Fig. 13.1
Cross-sectional SD-OCT B-scans through the fovea of a 61-year-old man (right eye) with intermediate AMD followed for over 2 years. (a) Baseline visit shows a large drusenoid retinal pigment epithelial detachment (PED) with signs predictive for pending atrophy such as hyper-reflective foci (arrow) and hyper-transmissions within the PED (arrowhead), presumably due to RPE breakdown. (b) After 1 year the large drusenoid PED has partially collapsed with an adjacent area of outer retinal subsidence (arrow), a sign for nascent geographic atrophy (nGA). (c) Two years after baseline the PED has completely collapsed, leaving drusen-associated atrophy with the characteristic hyper-transmission into the choroid (area between arrows) resulting from loss of the RPE, photoreceptor, and choriocapillaris
The algorithm developed at the Bascom Palmer Eye Institute (BPEI) for SD-OCT imaging (Cirrus, Carl Zeiss Meditec, Dublin, CA) defines typical drusen as accumulations of material below the RPE, which leads to an elevation of the overlying RPE (Gregori et al. 2011). Once drusen lead to an RPE elevation above a minimal threshold, SD-OCT can provide reliable volume measurements (Gregori et al. 2011; Gregori et al. 2014). This algorithm can therefore distinguish between typical drusen, which elevate the RPE, and subretinal drusenoid deposits (SDD), which are located above the RPE and underneath the retina (Curcio et al 2013; Zweifel et al. 2010). Due to the selected threshold, this SD-OCT algorithm does not include small, shallow drusen or SDDs, which might be apparent on color fundus imaging. This could explain why there was only fair agreement between drusen area measurements obtained from color fundus photos and the SD-OCT algorithm, with the SD-OCT algorithm missing the small, flat drusen resulting in smaller drusen area measurements compared with manual color fundus measurements (Yehoshua et al. 2013). However, this SD-OCT algorithm does reliably measure the more clinically meaningful medium and large drusen, which are widely acknowledged risk factors for disease progression. Moreover, when comparing the change in drusen area assessed by SD-OCT and color fundus photos longitudinally, the two image modalities showed a good correlation. This suggests that both imaging modalities essentially captured the same changes. SD-OCT imaging has the added advantage that it can measure changes in drusen volume, which is a far more sensitive technique for measuring changes in drusen size compared with area measurements. For example, drusen volume can decrease by more than 50 % of the cube root volume without any apparent change in drusen area on the corresponding color fundus photo (Yehoshua et al. 2011b). The reason why drusen volume was found to be a more sensitive indicator of drusen growth compared with area measurements is because area measurements tended to plateau, while drusen volume continued to increase over time. Based on these findings, SD-OCT imaging is currently the most reliable strategy for following drusen morphology and progression over time and has replaced color fundus imaging for clinical trials.
13.4 SD-OCT Algorithms to Quantify Drusen Load
Different research groups have developed SD-OCT algorithms for quantifying drusen in the macula and monitoring their morphological changes over time (Chen et al. 2014; Folgar et al. 2016; Gregori et al. 2011; Schlanitz et al. 2015). The algorithm developed at BPEI is fully automated, FDA approved, and commercially available on the Cirrus SD-OCT instrument (Carl Zeiss Meditec Inc, Dublin, CA). This algorithm calculates the difference between the elevation of the RPE (caused by drusen) and a virtual RPE floor free of deformations (called the RPE fit) (Gregori et al. 2011). The difference between the RPE and the RPE fit results in reproducible measurements of drusen area and volume (Gregori et al. 2011, 2014) (Fig. 13.2).


Fig. 13.2
SD-OCT imaging of small- to medium-sized drusen. (a) Color fundus photo with a superimposed SD-OCT dataset indicated by the blue square. (b) Two cross-sectional SD-OCT B-scans correspond to the retinal locations marked by white lines on the color fundus photo. The segmentation of the RPE is shown in red, and the RPE floor (RPE fit = free of any deformations) is shown in yellow. (c) Surface rendering of the RPE segmentation. (d) Retinal thickness map. (e) Surface rendering of the RPE floor. (f) Drusen thickness map (Figure, courtesy of Giovanni Gregori, Gregori G et al. 2011)
The minimum detectable RPE elevation was chosen to be 19 μm (10 pixels) to minimize the contribution of noise that accompanies any measurement technique. A significant deviation from the RPE fit would therefore be any RPE elevation greater than 19 μm, which would subsequently be counted as a druse. The preferred pattern for measuring drusen is the 200 × 200 scan pattern (200 A-scans per B-scan and 200 B-scans in each cube), which consists of 40,000 A-scans with a uniform spacing of 30 μm between each A-scan and each B-scan. This scan pattern covers a 6 mm × 6 mm area centered on the fovea.
Another SD-OCT algorithm for drusen segmentation was developed by a research group at Duke University (Chiu et al. 2012; Jain et al. 2010). This algorithm segments the retina into three retinal boundaries: inner aspect of the inner limiting membrane (ILM), inner aspect of the RPE-drusen complex, and outer aspect of Bruch’s membrane. The spacing between adjacent B-scans was 66 μm, which might explain why this algorithm had difficulty detecting small drusen. Any hyper-reflective material contiguous with the RPE, such as SDDs, is segmented and included into the analysis as well, which is a unique feature of this algorithm. This algorithm segments at the inner border of the RPE band rather than the outer border and can therefore capture diffuse RPE thickening even before drusen form. However, this algorithm was designed for the Bioptigen system and is not currently commercially available.
Another drusen segmentation strategy is based on polarization-sensitive OCT (PS-OCT), which segments the RPE based on its depolarizing properties and allows quantitative drusen area and volume assessment (Baumann et al. 2010; Gotzinger et al. 2008; Sugita et al. 2013). After the actual position of the RPE is located based on its polarization uniformity (DOPU) value, drusen are segmented by calculating the difference between the actual RPE position and a virtual RPE position free of any deformations. However, this segmentation strategy is experimental and not widely available at this time.
The performance characteristics of commercially available algorithms for drusen detection were compared between three different SD-OCT instruments. Drusen segmentation results were compared between the Cirrus instrument (Carl Zeiss Meditec, Dublin, CA), the 3D OCT-1000 device (Topcon, Tokyo, Japan), and the Spectralis instrument (Heidelberg Engineering, GmbH, Heidelberg, Germany) (Schlanitz et al. 2010). Overall, the Cirrus drusen segmentation algorithm made significantly fewer errors in drusen detection than the 3D OCT-1000 instrument (p < 0.001). Due to the fact that the Spectralis instrument does not offer a true RPE segmentation, it performed poorly.
13.5 Following Drusen Growth with SD-OCT Imaging
Population-based trials studied the natural history of drusen to determine risk factors for disease progression (Chew et al. 2014; Cukras et al. 2010; Klein et al. 2007; Sparrow et al. 1997). Drusen size and number, extent of pigmentary abnormalities, and extent of involvement in one or both eyes are known risk factors for the development of late AMD (Ferris et al. 2013). Furthermore, the underlying genetics with the presence or absence of at-risk and/or protective alleles influences the phenotype of AMD, as well as its progression. For example, the COMPLETE study showed a significant association between the number of at-risk alleles in complement factor H (CFH; rs1061170) carried by a patient and the increase in drusen volume over 6 and 12 months (p < 0.001) (de Amorim Garcia Filho et al. 2014). In another study, a significant association was found between the area and volume of macular drusen in an Amish AMD population and the number of at-risk CFH alleles (CFH; rs12038333) carried by these patients (Chavali et al. 2015).
In addition to being able to measure drusen volume, SD-OCT imaging has revealed that drusen are not stable structures and far more dynamic than originally appreciated using color fundus imaging. Drusen undulate over time and undergo repeated cycles of growth and shrinkage, with growth more likely than shrinkage (Yehoshua et al. 2011b), and the risk of progression to either GA or MNV increases as drusen enlarge (Chew et al. 2014; Cukras et al. 2010; Ferris et al. 2013; Schlanitz et al. 2015; Yehoshua et al. 2011b). Drusen can also spontaneously regress without leaving any traces (Bressler et al. 1995; Klein et al. 2007; Sparrow et al. 1997), which would be the most desirable outcome in a clinical trial (Fig. 13.3).


Fig. 13.3
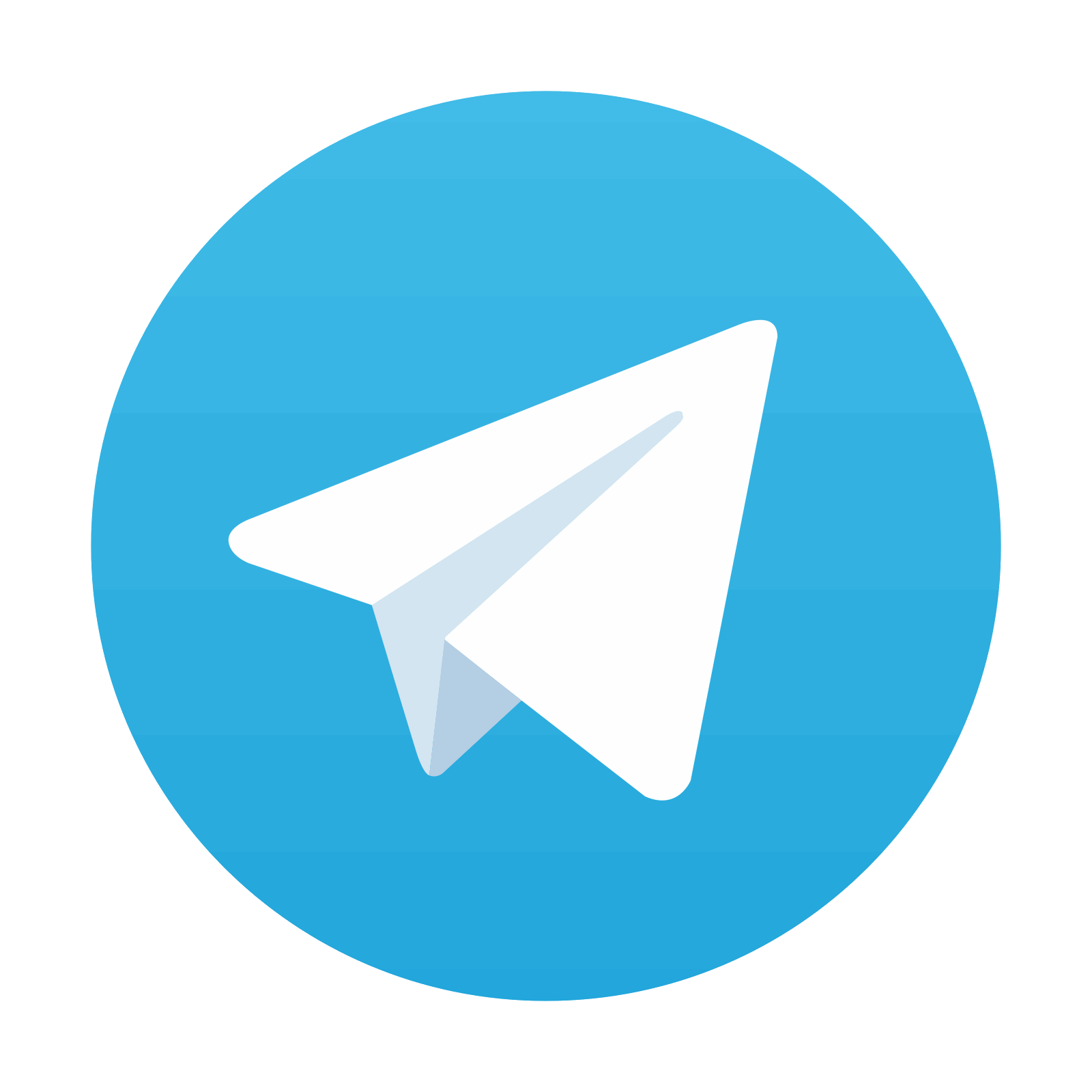
Examples of how drusen can change over time with different outcomes such as GA, MNV, or regression without any sequelae. Panel (a) 76-year-old woman (right eye) with drusen at baseline and developed drusen associated atrophy over 12 months. Color fundus photos with a white line representing the location of the OCT B-scans (A, F), horizontal cross-sectional B-scans (B, G), RPE segmentation maps (C, H), hybrid map made of OCT fundus image and drusen thickness map (D, I), and fundus autofluorescence (E, J). At 12 months, GA appears with an area of 1.28 mm2. Images are shown at baseline (A–E) and 12 months follow-up (F–J). Panel (b). A 64-year-old woman (left eye) with drusen at baseline and developed macular neovascularization (MNV) over 15 months. Drusen were found to increase in volume and then regress, eventually leading to MNV. Color fundus photos with a white line representing the location of the OCT B-scans (A, E, I), horizontal B-scans (B, F, J), RPE segmentation maps (C, G, K), hybrid map made of OCT fundus image, and drusen thickness map (D, H, L). Images are shown at baseline (A–D), at 6 months (E–H), and at 15 months of follow-up (I–L). Baseline drusen volume was 0.19 mm3 (D) and 0.23 mm3 at 6 months (H). Panel (c). A 64-year-old woman (right eye) with drusen at baseline and spontaneous drusen regression over 6 months without progressing to late AMD (MNV and/or GA). Color fundus photos with a white line representing the location of the OCT B-scans (A, E, I), horizontal B-scans (B, F, J), RPE segmentation maps (C, G, K), hybrid map made of OCT fundus image, and drusen thickness map (D, H, L). Images are shown at baseline (A–D), at 3 months (E–H), and 6 months follow-up (I–L). Baseline drusen volume was 0.21 mm3 (D), 0.26 mm3 at 3 months (H), and 0.001 mm3 at 6 months follow-up (L). (Figure courtesy of Zohar Yehoshua, modified from Yehoshua Z et al. 2011b)
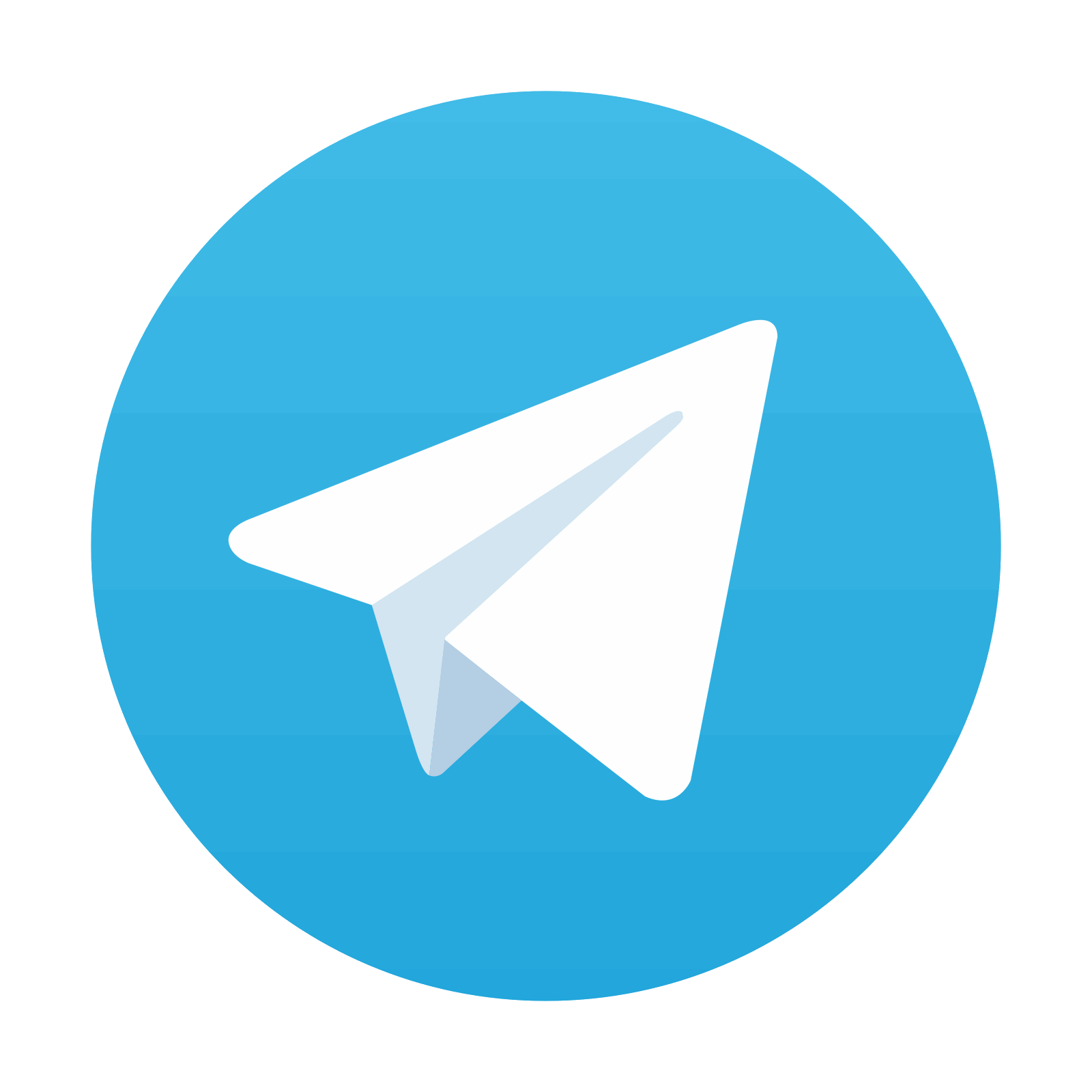
Stay updated, free articles. Join our Telegram channel

Full access? Get Clinical Tree
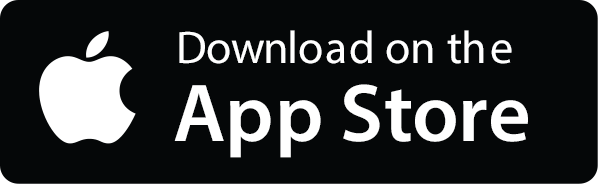
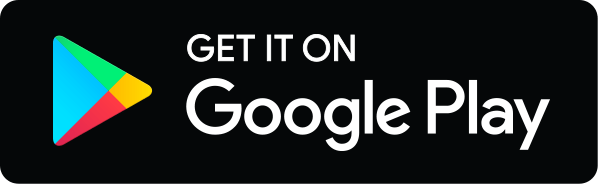
