Electrical Signals of the Retina and Visual Cortex
Neal S. Peachey
Vision begins when the retina converts light into a neural signal. This process begins in the rod and cone photoreceptors, which are specialized for detecting light. Each of the four major photoreceptors in the human eye (rods, red cones, green cones, blue cones) are tuned to capture light within a specific range of wavelength. The subsequent extraction of visual information, such as the location of edges and the identification of objects, is accomplished through neural processing in the postreceptoral retina and the visual cortex.
Due to the anatomic organization of the visual system, it is possible to record field potentials generated by different retinal cell types and by activity in the visual cortex. These potentials provide practical tools for vision research and clinical diagnosis.
Retinal Neurons and Their General Organization
Figure 1 shows the appearance of a normal retina as viewed through the pupil by using an ophthalmoscope. The retinal area with the highest acuity vision is called the fovea; the foveal region is distinguished by a higher concentration of macular pigments. The retinal blood vessels enter and exit the retina at the optic nerve. The peripheral section shows that the retina is composed of millions of neurons organized into cellular layers, containing primarily cell bodies, and plexiform layers, where the majority of synaptic connections are made. In most regions of the retina, three distinct cellular layers can be identified. The outer nuclear layer (ONL) contains cell bodies primarily of photoreceptor cells and also of horizontal cells; the inner nuclear layer (INL) contains cell bodies of bipolar and amacrine cells; and the ganglion cell layer (GCL) contains cell bodies of ganglion cells as well as of displaced amacrine cells. Connections between these neural layers are made by processes that lie in the outer plexiform layer (OPL) and the inner plexiform layer (IPL). The retinal pigment epithelium (RPE) is a single layer of contiguous cells lying beneath the photoreceptors that lies at the back of the eye.
The first stage of vision occurs when light is captured in the photoreceptor outer segment, a specialized compartment in which disk-shaped membranes are densely packed. In rods, disks are disconnected from the plasma membrane, while cone outer segment disks are connected to the plasma membrane. Each disk contain a very high concentration of visual pigment molecules as well as proteins involved in phototransduction, the deactivation of phototransduction, or maintaining the structural properties of the outer segment. In darkness, the rod visual pigment rhodopsin is linked to the chromophore 11-cis retinal to form a stable complex. Light activation of rhodopsin causes the chromophore to isomerize to the all-trans configuration and to dissociate from rhodopsin. Photoactivated rhodopsin (R*) then activates transducin, which in turn activates phosphodiesterase to hydrolyze cyclic guanosine monophosphate (cGMP). Cation channels along the outer segment membrane are held in the open state by cGMP and close in response to light. Because a Na+/Ca++K+ exchanger in the outer segment and a Na+-K+ATPase in the inner segment balance cation influx through the cGMP-gated-channels, the net result is that photoreceptors are partially depolarized in darkness and hyperpolarize in response to light. In terms of neurotransmitter release, glutamate release from rod and cone photoreceptors is maximal in darkness and is decreased in response to light.
The primary retinal pathways by which visual information is transmitted centrally are diagrammed in Figure 2. Changes in glutamate release are sensed by two general classes of bipolar cells, distinguished by the polarity of their response to light. As the names imply, the membrane potential of depolarizing bipolar cells (DBCs) depolarizes in response to light, while hyperpolarizing bipolar cells (HBCs) hyperpolarize during the presentation of a light stimulus. While cone photoreceptors synapse on both classes of DBCs and HBCs, rods synapse directly onto only a single class of rod DBCs. Bipolar cell responses of opposite polarity are mediated by the different glutamate receptors used by DBCs (metabotropic or sign inverting) and HBCs (ionotropic or sign conserving). In addition to the different response polarity, the terminals of DBCs and HBCs lie in different sublamina of the IPL. In the cone pathway, visual signals are transmitted from cones to cone DBCs/HBCs to ganglion cells. The rod pathway is less direct, as rod DBCs synapse onto AII amacrine cells, which in turn synapse onto ganglion cells. The AII amacrine cell receives inputs from the many other classes of amacrine cell present in the retina to collectively control signal transmission through the rod pathway. Note that despite the early independence of rod and cone retinal circuits, these pathways converge at the ganglion cell level. Ganglion cells may be distinguished by whether a light stimulus presented to their receptive field center, which is encircled by a receptive field surround of opposite polarity, increases (on center) or decreases (off center) the cells’ firing rate. Ganglion cells may also be color opponent, and their output to the central visual pathway provides fundamental building blocks for analyzing the visual world.
The central area or fovea of the primate retina is specialized for high-acuity vision. As shown in Figure 1, the center of the fovea contains only cones, which are packed in a tight hexagonal arrangement so that the receptor density is maximal. Each foveal cone synapses onto a single DBC and HBC, preserving the fidelity of the spatially organized signal. The cuplike appearance of the fovea is caused by these second-order neurons, and the third-order neurons that they connect to, being pushed to the parafoveal region. This organization and the lack of foveal blood vessels ensure that the fovea detects the sharpest optical image with the highest receptor density and that this high-acuity signal is not degraded as it is transmitted centrally. In fact, the fovea is represented disproportionately in the cortical projection. In comparison, the peripheral retina is specialized for high sensitivity. The peripheral retina is characterized by a dense packing of rod photoreceptors, which, in comparison to cones, possess a higher overall sensitivity to light. This sensitivity is augmented by the fact that many rods synapse onto a single-rod DBC. The net result is that the rod pathway allows the primate retina to detect extremely dim stimuli at the expense of spatial information, while the cone pathway provides a high-acuity output at the expense of overall sensitivity to light.
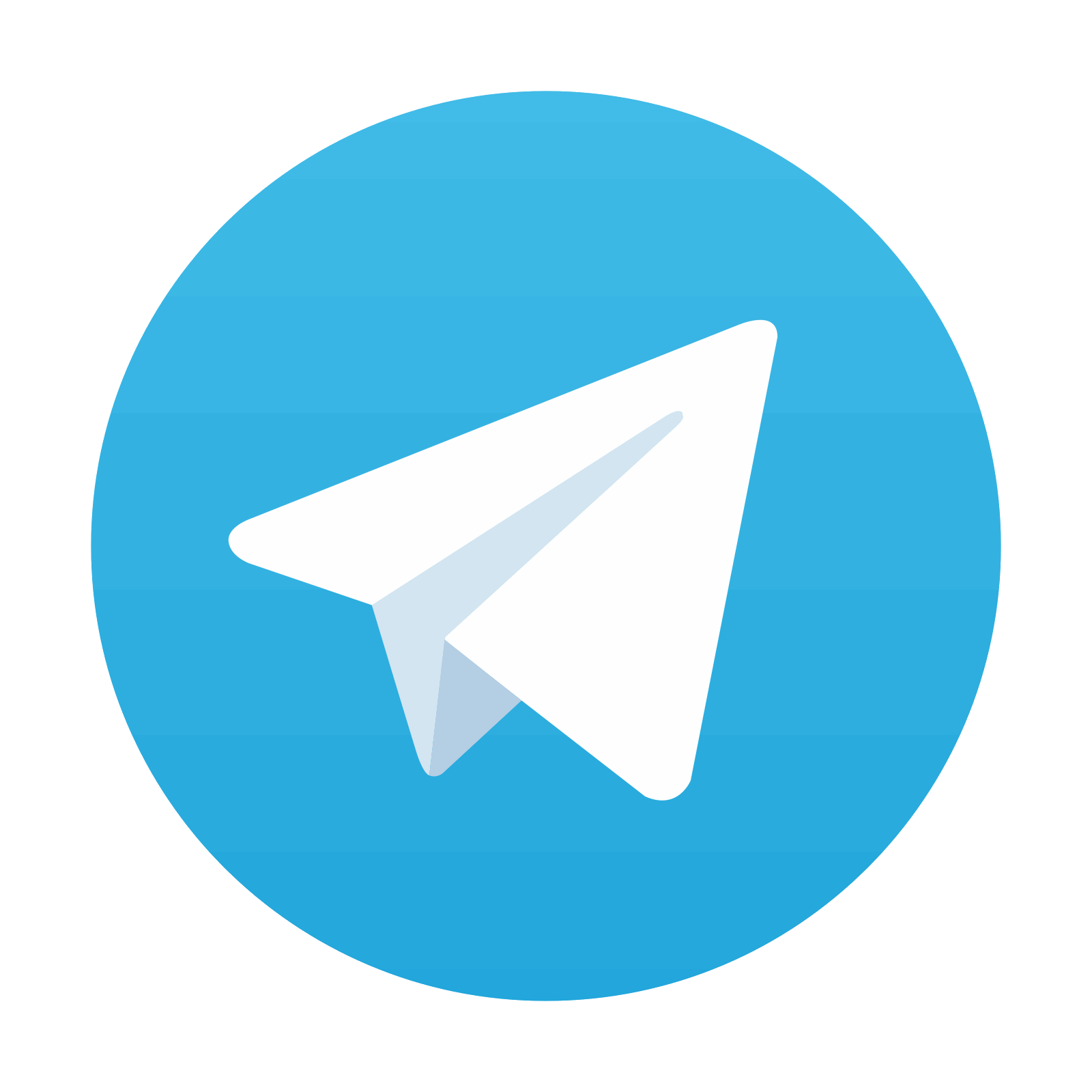
Stay updated, free articles. Join our Telegram channel

Full access? Get Clinical Tree
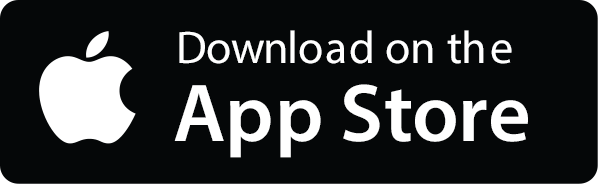
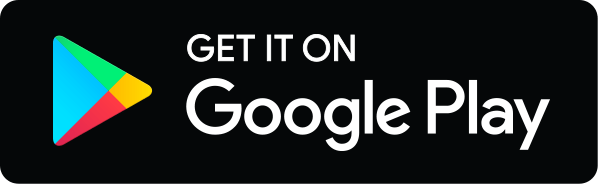