Fig. 13.1
Following SNHL, the most dominant TFS response component was below the estimated cochlear CF (arrow in top left panel, Liberman 1984). (a–d) Normalized power spectra of difcor functions. (e, f) Same for sumcor functions. Each row represents a rank and F0 condition
To quantify the mismatch between the frequency of the most dominant TFS component and the estimated cochlear CF, the ratio of these two quantities was computed for every fiber. For noise-exposed fibers, the ratio was lower than 1 on average for low harmonic ranks (N = 2 or 4), and it slowly approached 1 as N increased toward 20 (Fig. 13.2). These results provide further evidence that following SNHL, there is a mismatch between the CF of a fiber and the frequency of the TFS component that is most strongly represented in the ISIs of this fiber; this mismatch is most marked for fibers responding to partially resolved components. In contrast, for normal fibers, the ratio was always around one, indicating a good match between cochlear CF and the most dominant TFS response component for all harmonic-rank conditions.
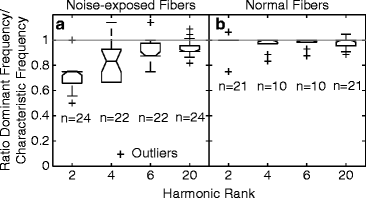
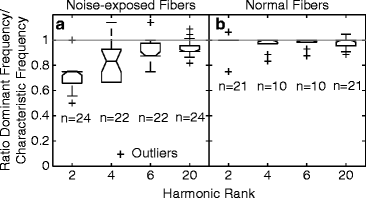
Fig. 13.2
Ratio of the most dominant TFS response component to cochlear CF for a population of normal and noise-exposed fibers. (a) Noise-exposed fibers. (b) Normal fibers. Number of fibers (n) that went into the computation of median and interquartile ranges is shown below each box-whisker plot. Outliers are indicated by “+”
Figure 13.3a, b shows the correlation coefficients, ρ TFS and ρ ENV , as a function of the F0 difference (ΔF0) between REF and TEST stimuli. Although both coefficients decreased as ΔF0 increased, for ρ ENV , the decrease became less and less steep as N increased. The latter effect can be explained by considering that for a given CF, the F0 was lower for N = 20 than for N = 2 and hence the ΔF0 in Hz was lower for N = 20 than for N = 2. Consistently across the population of normal and impaired fibers, ρ ENV corresponding to a frequency shift of 0.5 % (of F0) was higher for N = 20 than for N = 2 (Fig. 13.3c, d). Further analysis of the data showed that a given ΔF in Hz produced equivalent changes in ρ TFS and ρ ENV . These results suggest that based on within-fiber spike-timing information, TFS and ENV cues for the discrimination of frequency shifts in Hz are equally strong. Figure 13.3e, f shows that for both fiber populations, the ρ TFS value corresponding to a ΔF0 of 0.5 % was similar for N = 2 and N = 20.
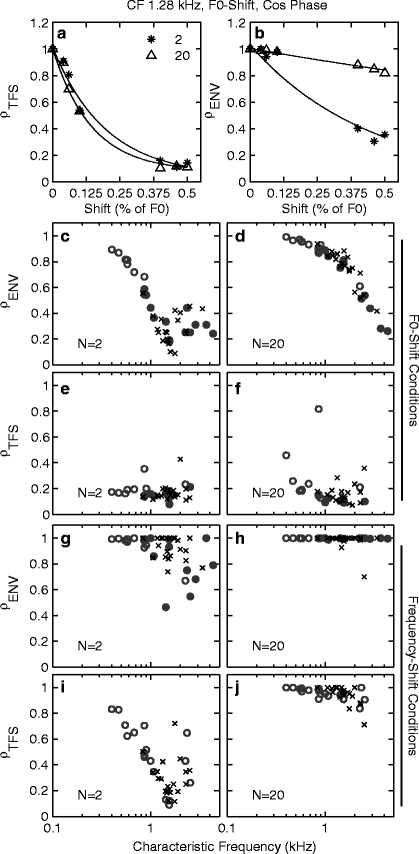
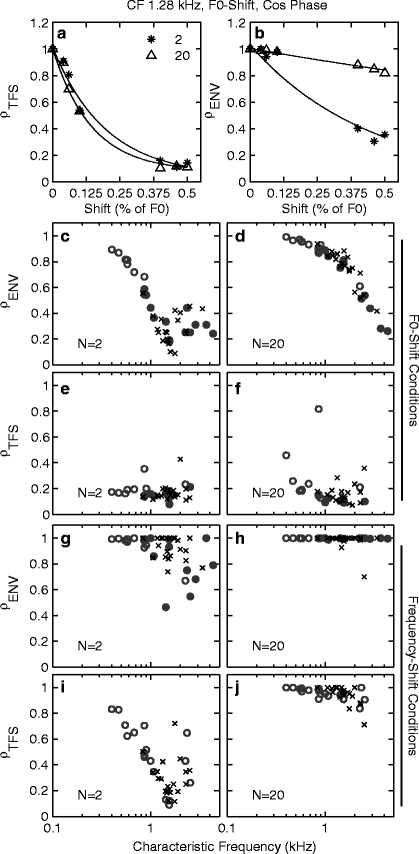
Fig. 13.3
ρ TFS (a) and ρ ENV (b) as a function of ΔF0 (in % of F0) are shown for a normal fiber. ρ TFS and ρ ENV corresponding to ΔF0 of 0.5 % are shown for the populations of normal (x) and impaired (o) fibers for F0 discrimination (c–f) and H–I discrimination (g–j). Filled circles are fibers with significantly broadened tuning
For H–I discrimination, ρ ENV was saturated near 1 (Fig. 13.3g, h). The latter result is consistent with the fact that the temporal envelope of a sound is unaffected by a coherent frequency shift of all the components in the sound, and it demonstrates that this was also the case after cochlear filtering—at least for small frequency shifts, for the cosine phase condition, and for the stimuli and fibers considered here. Overall, the ρ metrics across the NH and HI fiber populations were quite similar, suggesting that the ability of AN fibers to encode F0 or frequency shifts in the timing of their discharges (phase locking to the TFS or the ENV) is not affected by SNHL.
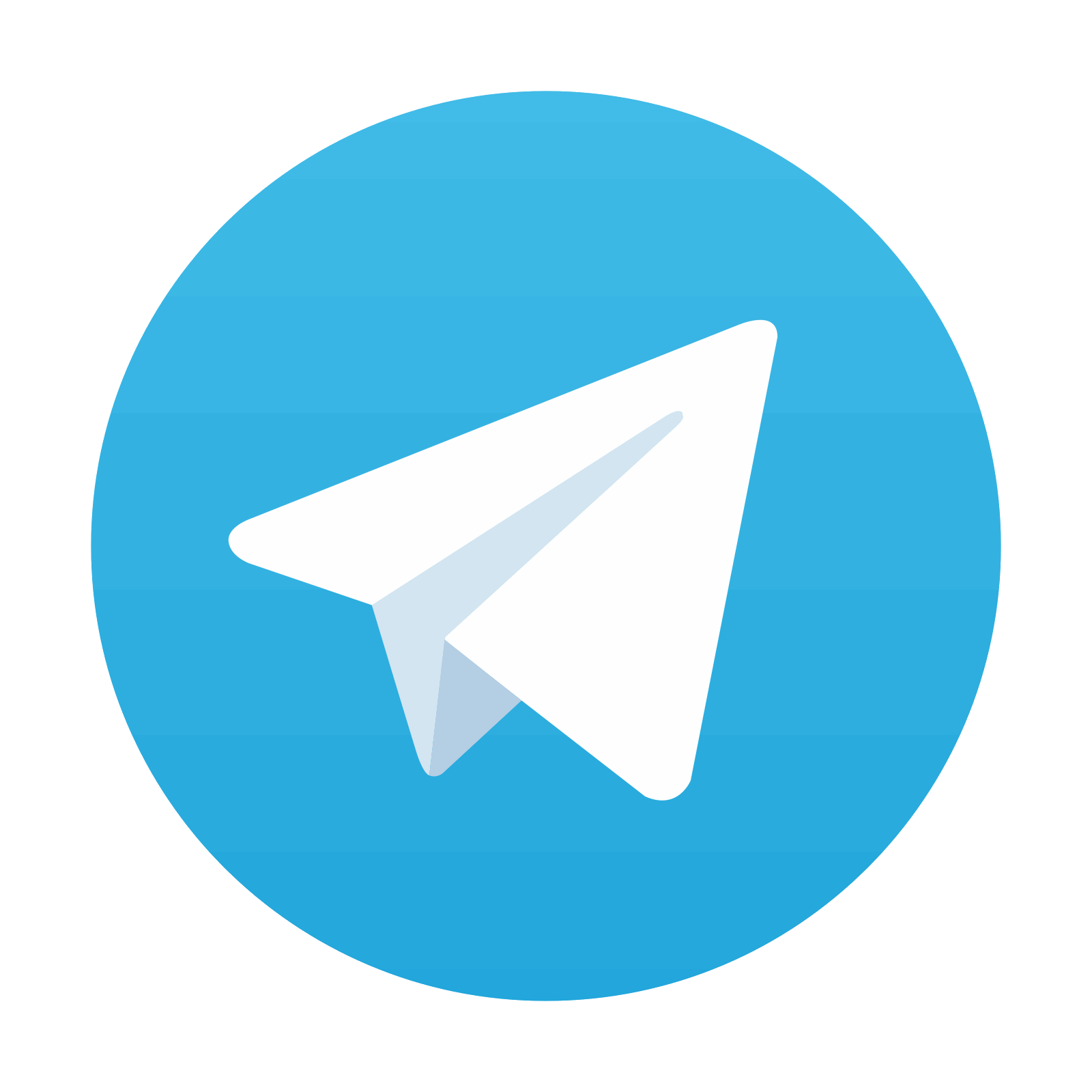
Stay updated, free articles. Join our Telegram channel

Full access? Get Clinical Tree
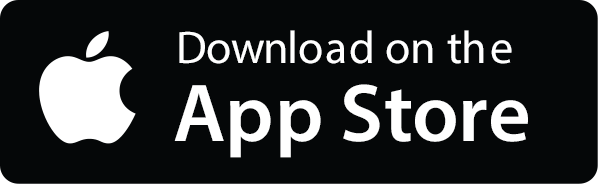
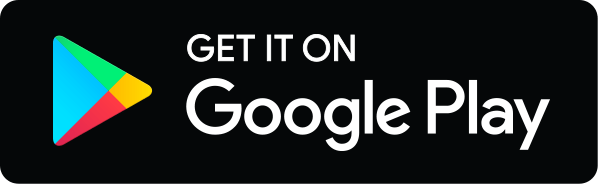