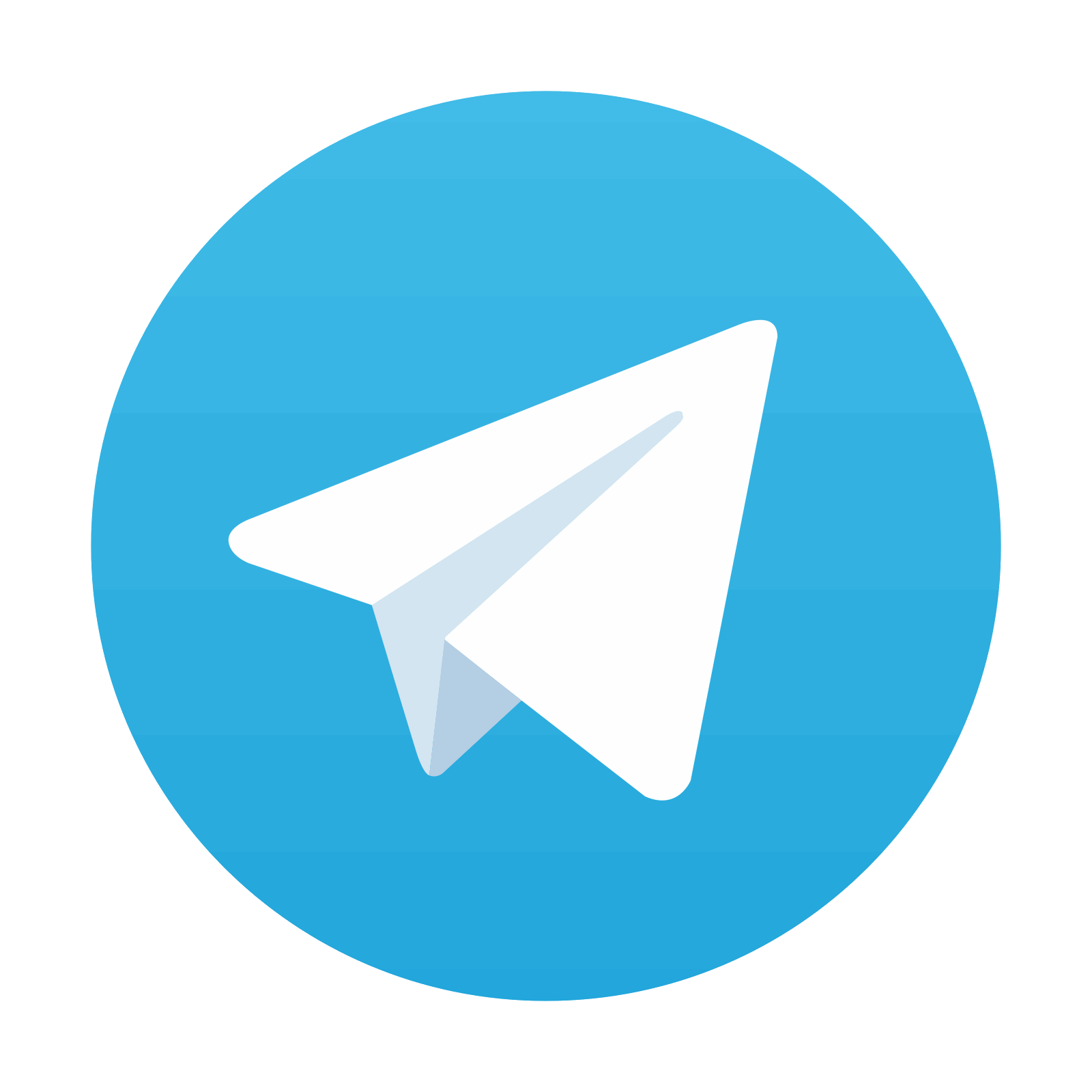
Stay updated, free articles. Join our Telegram channel

Full access? Get Clinical Tree
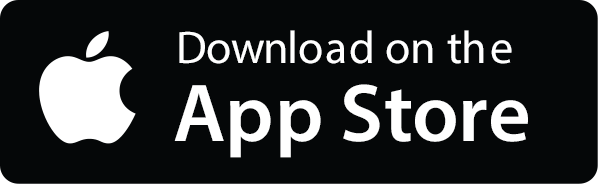
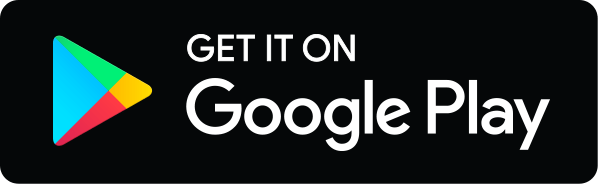