The topic of ocular tear film breakup has long been the subject of considerable attention due to its implications both in contact lens design and in the study of corneal dewetting. A better understanding of the causes of the breakup could enhance lens design and guide research toward improved dry-eye treatment options. The integrity of the pre-corneal tear film is vitally important both optically, in providing a smooth optical surface, and physiologically, in preventing corneal desiccation. The breakup time (BUT) of this film, clinically defined as the period between the last blink and the first instance of film breakup, averages 10 seconds in normal humans. Because this is greater or comparable to the average interblink period of 5–10 seconds, the tear film is normally sufficiently stable. Patients with dry eye, on the other hand, are characterized by a pre-corneal tear film that does not maintain integrity in the interblink period. In these patients, the breakup time may be as low as 1–2 seconds, and corneal dewetting thus occurs between blinks ( Box 16.1 ).
- •
Tear film is about 3–7 µm with lipid, aqueous, and mucous layers
- •
Small amount of tear volume is needed on the ocular surface
- •
Average 10 seconds of tear breakup may be sufficient for protecting ocular surface
Structure of tear film
The ocular tear film is composed of three layers. Contacting the corneal epithelium is a hydrophilic mucous layer approximately 0.02–0.05 µm thick. As the underlying cornea itself is extremely hydrophobic, this layer is believed to provide a wetting substrate for the aqueous layer above it. Lying on this layer is a relatively thick aqueous layer that comprises the bulk of the tear film. Between this layer and the air is a 0.1–0.2-µm thick lipid layer that retards tear evaporation.
Tear film thickness and tear volumes on the ocular surface
There has been no consensus on the thickness of human tear film for a long time due to the lack of direct visualization and measurement. Clearly, the thickness of the tear film and thinning rate hold the key to understanding the dynamics of the tear system in protecting the ocular surface. The indirect or invasive methods used to measure the layer resulted in thickness estimates from approximately 3 µm to 40 µm. The early measurements of tear film thickness (TFT) used invasive methods that potentially disturbed the tear film. Both Mishima and Benedetto et al measured fluorescence after instilling fluorescein and reported a TFT of 4 µm; however, the tear film might have been diluted by the saline-fluorescein instillation. Using confocal microscopy, Prydal et al reported thickness values of 41−46 µm. For rabbits, Mishima used fine glass filaments and fluorometry to measure a TFT thickness of 7 µm. This thickness has been widely cited in the literature, although whether the rabbit tear film differs from that in humans is unknown. By applying an absorbent paper disc to the cornea, human TFT was found to be approximately 8 µm, which could be an overestimate if reflex tears were generated and/or if the fluid was drawn from the tear film surrounding the disc or epithelium. Chen et al used an in vivo cryofixation method to examine the tear film in rat corneas. They found that the tear film varied from 2 to 6 µm in thickness on the corneal surface. Using a modified, noninvasive optical coherence tomography (OCT) instrument, Wang et al studied 80 human eyes and found the thickness of the tear film to be 3.3 µm, which is in agreement with King-Smith et al’s estimate of 3 µm determined by an interferometric method in vivo. In another study using a real-time anterior-segment OCT, Wang et al measured TFT and found the layer to be 3.4 µm.
The total tear volume is estimated over the exposed ocular surface and tear menisci to be about 2–4 µl, including the tear fluid volume of 1 µl. In another study using photography, tear volume in the lower tear meniscus was estimated at about 0.5 µl, which appears to be too low compared to others. The distribution is about equal in these three compartments. Although there are some exchanges and renewal, the system is balanced with a fixed amount in each compartment regardless of blinking. However, the system changes when extra tears are produced or added.
In the normal tear system, a small amount of tears such as 3 µl may be enough for the ocular surface to remain wet. This figure of basal tear volume is slightly lower than the values reported by Mishima et al using a fluorescein method. Each blink redistributes and mixes the tears and the lid movement facilitates the action of the drainage system by compressing the canaliculi and lacrimal sac to expel the tears from the system.
Tear film breakup mechanisms ( Box 16.2 )
The presence of mucins on the corneal surface renders the surface hydrophilic, i.e., the surface energy of mucin-covered cornea surface is lower if it is in contact with water compared to the surface energy if it is in contact with air. In this scenario, the thin tear film is stable and so not likely to break, except by mechanisms that draw fluid out of the tear film such as evaporation. A number of researchers have measured evaporation rates from tear films, and under normal circumstances, the measured values are significantly lower than the lacrimal secretion. However damage to the lipid layer can lead to a substantial increase in evaporation rates.
- •
There are many mechanisms of tear breakup
- •
The thin tear film is stable and not likely to break, except by mechanisms that draw fluid out of the tear film such as evaporation
- •
The presence of a mucin-covered hydrophilic surface makes tear breakup unlikely
- •
The breakup time may depend strongly on the initial tear film thickness
Since the presence of a mucin-covered hydrophilic surface makes tear breakup unlikely, some researchers have speculated that destruction of the mucin layer is perhaps the first step in the breakup. Holly and Lemp showed that the underlying corneal epithelium is extremely hydrophobic in the absence of coating mucus, but that mucus itself is highly wettable. In a series of papers, they proposed a mechanism in which lipids from the outer film layer diffuse rapidly through the aqueous layer and deposit on the mucous layer, converting it to a hydrophobic substrate. However, a theoretical analysis of the fluid flow in the tear film shows that this mechanism is physically inconsistent. Moreover the low solubility of lipids in tears makes the process of lipid deposition on the mucus unlikely.
Proposing an alternative mechanism, Lin and Brenner conducted a theoretical analysis of the film under the influence of van der Waals dispersion forces. They calculated the minimum stable film thickness for a number of retarded Hamaker constant values and reported that by this method a minimum constant of 10 −13 erg/cm would be necessary to break a 7-µm thick film. To rupture a 4-µm thick film in 50 seconds, their method requires the Hamaker constant to be about 3 × 10 −13 erg/cm. This constant, however, has been estimated to have a maximum value of about 10 −19 erg/cm, about 6 orders of magnitude smaller than that required by Lin and Brenner. This mechanism thus appears to be insufficient to produce film breakup under physiological conditions in a reasonable time span.
Sharma and Ruckenstein showed that the mechanism proposed by Lin and Brenner cannot produce breakup in a clinically consistent time span with a realistic Hamaker constant. As an alternative, they propose a double-layer, double-step mechanism for breakup. In their mechanism, they initially neglected the aqueous layer in order to show rapid breakup of the mucin layer. They performed a stability analysis on the mucus–cornea system and established that the mucous layer can break locally due to van der Waals interactions with the underlying corneal epithelium. They calculated that this breakup can occur within time periods consistent with clinical observation (4–50 seconds) with a Hamaker constant they believed to be reasonable for this system (10 −14 to 10 −13 erg for a mucous layer thickness of 200–500 Å). They then cited experimental evidence to support a near-spontaneous aqueous film rupture once the mucous layer has been broken. However, their own stability analysis shows that in order for this breakup to occur within a reasonable time span, the retarded Hamaker constant for the cornea–aqueous layer system must be about 3 × 10 −13 erg/cm, about six orders of magnitude larger than the estimated value of 10 −19 erg/cm. A further mechanism is thus still necessary to break up a micrometers-thick aqueous film over such a substrate. The BUT predicted by this mechanism depends strongly on the initial TFT. If the starting film thickness is reduced to a fraction of a micron, the breakup may occur in a reasonable time for physiological Hamaker constants.
Recently, Zhu and Chauhan have shown that an increase in evaporation rate or a decrease in tear production rate leads to thinner tear films. Also, a number of reports show a clear relationship between evaporation rates and dry eyes. So it may be argued that dry-eye sufferers have significantly thin tear films, which makes them susceptible to tear breakup. However, clinical studies also show that there is no significant difference between volumes of dry-eye sufferers and normal subjects. Furthermore, even if the thickness of the tear film in mathematical models is reduced to a fraction of a micron, breakup still does not occur in a few seconds, unless the Hamaker constant is artificially large. It should be noted that the TFT at the intersection of the meniscus and the tear film becomes very thin immediately after a blink. This thin region is commonly referred to as a “black line,” and it has been suggested that the tear film breakup is initiated in this region.
However, the tear breakup on dry-eye sufferers happens in other locations also, implying that the “black line” cannot be the main contributor to tear breakup. Most studies of thin film breakup have relied upon analysis of systems in which the tear film lies upon a uniform nonwetting surface. In reality, corneal surface is expected to be patchy with both physical and chemical heterogeneities. The chemical heterogeneities drive flow from the less wettable region to the more wettable region due to gradients in surface energies in a manner analogous to the Marangoni flow. The growth rates of the instabilities driven by surface energy gradients can be significantly larger than those for homogeneous surface and vary inversely as the potential difference (thickness or wettabilility differences) introduced by the heterogeneities. Several papers have investigated the instabilities driven by physical heterogeneities as well as a combination of physical and chemical heterogeneities. These studies listed above and others have demonstrated that both physical and chemical variations on a surface can have a significant impact on breakup of thin films. However, even after incorporating chemical and physical gradients, the value of Hamaker constant required to drive breakup in a few seconds is larger than 10 −17 J, which is an unreasonably large value.
A complete description of the mechanisms that can lead to tear film breakup in reasonable times with physiologically reasonable parameters is still lacking. While none of the theories are able to explain all observations related to tear breakup, each provides useful insight into the process, and it is likely that the actual breakup is caused by a combination of all the factors and mechanisms cited above.
Future directions on the mechanism
Other mechanisms that could lead to thinning of the tear film are local evaporation and fluid flow driven by Marangoni gradients created by variations in lipid concentrations. With each blink, the lipid layer is largely wiped off the aqueous layer and deposited at the terminal area of the blink. Immediately after the blink, the lipids redistribute themselves across the eye due to the action of surface tension gradients (the Marangoni effect). This redistribution will carry with it some of the aqueous layer itself, causing deformation and localized thinning and thickening of the film. After the film has thinned due to the Marangoni flow, the breakup could be caused by the mechanisms proposed above. This mechanism of thinning driven by Marangoni gradients has not been sufficiently explored in the context of tear film breakup due to lipid spreading. Some researchers have reported observing lipid aggregates in dry-eye sufferers and images of breakup suggest that the breakup is initiated around these aggregates. This observation reinforces the importance of Marangoni gradients because it is feasible that the lipid aggregates provide a continuous source of lipids to the surface immediately surrounding the aggregates. The higher concentration of the lipids will reduce the surface tension, driving fluid flow away from the aggregates and causing film thinning and potential breakup.
Another potential breakup mechanism that has not received much attention is the possibility of nonuniform mucin deposition leading to hydrophobic patches on the cornea. During the blink, the coating on the hydrophobic patches may not be as thick as on the remaining cornea. In fact it is feasible that microbubbles may become trapped on the hydrophobic patches of the cornea, leading to rapid breakup after eye opening. The hydrophobic patches may be redistributed during the blinks, leading to variations in the locations of the breakup from blink to blink.
While the detailed mechanism that leads to tear breakup is not completely understood, it is clear that the quality and quantity of tears are the most important variables that have an impact on tear film disruption and breakup. Issues related to dynamics and tear film regulation are discussed below.
Dynamic tear system with regulation
The tear system is a highly regulated dynamic system with an interaction between tears and blinking ( Box 16.3 ). The system includes tear secretion, distribution, drainage, absorption, and evaporation, with mostly a dynamic balance in healthy eyes. The dynamic balance is maintained with a certain amount of the tears on the ocular surface in three compartments: upper and lower tear menisci and precorneal tear film. The system is also kept balanced with tear renewal between fresh tears from the secretion and tear loss due to evaporation, drainage, and absorption. The constant tear renewal with high-quality fresh tears is essential to keep the ocular surface wet during the interblink period, so that sharp vision and ocular comfort are maintained. Each blink initiates a cycle of tear secretion, spreading and drainage of tears and evaporation, which happens immediately after eye opening. Any alterations in blinking might affect the tear distribution, secretion, and drainage, leading to changes in the protection of the ocular surface. McDonald and Brubaker demonstrated that upper and lower menisci draw fluid from the preocular tear film and swell with time after a blink. Slit-lamp photography and video meniscometry have also been used to measure the tear meniscus around the lower eyelid and changes in the dynamics were found.
- •
The tear system is a highly regulated dynamic system with the interaction between tears and blinking
- •
Constant tear renewal with high-quality fresh tears is essential to maintain the ocular surface wet during the interblink period
- •
Tear volume and distribution on the ocular surface alter by blinking
These techniques have the disadvantage of using visible light and/or introducing fluorescein dye into the eye, which may induce reflex tearing and alter the test results. Most of these studies reported the static values of various lower tear meniscus parameters some time after blinking. Using real-time OCT, Wang et al imaged the tear film and tear menisci around the upper and lower eyelids simultaneously for the first time. The changes in the tears impacted by blink were clearly visualized ( Figure 16.1 ). The device is a noncontact and noninvasive modality which has been validated with good repeatability for measuring the TFT and tear meniscus. Using this method, the changes of TFT impacted by blinking and eye opening can be studied ( Figure 16.2 ). The effect of blinking on tear menisci can also be tracked ( Figure 16.3 ). Tear volume was found to be altered by blinking and the tear distributions with outcomes (gain or loss) during normal blinking and delayed blinking were reported previously ( Figure 16.4 ).The relationship between TFT and tear menisci and the changes in tear dynamics impacted by the blink after the instillation of artificial tears were studied. The responses of the tear system to different artificial tears were also investigated, and different dynamic responses to different artificial tears were found.

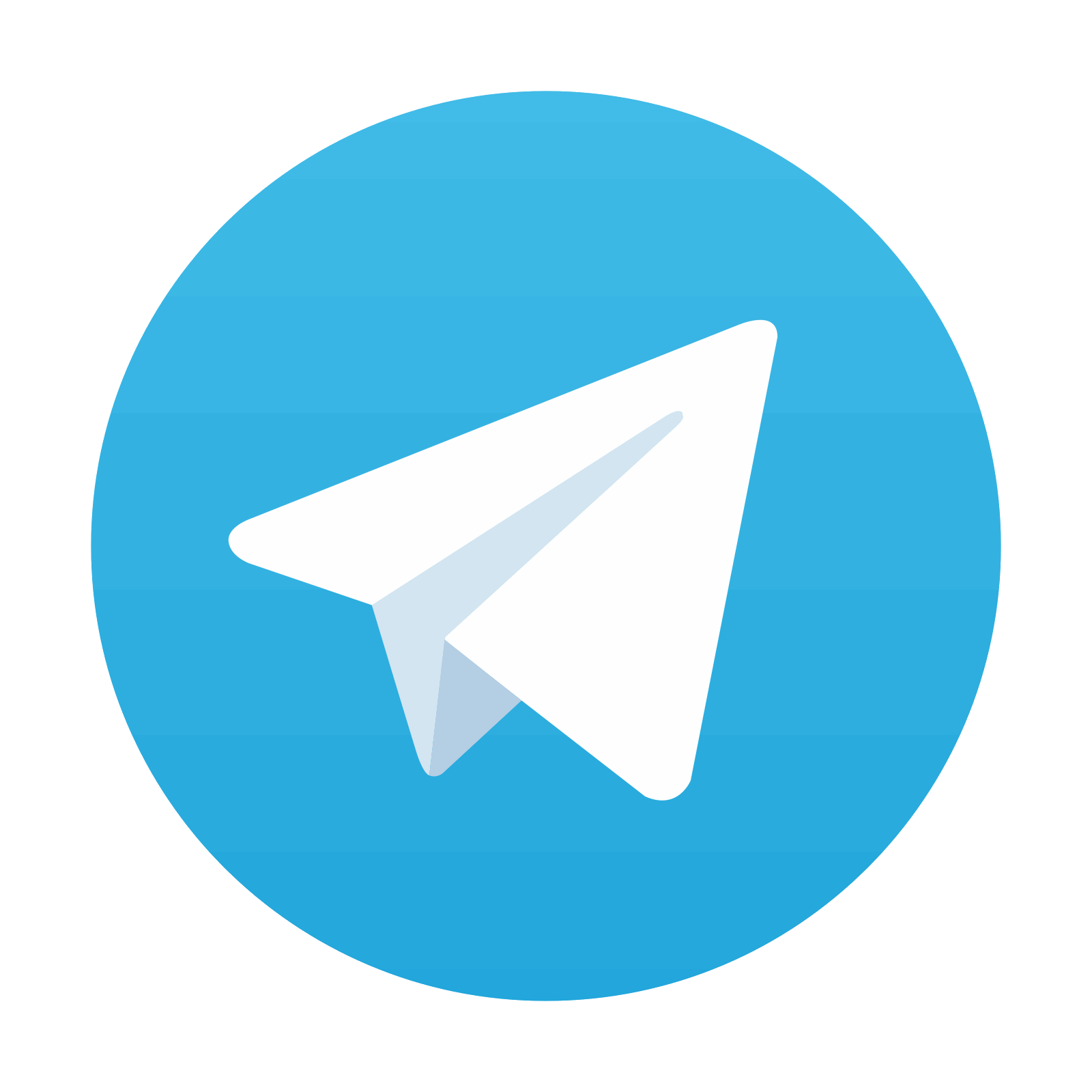
Stay updated, free articles. Join our Telegram channel

Full access? Get Clinical Tree
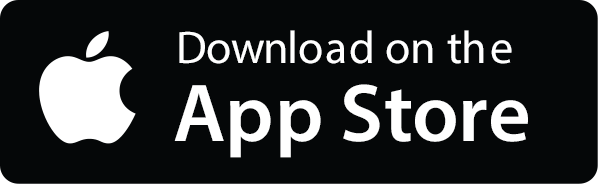
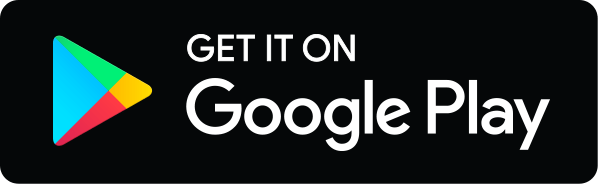
