, Maneli Mozaffarieh1 and Hans Bebie2
(1)
Department of Ophthalmology, University of Basel, Basel, Switzerland
(2)
Institute for Theoretical Physics, University of Bern, Bern, Switzerland
Abstract
Deoxyribonucleic acid (DNA) can be compared with a CD-ROM compact disc. This means that information is stored that can be repeatedly read without changing the information. The DNA codes the information that finally guides the metabolism and structure of cells. Let’s explain this with an analogy.
14.1 DNA as the Hard Disk of the Cell
Deoxyribonucleic acid (DNA) can be compared with a CD-ROM compact disc. This means that information is stored that can be repeatedly read without changing the information. The DNA codes the information that finally guides the metabolism and structure of cells. Let’s explain this with an analogy.
In your clinic, you have a library with a number of books. Within these books, you’re searching for one with a description of a particular operation. Since the book is valuable, you cannot take it out of the library. Instead, you make a copy of the book and intend to take this copy to the operating theater. The individual chapters in the books correspond to the genes written in DNA, whereas the copies correspond to the RNA. Fortunately, you have a photocopy machine in your library. The photocopy machine corresponds to the transcriptional machinery. As you have only copied individual chapters, these copies are smaller than the books and can be carried easily. Likewise, the mRNAs are much shorter than the DNA. Correspondingly, the mRNA moves out of the nucleus to the ribosomes. With the help of this information, you carry out your operation. This corresponds to the translation of RNA to one protein.
14.2 Discovery of DNA
Since early times, man has witnessed how children inherit characteristics from their parents. However, it was not until 1866 that the most important fundamental rules of genetic inheritance were recognized. Mendel1 conducted a series of breeding trials with plants and, thus, discovered classical genetics. Taking what he learned from pea plants, he demonstrated that the form and color of the peas were determined by “factors,” now known as genes. Mendel was completely correct in his assumption that these “factors” occur as pairs, i.e., one from the mother and one from the father.
The first step in the discovery of DNA was made in 1869 when it was first isolated by the Swiss scientist Miescher2 (Fig. 14.1). He was born in the city of Basel. His father was professor of anatomy and Miescher began studying medicine as well. However, after a typhus infection, he went partially deaf and feared that this handicap would limit his ability to be a successful physician. As a result, he switched his major to chemistry, where he began analyzing the pus from wounds. This led to the discovery of DNA as Miescher found a previously unknown substance within the white blood cells of the pus. The new substance was unique since it contained phosphate. All other known organic molecules were made from carbon, oxygen, nitrogen, and hydrogen. Miescher named the newly found substance “nuclein” since it came from the cell’s nucleus. A student of his, Altmann,3 renamed nuclein “nucleic acid” since it was an acid molecule. Miescher later extracted DNA from many other cells, particularly from salmon sperm and oocytes (Fig. 14.2). At the time, however, it was not clear whether this “nucleic acid” was the molecule of heredity.
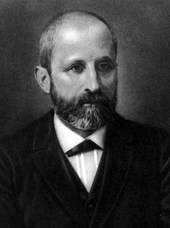
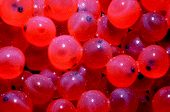
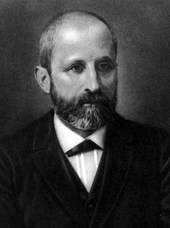
Fig. 14.1
Friedrich Miescher
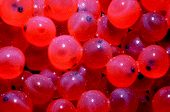
Fig. 14.2
Salmon oocytes. Miescher first extracted DNA from these types of oocytes
A breakthrough came about in 1928 when an army medical officer named Griffith4 was trying to find a vaccine against Streptococcus pneumoniae. Griffith was experimenting with bacteria and mice. During his experiments, he made the interesting observation that characteristics of bacteria, even after the bacteria were killed, could still be transferred to other bacteria. This told him that certain molecules remained intact even after death and that they were capable of storing genetic information. However, it was not clear whether these molecules were proteins or other molecules.
Later, in 1944, Avery5 was able to show that, indeed, DNA, not protein, was the hereditary molecule. Avery showed that he could transfer the information from cells after proteins had been digested but that, when DNA was digested, the hereditary transformation was blocked.
In 1950, Chargaff,6 an Austrian biochemist who later became an American citizen, found that the amounts of adenine and thymine in DNA were roughly the same, as were the amounts of cytosine and guanine. Wilkins7 and Franklin8 applied the X-ray diffraction technique to DNA and concluded that DNA must be a long molecule with a regular, repetitive skeleton. The method of X-ray diffraction is based on the following principle. The molecule of interest is first purified and then crystallized. When the X-ray penetrates the crystalline structure, the rays are diffracted (see Sect. 2.10). When this refracted light is projected on a film, it results in an interference pattern. From this pattern, the three-dimensional structure can be calculated.
Based on these types of X-ray diffraction images, in 1953, Watson9 and Crick10 were the first to propose a double helical structure for DNA (Fig. 14.3).
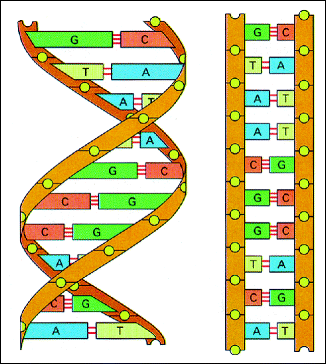
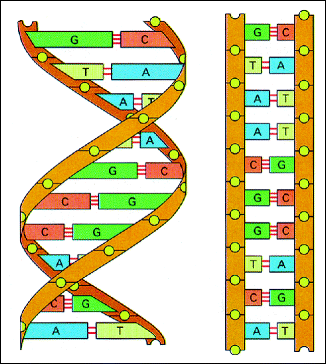
Fig. 14.3
Double helical structure of DNA. The structure of DNA is illustrated by a double helix (left) with about ten nucleotide pairs per helical turn. Each spiral strand, composed of a sugar phosphate backbone and attached bases, is connected to a complementary strand by hydrogen bonding (non-covalent) between paired bases, adenine (A) with thymine (T) and guanine (G) with cytosine (C) (right). (From Flammer J (2006) Glaucoma. Hogrefe&Huber, Bern. With permission)
14.3 Structure and Function of DNA
The two helical strands of the DNA molecule form a backbone. The DNA backbone is a polymer with an alternating sugar-phosphate sequence. The deoxyribose sugars are joined at both the 3’-hydroxyl and 5’-hydroxyl groups to phosphate groups in ester links, also known as “phosphodiester” bonds (Fig. 14.4).
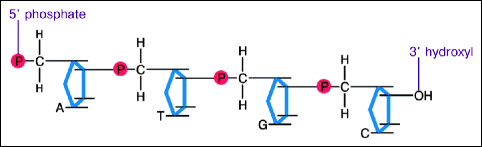
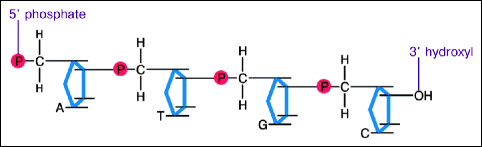
Fig. 14.4
DNA backbone. The basic structure of DNA can be divided into two parts: the external sugar-phosphate backbone and the internal bases. The backbone is constructed by alternating 2-deoxyribose sugar and phosphate molecules, which are highly polar. Because the backbone is polar, the molecule is hydrophilic
The human cell contains two types of DNA – nuclear and mitochondrial. The first constitutes the 23 pairs of chromosomes contained within the nuclei of human cells. Nuclear DNA is in the form of a double chain. The chains are complementarily arranged; i.e., there are fixed base pairings. Certain bases are always positioned across from each other. Thus, thymine is always across from adenine and cytosine is always across from guanine and vice versa, explaining the previously mentioned explanation of Chargaff. This permits a rapid doubling of the entire genetic information when a cell divides. When a double chain splits open, like a zipper, each chain is rapidly completed by a complementary chain. In humans, the entire genetic code is stored in the form of several billion base pairs that contain information for thousands of genes. Such an enormous amount of information is difficult to imagine. If one could lay out the DNA of a human being in a straight line and one base pair were placed every millimeter, then the entire length of the genetic information would stretch the distance between the East and West coasts of America. What is even more impressive is that every single cell in our body has the entire genetic information stored twice: once from the mother and once from the father. This is an amazing concentration of information, information that allows a human being, with all his/her physical, mental, and emotional qualities, to grow and develop from one fertilized egg.
< div class='tao-gold-member'>
Only gold members can continue reading. Log In or Register a > to continue
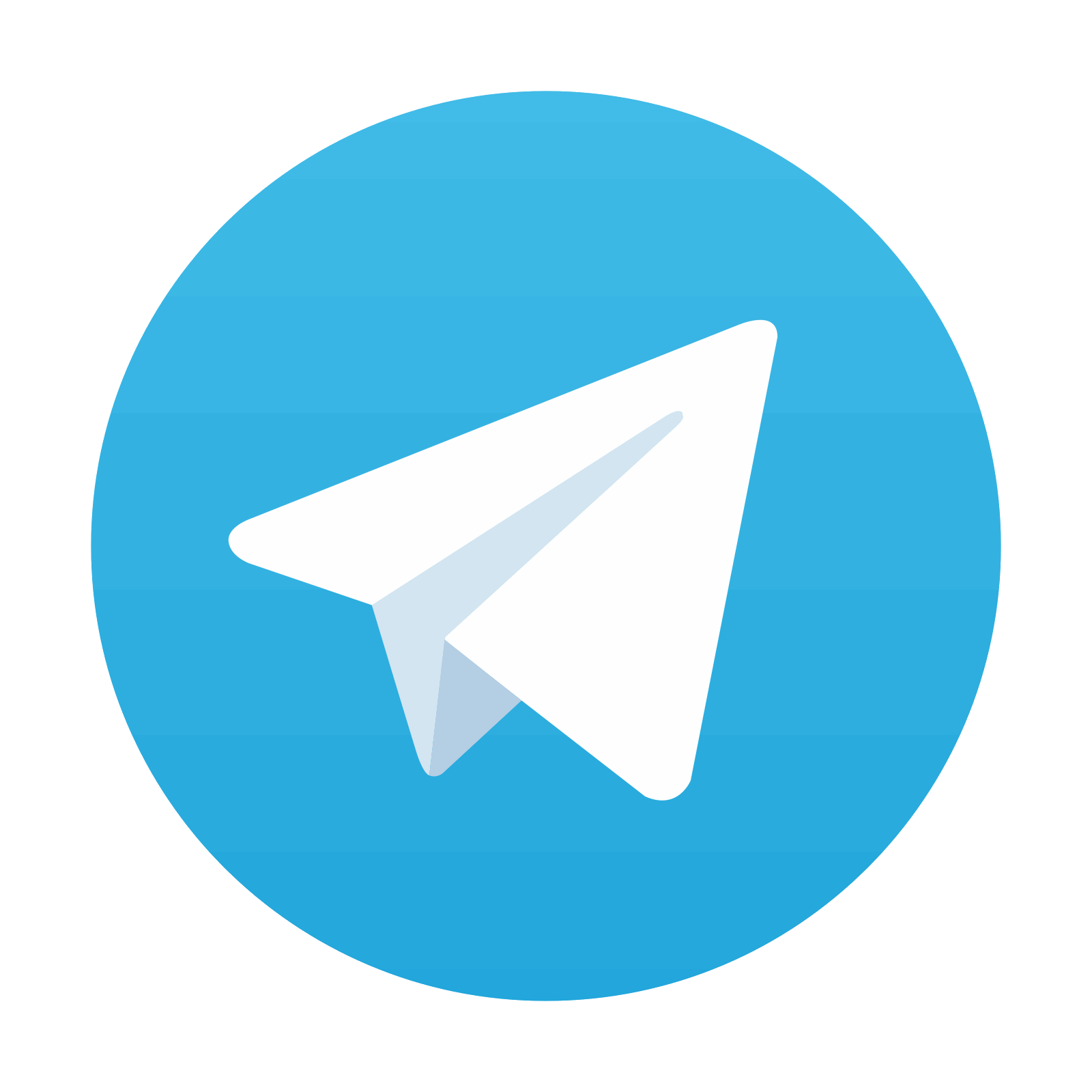
Stay updated, free articles. Join our Telegram channel

Full access? Get Clinical Tree
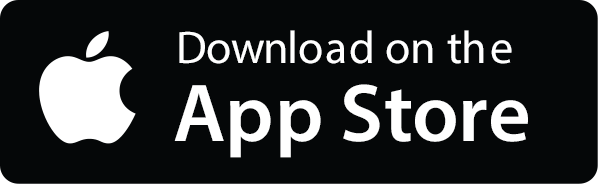
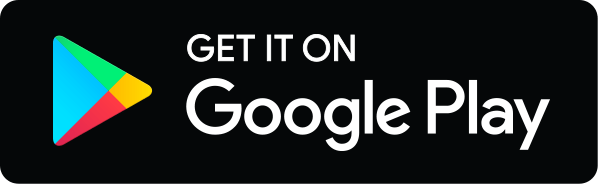