Monocular Diplopia
Monocular diplopia is not related to a neurologic disorder and usually results from an optical problem (e.g., abnormal diffraction of light within the eye). It is almost always secondary to an ocular disease (e.g., cataract) or a refractive problem (e.g., astigmatism or issues with glasses).
It is typically alleviated when the patient looks through a pinhole (opaque panel perforated with one or multiple holes of 1 to 1.5 mm diameter). The holes restrict incoming light rays to a narrow path that bypasses refractive irregularities and presents a single, focused image to the retina. If monocular diplopia is due to an optical problem, the pinhole will usually make the secondary images disappear (▶ Fig. 13.1).
Fig. 13.1 Pinhole. The patient reads letters through small holes. Improvement of visual acuity suggests a refractive error or a media opacity. Monocular diplopia secondary to optical aberrations resolves or improves when looking through a pinhole.
Any uncorrected refractive error or media opacity may be responsible for monocular diplopia. Not all refractive errors can be fully corrected with glasses. In cases of monocular diplopia, correction with contact lenses should be tried.
Lens opacities in the visual axis are a common cause of monocular diplopia, which resolves after cataract surgery (▶ Fig. 13.2).
Fig. 13.2 Lens opacities responsible for monocular diplopia. The lens opacities (better seen on the enlarged photo on the right) are in the visual axis and are responsible for abnormal diffraction of incoming light.
Other causes of monocular diplopia include corneal surface irregularity (e.g., irregular astigmatism from pterygium or keratoconus, corneal edema, corneal scar, problem with contact lens, dry eye syndrome), post–cataract surgery (e.g., eccentric implant, tilted implant, multifocal implant, posterior capsular opacification), and retinal surface irregularity (e.g., macular edema, epiretinal membrane).
Damage to the visual association areas may very rarely produce duplicative images. In this case, the monocular diplopia or polyopia is present in both eyes (the patient sees double with either eye covered) and is identical in both eyes. Cerebral monocular diplopia is often monocular polyopia (i.e., more than two images).
Monocular diplopia or polyopia from cerebral lesions, however, is rarely an isolated finding; homonymous visual field defects are usually present, and other higher-order visual deficits such as alexia, prosopagnosia, and visual agnosia may be noted (see Chapters ▶ 10 and ▶ 11).
13.2 Binocular Diplopia
When the diplopia is binocular, it results from ocular misalignment secondary to dysfunction of the extraocular muscles, neuromuscular junction, ocular motor cranial nerves, or the internuclear and supranuclear pathways of eye movement control (▶ Fig. 13.3).
Fig. 13.3 Diagnosis of diplopia.
13.3 Understanding Eye Movements
Movements of the eyes are produced by the six extraocular muscles that are innervated by cranial nerves III (oculomotor nerve), IV (trochlear nerve), and VI (abducens nerve). To change visual fixation or to maintain fixation on an object that is moving relative to the observer, the eyes have to move with exquisite precision, and both eyes must move together. This requires a high degree of coordination of both the individual muscles to each eye and the muscle groups in each orbit. To achieve this, the nuclei of cranial nerves III, IV, and VI are controlled as a group by higher centers in the brainstem and the cerebrum.
Eye movements can be classified as follows, based on their role in vision:
Those that hold images steady on the retina
Fixation: holds the image of a stationary object on the fovea when the head is immobile
Vestibular (vestibulo-ocular reflex [VOR]): holds the image steady on the retina during brief head movements (tested using the oculocephalic reflex)
Optokinetic: holds the image steady on the retina during sustained head movements
Those that direct the fovea to an object of interest
Saccades: bring the image of an object of interest rapidly onto the fovea
Smooth pursuit: holds the image of a small moving target on the fovea
Vergence: moves the eyes in opposite directions (convergence or divergence) so that images of a single object are held simultaneously on both fovea
Six muscles are responsible for eye movements:
Medial rectus muscle: performs adduction
Lateral rectus muscle: performs abduction
Superior and inferior rectus muscles: elevate and depress the eye, respectively, performing these functions best when the eye is abducted.
Superior and inferior oblique muscles: work using a sling/pulley mechanism, with insertions of the muscles being located toward the posterior portion of the globe. The oblique muscles serve as rotators of the eye about the vertical and horizontal axis as the eye is viewed (clockwise or counterclockwise torsion), but also serve to elevate or depress the eye in adduction.
Superior oblique muscle: primarily intorts the eye (rotates the superior aspect of the globe toward the nasal bridge about the vertical axis, clockwise in the case of the right eye as viewed by the examiner); this muscle also depresses the eye in adduction.
Inferior oblique muscle: extorts the eye but also serves as an elevator in adduction.
In the primary position of gaze, the superior and inferior oblique and superior and inferior rectus muscles all perform a combination of vertical and torsional actions.
13.3.1 Laws of Ocular Motor Control
An agonist muscle moves the eye toward the desired direction. An antagonist muscle moves the eye away from the desired direction. According to Sherrington’s law of reciprocal innervation, whenever an agonist muscle receives an excitatory signal to contract, an equivalent inhibitory signal is sent to the antagonist muscle of the same eye (e.g., in left gaze the right medial rectus is excited while the right lateral rectus is inhibited). Yoke muscle pairs are pairs of muscles (one from each eye) that move both eyes toward the same direction (e.g., the left lateral rectus and the right medial rectus contract simultaneously in leftward gaze). According to Hering’s law of equal innervation, during conjugate eye movements, the muscles in the yoke muscle pair receive equal innervation so that the eyes move together.
13.3.2 Actions of the Extraocular Muscles
The primary action of an extraocular muscle is its major effect on the eye while the eye is looking straight ahead (in primary position). The secondary and tertiary actions of an extraocular muscle are additional effects on the eye while it is in primary position (▶ Table 13.1, ▶ Fig. 13.4).
Extraocular muscle | Primary action | Secondary action | Tertiary action |
Lateral rectus | Abduction | None | None |
Medial rectus | Adduction | None | None |
Superior rectus | Elevation | Incyclotorsion | Adduction |
Inferior rectus | Depression | Excyclotorsion | Adduction |
Superior oblique | Incyclotorsion | Depression | Abduction |
Inferior oblique | Excyclotorsion | Elevation | Abduction |
Fig. 13.4 Action of the six extraoculomotor muscles (right eye, examiner’s view). LR, lateral rectus, innervated by the abducens nerve (cranial nerve [CN] VI). SO, superior oblique, innervated by the trochlear nerve (CN IV); SR, superior rectus; IO, inferior oblique; MR, medial rectus; IR, inferior rectus. All are innervated by the oculomotor nerve (CN III), which also innervates the levator palpebrae and the pupil.
During the clinical examination, the primary position refers to the position when the eyes look straight ahead. The patient is then asked to look right, left, up, and down and then to look up and right, down and right, up and left, and down and left (▶ Fig. 13.5).
Fig. 13.5 Full extraocular movements in a normal subject (examiner’s view). The eye movements must be examined in the nine cardinal positions of gaze. IO, inferior oblique; IR, inferior rectus; LR, lateral rectus; MR, medial rectus; SO, superior oblique; SR, superior rectus.
The superior and inferior oblique muscles also rotate the eye, out for the inferior oblique (extorsion) and in for the superior oblique (intorsion) (▶ Fig. 13.6, ▶ Fig. 13.7, ▶ Fig. 13.8).
Fig. 13.6 a, b Cyclotorsion effect of the oblique muscles (on the right eye) as seen by the examiner. (a) The right inferior oblique extorts the right eye. (b) The left superior oblique intorts the right eye. The red arrow shows the action of the muscle, the black arrow demonstrates the resultant movement of the eye. (Adapted from From Schuenke M, Schulte E, Schumacher U, Ross LM, Lamperti ED, Voll M. THIEME Atlas of Anatomy, Head and Neuroanatomy. Stuttgart, Germany: Thieme; 2007. Illustration by Karl Wesker.)
Fig. 13.7 a, b Extraocular muscles in the orbit (right eye seen from above (a) and from the front (b)). (From Schuenke M, Schulte E, Schumacher U, Ross LM, Lamperti ED, Voll M. THIEME Atlas of Anatomy, Head and Neuroanatomy. Stuttgart, Germany: Thieme; 2007. Illustration by Karl Wesker.)
Fig. 13.8 a–c (a) Extraocular muscles on an axial computed tomographic scan of the orbits. The medial and lateral recti (in red) are seen on this cut. (b) Extraocular muscles on a coronal magnetic resonance imaging (MRI) scan of the orbits (medial rectus, inferior rectus, and inferior oblique complex; lateral rectus; superior rectus; and superior oblique are in red). The levator palpebra cannot be distinguished from the superior rectus. (c) Sagittal MRI of the orbits showing the superior and inferior recti. IO, inferior oblique; IR, inferior rectus; LP, levator palpebra; LR, lateral rectus; MR, medial rectus; SO, superior oblique; SR, superior rectus.
13.3.3 Cranial Nerves
The three ocular motor cranial nerves (III, IV, and VI) innervate the six extraocular muscles of each eye, the major eyelid elevator, and the parasympathetics (for pupillary constriction and lens accommodation) (▶ Fig. 13.9).
Fig. 13.9 Sagittal cut of the brain showing the origin of the ocular motor cranial nerves.
The third cranial nerve (oculomotor nerve) innervates the medial rectus, inferior rectus, superior rectus, inferior oblique, and levator palpebrae muscles, and it provides the parasympathetic pathway (pupillary constriction and accommodation).
The fourth cranial nerve (trochlear nerve) innervates the superior oblique muscle.
The sixth cranial nerve (abducens nerve) innervates the lateral rectus muscle.
All cranial nerves originate in the brainstem, where their nuclei are located. Each nerve has a short course within the brainstem (fascicle) prior to emerging and traveling in the subarachnoid space. Then all ocular motor cranial nerves enter the cavernous sinus and the superior orbital fissure to reach their corresponding extraocular muscles in the orbit. It is essential to understand the anatomical course of each cranial nerve to evaluate a patient with a cranial nerve palsy. By looking at accompanying signs, it is generally possible to accurately localize the lesion (▶ Fig. 13.10).
Fig. 13.10 Course of the third, fourth, sixth, and fifth cranial nerves (sagittal view). (From Schuenke M, Schulte E, Schumacher U, Ross LM, Lamperti ED, Voll M. THIEME Atlas of Anatomy, Head and Neuroanatomy. Stuttgart, Germany: Thieme; 2007. Illustration by Karl Wesker.)
13.3.4 Internuclear and Supranuclear Pathways
The initiation of conjugate eye movements is controlled by pathways and centers above the third, fourth, and sixth nerve nuclei (supranuclear pathways) and by interconnections among these nuclei (internuclear pathways). Inputs from the vestibular system (vestibulo-ocular pathways) also play an important role in the maintenance of eye position with head movement.
13.4 Examination of Eye Movements
Commonly Confused Ocular Motility Terms
Strabismus
This term is commonly used to describe ocular misalignment. It is a nonspecific term that does not indicate the underlying mechanism. It is, however, often used by ophthalmologists to describe congenital ocular deviation (i.e., congenital strabismus) rather than acquired ocular deviations. Neurologists do not use this term.
Comitance/Incomitance
Ocular misalignment (or strabismus) may be comitant or incomitant.
With comitant (or concomitant) strabismus, the magnitude of ocular deviation is the same in all directions of gaze and does not depend on the eye used for fixation.
With incomitant (or noncomitant) strabismus, the deviation varies in different directions of gaze. Most incomitant strabismus is caused by a paralytic or a mechanical restrictive process. The deviation is the largest when the eyes turn in the direction of the paralytic muscle. The deviation in incomitant strabismus also varies with the eye used for fixation. When the normal eye is fixating, the amount of misalignment is called the primary deviation. When the paretic eye is fixating, the amount of misalignment is called the secondary deviation. The secondary deviation is larger than the primary deviation in incomitant strabismus because an increase in innervation is needed for a paretic eye to fixate a target. By Hering’s law, the contralateral yoke muscle also receives more innervation, resulting in a larger deviation.
Causes of incomitant misalignment are as follows:
Extraocular muscle disease
Myasthenia gravis
Third, fourth, or sixth nerve palsy
Internuclear ophthalmoplegia
One-and-a-half syndrome
Causes of comitant misalignment are as follows:
Early childhood strabismus
Loss of fusion (severely decreased vision in one eye)
Acquired vergence disturbance (convergence, divergence)
Long-standing sixth nerve palsy (spread of comitance)
Skew deviation
Phoria/Tropia
A phoria (heterophoria) is an ocular deviation that occurs only when binocular fixation is disturbed, such as when one eye is covered. When viewing an object with both eyes, a subject with a phoria is capable of aligning the eyes to achieve fusion (single binocular vision). The descriptive prefixes (eso-, exo-, hyper-) are used to describe the heterophoria.
A tropia (heterotropia) is present even when both eyes are viewing and may result in diplopia. Similarly, the descriptive prefixes (eso-, exo-, hyper-) are used to describe the heterotropia.
Although phorias are very common in the general population, a phoria or a tropia has the same localization value in a patient with new-onset binocular diplopia.
Right and Left Designations of Phorias and Tropias
When a horizontal strabismus is incomitant and one eye is obviously deviated, then it makes sense to say “right esotropia” or “right exotropia.” In comitant horizontal strabismus, both eyes contribute equally to the problem, and the side is not designated. However, for vertical deviation, a side must be designated. Generally, the term hypertropia is preferred to hypotropia, and the patient is said to have a “right hypertropia” or a “left hypertropia” depending on which eye is higher. But “right hypertropia” or “left hypotropia” say the same thing: the right eye is higher than the left eye. These terms are describing the position of each eye only as it relates to the other and do not indicate the side of the pathological process. For example, a patient with a left third nerve palsy and an elevation deficit of the left eye may be described as having a “right hypertropia.”
Ocular Motor Nerves/Oculomotor Nerve
Ocular motor nerves refers to all three cranial nerves involved in ocular movements.
The oculomotor nerve is cranial nerve III alone.
13.4.1 Assessing Ocular Motility
The objective of the motility examination is to evaluate the integrity of the supranuclear and internuclear pathways, ocular motor nuclei, and ocular motor nerves and their muscles. Ductions (monocular eye movements) and versions (conjugate eye movements of both eyes) should be checked in the nine cardinal positions of gaze (“look up, look down, look right, look left”) (▶ Fig. 13.11).
Fig. 13.11 Full extraocular movements in a normal subject. IO, inferior oblique; IR, inferior rectus; LR, lateral rectus; MR, medial rectus; SO, superior oblique; SR, superior rectus.
The examiner should assess the eye movement by recording it as a percentage of normal.
For example, an abduction deficit in an isolated right sixth nerve palsy would be recorded as follows (▶ Fig. 13.12):
Fig. 13.12 Right abduction deficit from a right sixth nerve palsy.
Incomplete abduction of the right eye (20% of normal)
Normal elevation, adduction, and depression of the right eye
Full left eye movements
Absence of ptosis
Normal pupils
Another way to record eye movements is to use a scale from − 4 to 0 (with − 4 being complete paresis) and 0 being full eye movement (discussed later in chapter, ▶ Fig. 13.25c). With this scale, the incomplete abduction deficit shown in ▶ Fig. 13.12 would be described as a − 3 abduction of the right eye.
Paresis or Restriction
When movements of one or both eyes are limited, it is important to determine whether the limitation is due to a paresis of the muscle or a restriction of the muscle. For example, an abduction deficit from a sixth nerve palsy results from a paresis of the lateral rectus muscle. On the other hand, an abduction deficit from an enlarged medial rectus in thyroid eye disease results from restriction of the medial rectus. Additionally, an elevation deficit of one eye after an orbital floor fracture is most often due to a restriction of the inferior rectus that is bruised or trapped within the fracture.
In cooperative patients, the forced duction test allows differentiation between paresis and restriction (▶ Fig. 13.13) and is performed as follows:
Fig. 13.13 The right eye is deviated toward the nose. The examiner pulls on the conjunctiva with forceps while asking the patient to look to the right to verify that the right eye movements are not restricted.
Instill two drops of topical anesthetic in the eye.
Attempt to move the eye by pushing on the deviated globe with a cotton tipped swab or by pulling on the conjunctiva with forceps, while asking the patient to look in that direction.
Inability of the examiner to move the eye suggests restriction.
Bell Phenomenon
With eye closure, there is a normal upward rotation of the eye. This is called the Bell phenomenon. It keeps the cornea from being exposed during sleep.
This normal reflex is easily visible in patients with peripheral facial nerve paralysis who attempt to shut their eyes (▶ Fig. 13.14).
Fig. 13.14 a, b Right peripheral facial nerve palsy. (a) The right palpebral fissure is larger than the left (lagophthalmos). (b) With attempted eye closure, the Bell phenomenon is seen in the right eye.
In normal patients, it can be checked by having the patient try to close the eyes while the examiner holds the lids open.
In a patient with limited up gaze, a normal Bell phenomenon indicates a supranuclear defect with intact nuclear and infranuclear function (e.g., the Bell reflex is preserved despite up gaze paresis in Parinaud (dorsal midbrain) syndrome but is absent in a complete third nerve palsy or myopathy).
Saccades
Saccades, or fast eye movements, are tested by having the patient refixate between two targets. This can be easily performed by having the patient look at the examiner’s nose and then at an eccentrically placed finger: “look at my nose, look at my finger” (▶ Fig. 13.15).
Fig. 13.15 Testing saccades by asking the patient to look from one finger to the other.
Pursuit
To test the pursuit system, the patient is asked to keep his or her head still and to follow a target (the examiner’s finger) (“follow my finger”).
Oculocephalic Responses
Oculocephalic responses are used to test the vestibulo-ocular reflex and need to be tested in all patients with horizontal or vertical gaze paresis. Normally, the eyes should deviate conjugately in the direction opposite the head turn (▶ Fig. 13.16). Oculocephalic responses should not be tested in trauma patients who may have spinal lesions. Abnormal eye movements that are overcome by oculocephalic maneuvers are likely supranuclear (or nonorganic).
Fig. 13.16 Testing oculocephalic responses. The oculocephalic response (the vestibulo-ocular reflex) can be evaluated by having the patient fixate on a stationary target (the examiner’s nose or the patient’s own thumb), while the examiner gently rotates the head (horizontal), then extends and flexes the neck (vertical).
Vergences
Vergences are binocular eye movements in which the two eyes move in opposite directions (the eyes need to converge to see a single image at near, and need to diverge to see a single image at distance).
Convergence can be evaluated by having the patient look at his or her thumb or other accommodative target as it is brought toward the nose. Both eyes should adduct and there will be pupillary constriction and accommodation of the lens (the “near triad”) (▶ Fig. 13.17). Convergence insufficiency is a common cause of binocular horizontal diplopia when the patient is reading. Patients develop an exotropia at near (to be discussed).
Fig. 13.17 Testing convergence.
13.4.2 Assessing Ocular Misalignment
When a patient complains of binocular diplopia, there must be ocular misalignment. If you conclude that the “extraocular movements are full,” it usually means that you were not able to visualize small extraocular motor deficits. This is when the cover–uncover test, the cross-cover test, the red glass test, or the Maddox rod test becomes very helpful.
The Cover–Uncover Test
This test is based on evoking a fixational eye movement. In nearly all cases of ocular misalignment, one eye fixates the target while the other deviates. If the fixating eye is covered, the deviating eye will move to fixate the target. This test requires good vision and an attentive patient.
Procedure
The patient is instructed to fixate on a distant target while the right eye is covered. The left eye is observed to determine if it makes a fixational saccade toward the straight-ahead (primary) position. The direction of movement must be noted. Following removal of the cover from the right eye and after a delay of a few seconds, the left eye is covered and the right eye is watched for a fixational movement (▶ Fig. 13.18).
Fig. 13.18 a–d Cover–uncover test. (a) Esotropia (the eyes are deviated in). (b) Exotropia (the eyes are deviated out). (c) Left hypertropia (the left eye is higher than the right eye). (d) Left hypotropia (the left eye is lower than the right eye). The red arrows show the movement of the uncovered eye during the cover-uncover test.
This cover–uncover maneuver should be repeated on each eye until the observations are consistent. If a fixational eye movement repeatedly occurs in the same direction, a “manifest misalignment” or a “tropia” can be diagnosed as follows:
An outward movement denotes a convergent misalignment, or esotropia.
An inward movement denotes a divergent misalignment, or exotropia.
A downward movement denotes a hypertropia.
An upward movement denotes a hypotropia.
The type of misalignment in primary position, right, left, up, and down gaze should be noted (see ▶ Fig. 13.18).
The Cross-Cover Test
This test is used to determine if there is latent misalignment not revealed by the cover–uncover test. It is based on eliciting a fixational eye movement, like the cover–uncover test. However, it interferes with binocular vision to bring out any latent misalignments that might be suppressed by a force called binocular fusion. This force is normally exerted to avoid double vision when both eyes are viewing a target.
Procedure
After covering the right eye as before, and after a 1 second pause, the cover is quickly moved across the nasal bridge to cover the left eye. Pausing 1 second, the cover is shifted back to cover the right eye, then the left eye, and so on, alternately covering the two eyes. One observes whether the eye being uncovered is shifting its position, the observations being recorded as with the cover–uncover maneuver. If a consistent fixational eye movement that was not present with the cover–uncover test is elicited, a latent misalignment, or phoria, is identified. Phorias, particularly exophorias, are so common in normal individuals that they may be considered physiological unless the degree of misalignment is incomitant (i.e., varies from one gaze position to another). When the cross-cover test does not elicit any eye movement, the patient is said to be orthophoric (▶ Fig. 13.19).
Fig. 13.19 Cross-cover test–orthophoric in horizontal gaze. The occluder is shifted from one eye to the other as demonstrated in successive frames 1 through 4. The patient is tested while looking straight first (middle column), then when looking to the right (left column), then when looking to the left (right column).
The test is repeated in all directions of gaze to make sure that the patient is orthophoric in right, left, up, and down gazes (▶ Fig. 13.20).
Fig. 13.20 Cross-cover test–orthophoric in vertical gaze. The occluder is shifted from one eye to the other (i.e., frame 1 to frame 2). The patient is tested first while looking straight ahead (middle row), then while looking up (top row), then while looking down (bottom row).
In the patient with a complaint of horizontal diplopia, the cross-cover test may show an abduction deficit (▶ Fig. 13.21).
Fig. 13.21 Cross-cover test–right abduction deficit. The patient is tested while looking straight first (middle column), then when looking to the right (left column), then when looking to the left (right column). In straight-ahead gaze, covering the left eye elicits an outward deviation of the right eye (arrow), indicating an esotropia. The deviation is worse in right gaze and absent in left gaze, suggesting a right abduction deficit. The red arrows show the movement of the uncovered eye during the cross-cover test.
In the patient with a complaint of new vertical diplopia, the three-step test is critical in looking for a fourth nerve palsy (▶ Fig. 13.22).
Fig. 13.22 Cross-cover test–right superior oblique paresis (fourth nerve palsy). The patient is tested while looking straight first (middle column), then when looking to the right (left column), then when looking to the left (right column). Down gaze and head tilt are subsequently tested.
The three-step test measures ocular alignment in (1) straight-ahead gaze [step 1]; (2) right and left gaze [step 2]; (3) straight-ahead gaze with the head tilted right and then tilted left [step 3]. When the head is erect, the patient has a small right hyperdeviation (hypertropia) in straight-ahead gaze; the patient has normal alignment in right gaze, but a large right hyperdeviation in left gaze. In right head tilt, the patient has a very large right hyperdeviation; in left head tilt, the patient’s eyes are aligned. The patient also has a large right hypertropia in down gaze. The yellow arrows show the movement of the uncovered eye during the cross-cover test.
Step 1: Because there is a right hyperdeviation in straight-ahead gaze, there must be a deficit in the depressor muscles of the right eye (inferior rectus, superior oblique) or the elevator muscles of the left eye (superior rectus, inferior oblique).
Step 2: If the hyperdeviation is greater on left gaze than on right gaze, a vertical muscle active in left gaze is weak—either the right superior oblique or the left superior rectus.
Step 3: Tilting the head to either side evokes an otolith-mediated ocular righting response (“ocular tilt reaction”) designed to keep the eyes close to the plane of the horizon. In the right head tilt position, the right eye needs to intort (superior oblique, superior rectus), and the left eye needs to extort (inferior oblique, inferior rectus). If the right eye’s superior oblique is weak, then the only available “intorter” in that position is the intact right superior rectus. Because the primary action of the superior rectus is elevation, the depressor force of the weak superior oblique will be overwhelmed by the elevator force of the intact superior rectus, and the right globe will move upward. As for the left eye, its intact inferior oblique upward pull is neutralized by its intact inferior rectus downward pull, so the globe does not move vertically. Left head tilt evokes an ocular counter-rolling motion that uses four intact muscles, so that neither eye moves vertically.
Quantification of the Ocular Deviation
The ocular misalignment can be quantified by determining the power of the prism necessary to neutralize the ocular deviation (▶ Fig. 13.23). The prism is placed over one eye, with the apex of the prism (point) pointed in the direction in which the eye is deviated: base out for an esotropia, base in for an exotropia, and base down on the right eye for a right hypertropia (▶ Fig. 13.24; “the apex of the prism points toward the deviation”).
Fig. 13.23 a, b Types of prisms used to quantify the ocular deviation. (a) Single prisms. (b) Prism bars.
Fig. 13.24 Prism–alternate cover test.
Each eye is then alternatively covered as the amount of prism (measured in prism-diopters) is slowly increased. When the eyes no longer move on alternate-cover testing, the deviation has been neutralized and the amount of prism required can be read on the prism or the prism bar. This measurement can be done in all cardinal positions and at near, as well as with head tilt.
Pearls
Measurement of the ocular deviation in prism-diopters reliably quantifies the ocular deviation. It is very useful for follow-up and to verify stability prior to considering eye muscle surgery for diplopia.
The same technique of prism quantification can be used during the red glass test or the Maddox rod test. With horizontal and vertical deviations, the same prism techniques can be used, and prisms of different orientations can be combined at the same time.
Communicating the Results of the Examination
The eye movements can be described in several ways (▶ Fig. 13.25 and ▶ Fig. 13.26).
Fig. 13.25 a–c (a) Diagram for transcription of eye movements. (b) Example of limitation of eye movements in the left eye using the percentage method. Only abduction and intorsion are normal in the left eye. There is only 25% of normal elevation and depression of the left eye, and only 10% of normal adduction. (c) Using the 0 to – 4 scale, abduction of the left eye would be 0, and elevation, depression, and adduction would be – 3. These measurements suggest a left third nerve palsy. IO, inferior oblique; IR, inferior rectus; LR, lateral rectus; MR, medial rectus; SO, superior oblique; SR, superior rectus.
Fig. 13.26 a, b (a) Diagram for transcription of eye movements (with cross-cover test). (b) Example of a right hypertropia (RHT) measuring 4 prism diopters in primary position, increasing to 8 prism diopters in down gaze, resolving in right gaze and up gaze, and increasing to 10 prism diopters in left gaze. With right tilt, there is an 8 prism diopter right hypertropia, and with left head tilt, it improved to 2 prism diopters. These measurements indicate a right fourth nerve palsy.
The Red Glass Test
The red glass test relies on the patient’s ability to report the location of two different-colored lights. By convention the red filter is placed in front of the right eye (▶ Fig. 13.27). This maneuver is then repeated for all the relevant positions of gaze (▶ Fig. 13.28). The patient is requested to stare straight ahead at a bright light. If the eyes are aligned, the patient will report one red-tinged light; however, if they are out of alignment, the patient describes one white and one red light. The extent of separation of images horizontally and vertically is determined.
Fig. 13.27 Red glass test—orthophoric in horizontal gaze.
Fig. 13.28 Red glass test—orthophoric in up and down gaze.
With an esotropia or esophoria, the red image (as seen by the right eye) will appear to the patient as displaced to the right of the white image (▶ Fig. 13.29).
Fig. 13.29 Red glass test—right abduction deficit. With the red glass placed over the right eye, the patient reports that, in straight-ahead gaze, the red light appears to the right and the white light to the left, indicating an esotropia. In right gaze, the separation increases, indicating a greater esotropia in right gaze. In left gaze, the patient sees only a single red-tinged light.
With an exotropia or exophoria, the red image (as seen by the right eye) will appear to the patient as displaced to the left. One way to remember this rule of horizontal misalignment is that there is an X in exotropia. This means that the red image viewed by the right eye crosses over to the left of the white light in an exotropia (▶ Fig. 13.30).
Fig. 13.30 Red glass test—left adduction deficit. With the red glass placed over the right eye, the patient reports that, in straight-ahead gaze, the red light appears to the left and the white light to the right, indicating an exotropia. In right gaze, the separation increases, indicating a greater exotropia in right gaze (this patient has a partial left third nerve palsy with an adduction deficit of the left eye). In left gaze, the patient sees only a single red-tinged light.
With a right hypertropia (▶ Fig. 13.31), the red image will appear displaced below the white image; with a left hypertropia, the red image will appear displaced above the white image. One way to remember the rule for vertical deviations is that there is an R in sunrise. The red image sits below the white light (the sun) in a right hypertropia.
Fig. 13.31 Red-glass test—right hypertropia. There is a subtle elevation deficit of the left eye (partial left third nerve palsy), and the patient complains of binocular vertical diplopia in primary position and when looking up. With the red glass placed over the right eye, the patient reports that, in straight-ahead gaze, the red light appears below the white light, indicating that the right eye is higher than the left eye (right hypertropia). In up gaze, the separation increases, indicating a greater deviation. In down gaze, the patient sees only a single red-tinged light, indicating no vertical deviation.
The red glass test is more sensitive to small amounts of misalignment than the cover test, but it requires an attentive and verbally adept patient. Some patients have difficulty estimating spacing intervals, especially when the eyes are misaligned in two planes; the red glass test using the Maddox rod improves reliability under these circumstances.
The Maddox Rod Test
The Maddox rod itself consists of a stack of transparent red plastic cylinders. When placed between a light source and the eye, it produces an image of a straight red line oriented at 90 degrees to the axis of the cylinders (▶ Fig. 13.32).
Fig. 13.32 Maddox rod with cylinders oriented horizontally and vertically.
Thus, when the Maddox rod is held before the eye with cylinders oriented vertically, the viewer sees a point light source as a horizontal red line. When the Maddox rod cylinders are oriented horizontally, the red line is seen as vertical. This optical phenomenon allows the examiner to assess misalignment first in the horizontal plane, then in the vertical plane.
13.4.3 Testing for Horizontal and Vertical Deviation
The Maddox rod is used in the same way as the red glass, except that the patient is tested in all relevant gaze positions twice—first with the cylinders oriented horizontally and then with the cylinders oriented vertically (▶ Fig. 13.33 and ▶ Fig. 13.34).
Fig. 13.33 Maddox rod test—no horizontal deviation. The Maddox rod is placed in front of the right eye, with the cylinders oriented horizontally. The patient looks at the examiner’s light. The patient sees a white light and a vertical red line. When there is no horizontal ocular deviation (orthophoric), the patient sees the light through the red line.
Fig. 13.34 Maddox rod test—no vertical deviation. The Maddox rod is placed in front of the right eye, with the cylinders oriented vertically. The patient sees a white light and a horizontal red line when looking at the examiner’s light.
For horizontal deviations, the Maddox rod is placed in front of the right eye with the cylinders oriented horizontally. When there is no horizontal ocular deviation (orthophoric), the patient sees the light through the red line. When the line is seen to the right of the light (uncrossed diplopia), there is an esotropia (▶ Fig. 13.35). When the line is seen to the left of the light (crossed diplopia), there is an exotropia (▶ Fig. 13.36).
Fig. 13.35 Maddox rod test—right abduction deficit (esodeviation). When the Maddox rod with cylinders oriented horizontally is placed in front of the right eye as the patient gazes straight ahead, a vertical red line appears 1 inch to the right of the white light. In right gaze, the vertical red line appears 3 inches to the right, and in left gaze, the vertical red line intersects the white light.
Fig. 13.36 Maddox rod test—left adduction deficit (exodeviation). A vertical red line appears
1 inch to the left of the white light when the Maddox rod with cylinders oriented horizontally is placed in front of the patient’s right eye in a straight-ahead gaze. In right gaze, the vertical red line appears 3 inches to the left, and in left gaze, the vertical red line intersects the white light.
For vertical deviations, the Maddox rod is placed in front of the right eye, with the cylinders oriented vertically; the patient looks at the examiner’s light. The patient sees a white light and a horizontal red line. When there is no vertical ocular deviation (orthophoric), the patient sees the light through the red line (see ▶ Fig. 13.34). When the light is seen above the line, there is a right hypertropia (▶ Fig. 13.37). When the light is seen below the line, there is a right hypotropia (or left hypertropia).
Fig. 13.37 Maddox rod test—right hypertropia. In the case of a subtle elevation deficit of the left eye (partial left third nerve palsy), with the Maddox rod placed over the right eye, the patient reports that, in straight-ahead gaze, the red line appears below the white light, indicating that the right eye is higher than the left eye (right hypertropia). In up gaze, the separation increases, indicating a greater deviation. In down gaze, the patient sees the red line intersect the white light, indicating no vertical deviation.
Testing for Cyclotorsion
The double Maddox rod test helps demonstrate and quantify cyclotorsion: Maddox rod lenses are placed over each eye in a trial frame (by convention, red in front of right eye and white in front of left eye), with the cylinders oriented vertically (each at the 90 degree mark). As the patient is viewing a hand-held light at distance, two separate horizontal lines will be seen, one with each eye (right eye: red line; left eye: white line). The patient is asked to align the two lines so that they are parallel to each other and the floor by rotating the dials of the trial frame (▶ Fig. 13.38 and ▶ Fig. 13.39).
Fig. 13.38 Double Maddox rod testing in a patient with no deviation (the yellow lines show the orientation of the Maddox rods).
Fig. 13.39 Double Maddox rod testing in a patient with a left fourth nerve palsy (left hypertropia). The patient shows excyclotorsion of the left eye while the right eye is not torted (the yellow lines show the orientation of the Maddox rods).
13.4.4 Testing Uncooperative Patients or Young Children
Observe spontaneous eye movements and eye position to determine whether the ocular alignment is normal.
Use the Hirschberg test (corneal reflection test).
Try the uncover–cover test with a noisy target such as a toy.
The Hirschberg and Krimsky Tests
In uncooperative patients or in children, ocular deviations can be assessed using corneal reflexes. When a fixation light is held 33 cm from a patient, the corneal light reflex should be centered in each pupil and should be symmetric. When the light reflex is deviated from the center of the pupil, there is a tropia (▶ Fig. 13.40).
Fig. 13.40 Demonstration of an esotropia by the Hirschberg test. Note the corneal light reflex in each eye (arrows).
Hirschberg test: the deviation of the corneal light reflex from the center of the pupil can be estimated in millimeters (see green arrow in ▶ Fig. 13.41):
Fig. 13.41 Deviation of the corneal light reflex from the center of the pupil (green circle) in esotropia (green arrow).
1 mm decentration = 7 degrees of ocular deviation = 14 prism-diopters
The deviation of the light reflex is measured as follows:
Edge of pupil: 15 degrees = 30 prism-diopters
Middle iris: 30 degrees = 60 prism-diopters
Edge of iris: 45 degrees = 90 prism-diopters
Krimsky test: using the Hirschberg test, prisms of increasing power can be placed in front of the fixating eye until both corneal reflexes are centered. The prism with sufficient power to achieve centration of the light reflex indicates the magnitude of the deviation (▶ Fig. 13.42).
Fig. 13.42 Measurement of the esotropia by Krimsky test (prisms base out are placed in front of the fixating eye; the tip of the prism points toward the ocular deviation).
Esotropia: orient the prism base out
Exotropia: orient the prism base in
Hypertropia: orient the prism base up (over the fixating eye); most often patients fixate with the normal eye (the hypertropic eye is deviated upward); hence the need to place the prism base up over the normal fixating eye.
Hypotropia: orient the prism base down (over the fixating eye); most often patients fixate with the normal eye (the hypotropic eye is deviated downward); hence the need to place the prism base down over the normal fixating eye.
13.4.5 Testing Comatose Patients
Eye movements are assessed using reflex maneuvers. In the oculocephalic reflex, the eyes should deviate conjugately in the direction opposite the head turn. Dysconjugate horizontal eye movements suggest a sixth nerve palsy, internuclear ophthalmoplegia, or third nerve palsy. Oculocephalic eye movements are typically preserved in cerebral hemisphere injury. Oculocephalic maneuvers should not be performed in patients with cervical injury.
If the patient does not have any oculocephalic eye movements (or if testing them is contraindicated), caloric testing can help evaluate eye movements.
After examination of the external auditory canal to exclude a rupture of the tympanic membrane or cerumen impaction, 30 to 60 mL of ice-cold water is irrigated into the patient’s ear with the head angled 30 degrees above the horizontal (to align the horizontal semicircular canals perpendicular to the floor). The eyes deviate slowly toward the irrigated ear in patients with intact brainstem function, followed by a fast corrective phase in the opposite direction to reset the eyes, and then the cycle repeats. Warm water stimulation produces a contralateral slow phase and ipsilateral fast phase.
The direction of the caloric response is named after the fast phase:
(remember: COWS for cold—opposite, warm—same)
Pearls
The goals of the evaluation of the diplopic patient are as follows:
Recognize the ocular misalignment.
Characterize the misalignment.
Determine the type of deviation: esotropia, exotropia, hypertropia.
Quantify the misalignment (prisms).
Ascertain whether it is comitant (same amount of deviation in all directions of gaze) or incomitant (varies from one direction of gaze to another).
Determine whether it maps to a cranial nerve or suggests a muscle or neuromuscular junction lesion.
Describe the pupils, visual acuity, and fundus.
Look for orbital signs.
Look for associated neurological signs.
These findings allow you to do the following:
Localize anatomically the lesion responsible for the diplopia.
Make hypotheses regarding the mechanism of the lesion.
Obtain further testing to confirm your clinical impression.
13.5 The Diagnosis of Binocular Diplopia
It is important to determine by clinical examination whether the lesion responsible for diplopia involves the extraocular muscles, the neuromuscular junction, a cranial nerve, or the internuclear or supranuclear pathways.
13.5.1 The Lesion Is in the Extraocular Muscles
Disorders of the extraocular muscles (and neuromuscular junction) can produce a wide range of abnormal eye movements. Their clinical manifestations are not limited by the scope of a single cranial nerve or supranuclear process. These disorders are frequently bilateral, involve the levator palpebrae and the orbicularis oculi, and never involve the pupil. They can result in total bilateral ophthalmoplegia and are sometimes associated with systemic manifestations. Numerous disorders can involve the extraocular muscles directly (myopathy) or can involve the orbit and impair the free movements of the muscles in the orbit.
Diseases of the extraocular muscles can produce motility disturbances in two ways: weakness (paresis) or restriction.
The disease process can affect the muscle’s ability to contract and cause weakness, or paresis.
The muscle’s movements may be restricted by local disease (such as inflammation, fibrosis, trauma, tumor). In this case, the eye is pulled in the direction of the abnormal muscle (▶ Fig. 13.43).
A forced duction test allows differentiation between paresis (the paretic eye moves easily when pushed or pulled) and restriction (the restricted eye does not move). This test can be painful and may be difficult to interpret (see ▶ Fig. 13.13).
Fig. 13.43 a, b (a) Trauma to the right eye with mild ecchymosis. The right eye does not elevate with attempted up gaze (b). (c) Coronal computed tomographic scan of the orbits without contrast showing a fracture of the right orbital floor. There is herniation of the orbital contents (including inferior rectus and inferior oblique muscles), resulting in restriction of elevation of the right eye.
Differential Diagnosis of Enlarged Extraocular Muscles
Enlarged ocular muscles may result most commonly from the following:
Thyroid eye disease
Inflammatory disorders
Idiopathic orbital inflammation with myositis (inflammatory orbital pseudotumor)
Immunoglobulin G4 (IgG4) related disease
Wegener granulomatosis
Sarcoidosis
Crohn disease and inflammatory bowel syndrome
Connective tissue diseases
Tumors
Lymphoma
Metastatic tumors
Rhabdomyosarcoma
Infections
Trichinosis
Orbital venous congestion
Carotid cavernous fistula
Carotid cavernous thrombosis
Infiltration
Amyloidosis
Thyroid Eye Disease
Thyroid eye disease (Graves disease) is an autoimmune disorder characterized by enlargement of the extraocular muscles and an increase in orbital fat volume.
Pearls
The vast majority of patients with enlarged extraocular muscles have thyroid eye disease or idiopathic orbital inflammation (orbital pseudotumor).
Clinical Presentation
Thyroid eye disease is usually easily suspected clinically when patients present with the following:
Unilateral or bilateral proptosis
Lid retraction with lid lag in down gaze
Ptosis (rare and should indicate possible associated myasthenia gravis)
Orbital congestion with periorbital edema, puffiness of the eyelids, chemosis (these signs are worse in the morning)
Diplopia with restricted eye movements (restricted elevation of the eye and esotropia are most common because of the classic involvement of the inferior and medial recti) (▶ Fig. 13.44 and ▶ Fig. 13.45)
Visual loss, which can occur from the following:
Ocular surface disorder (dry eyes are common)
Exposure keratopathy (in the setting of severe proptosis)
Compressive optic neuropathy by the enlarged extraocular muscles
Glaucoma (increased intraocular pressure is common in thyroid eye disease)
Fig. 13.44 a, b Restricted eye movement from thyroid eye disease. (a) The eyes in primary position. There is severe proptosis with lid retraction, chemosis, and corneal exposure. The eye movements are diffusely restricted. (b) Coronal computed tomographic scan of the orbits with contrast showing enlargement of all extraocular muscles.
Fig. 13.45 a, b Restricted eye movement from thyroid eye disease. (a) Position of the eyes in up gaze. The right eye does not elevate. There is mild conjunctival hyperemia and lid retraction. The proptosis is mild. (b) Axial computed tomographic scan of the orbits with contrast showing enlargement of the medial recti and normal lateral recti in both eyes. There is a right abduction deficit (small red arrow) related to restriction of the enlarged medial rectus muscle. The adduction of the right eye (long red arrow) is normal.
Thyroid dysfunction in patients with thyroid eye disease is variable: 90% of patients are hyperthyroid and up to 6% of patients are euthyroid. There is no correlation between the thyroid hormone levels and the orbital disease. However, some treatments administered to treat hyperthyroidism (such as radioactive iodine) often worsen the orbital manifestations of Graves disease.
Pearls
The inferior rectus muscle is commonly involved in thyroid eye disease, causing restricted up gaze. The medial rectus muscle is the second most commonly involved muscle (causing an esotropia), followed by the superior and lateral rectus muscles.
Diagnosis
Diagnosis relies mostly on clinical presentation and imaging: diagnosis of thyroid eye disease is sometimes based only on the findings of enlarged extraocular muscles on a computed tomographic (CT) scan or magnetic resonance imaging (MRI) of the orbits.
Thyroid function tests are often normal.
Autoantibodies (antithyroglobulin and antiperoxidase antibodies) are sometimes present and indicate an autoimmune disorder.
Treatment
Treat thyroid abnormalities.
Lubricate the cornea; temporary tarsorrhaphy may be required if there is corneal exposure.
Treat elevated intraocular pressure.
Elevate the head of bed at night to decrease orbital congestion.
Use ocular occlusion and prisms for diplopia.
Administer steroids (usually oral prednisone, except in cases of compressive optic neuropathy, in which case high-dose intravenous steroids may be preferred).
If surgery is necessary, do orbital decompression first, followed by strabismus surgery and lid repair.
Radiation therapy of the orbits is sometimes helpful.
Encourage patients to discontinue cigarette smoking, which is associated with a worse prognosis for thyroid eye disease.
Pearls
Thyroid eye disease is the most common cause of unilateral and bilateral proptosis. Thyroid eye disease and idiopathic orbital inflammation (orbital pseudotumor) can usually be differentiated based on clinical and radiologic characteristics (▶ Table 13.2, ▶ Fig. 13.46 and ▶ Fig. 13.47).
Table 13.2 Comparison of thyroid eye disease and inflammatory orbital pseudotumor
Thyroid eye disease
Inflammatory orbital pseudotumor
Common
Rare
Usually bilateral
More often unilateral
Chronic disease
Acute or subacute onset
No pain or mild discomfort
Pain often severe
Inferior rectus and medial rectus are most often affected
Any extraocular muscle is affected
Lid retraction
Ptosis
Response to steroids often moderate
Response to steroids often dramatic
Fig. 13.46 Axial computed tomographic scan of the orbits with contrast showing thyroid eye disease. Enlargement and enhancement of both medial recti sparing the tendon itself (arrow). The orbital fat is not enhancing.
Fig. 13.47 Axial T1-weighted magnetic resonance imaging of the orbits with fat suppression and contrast showing orbital inflammatory pseudotumor. Enlargement and enhancement of the right medial rectus extending to the tendon itself (arrow). The orbital fat is also enhancing on the right.
Myositis
Inflammation of extraocular muscles can occur in the setting of any orbital inflammatory syndrome (such as nonspecific inflammation from orbital pseudotumor or Wegener granulomatosis) or any orbital infection (orbital cellulitis).
Clinical Presentation
Orbital myositis classically represents with a painful orbital symptoms, which includes the following:
Pain over the eye or periorbital region
Orbital syndrome
Periorbital swelling
Proptosis
Redness of the eye, chemosis
Possible visual loss from optic neuropathy
Diplopia with limitation of eye movements
Ptosis or lid retraction (if major proptosis)
Imaging of the orbit (MRI of the orbits with fat suppression and contrast or CT of the orbits with contrast) confirms the diagnosis by showing enlargement and enhancement of the extraocular muscles and their tendons, as well as enhancement of other orbital structures (fat, sclera, optic nerve sheath).
Infectious Myositis
Trichinosis is a parasitic infection (undercooked meat is the vector) with a predilection for muscles. It is an acute systemic myositis involving the extraocular muscles associated with bilateral periorbital edema, diarrhea, eosinophilia, and fever.
Infectious orbital cellulitis and orbital or periosteal abscesses can result in ophthalmoplegia and diplopia. Infectious sinusitis involving the facial sinuses adjacent to the infected orbit is common, particularly in children (▶ Fig. 13.48).
Fig. 13.48 a, b (a) Right orbital cellulitis with ptosis, ophthalmoplegia, pain, and periorbital edema and redness. (b) Coronal computed tomographic scan of the orbits with contrast showing an inferior orbital abscess with bone erosion and adjacent sinusitis.
Treatment with broad spectrum antibiotics and surgical drainage usually results in dramatic improvement.
Noninfectious Inflammatory Myositis, or Idiopathic Orbital Inflammation (Pseudotumor)
In most cases, inflammatory orbital myositis is due to “idiopathic inflammation” of the orbital contents, also called orbital pseudotumor (▶ Fig. 13.49). There is no infection and no underlying systemic disorder.
Fig. 13.49 a, b (a) Left idiopathic orbital inflammation with myositis. Eye movements with attempted left gaze showing left proptosis, ptosis, periorbital swelling, and conjunctival hyperemia. The left eye does not abduct (restriction of all eye movements). (b) Coronal computed tomography of the orbits without contrast demonstrating very large extraocular muscles in the left orbit, consistent with myositis.
Diagnosis
The diagnosis is suspected clinically in an otherwise healthy patient presenting with unilateral or bilateral acute or subacute orbital syndrome associated with pain. Diplopia is very common, and visual loss can occur as a result of adjacent inflammation of the optic nerve or of the sclera.
Infectious orbital cellulitis is ruled out by the following:
Absence of fever
Absence of associated sinusitis or abscess on imaging
Normal white blood cell count
General examination and blood tests rule out an underlying systemic disorder such as IgG4 related disease, Wegener granulomatosis, sarcoidosis, Crohn disease, connective tissue diseases.
Clinical Presentation
All orbital structures can be involved in idiopathic orbital inflammation (orbital pseudotumor), explaining the various clinical presentations of this syndrome:
Extraocular muscles
Myositis, which produces ophthalmoplegia
Orbital fat
Proptosis
Optic nerve sheath
Perineuritis and disc edema
Optic nerve
Optic neuritis and visual loss (optic neuropathy)
Sclera
Posterior scleritis and visual loss
Lacrimal gland
Dacryoadenitis with eyelid swelling
Pain is usually severe, although sclerosing forms of orbital pseudotumor may have a more chronic indolent course.
The inflammation may also extend into the cavernous sinus, resulting in multiple cranial nerve palsies. A similar disorder can involve the intracranial meninges and is called pachymeningitis.
Treatment
Orbital pseudotumor usually responds dramatically to steroid treatment. Symptoms and signs improve within a few hours after receiving steroids. When there is visual loss, intravenous steroids are usually preferred to oral prednisone.
Steroids need to be tapered very slowly, and steroid dependence (rebound during taper or after discontinuation) is common. For this reason, steroid-sparing agents or radiation of the orbit is sometimes recommended. Lubrication of the cornea and management of intraocular pressure are important during the acute phase.
Myositis of the Superior Oblique Muscle or Its Tendon
This produces an acquired Brown syndrome. The eye does not elevate in adduction (there is a downshoot of the eye in adduction).
Sometimes, Brown syndrome is intermittent (the superior oblique tendon is intermittently blocked in the pulley), and the patient hears a “snap” when the tendon releases and the eye fully elevates.
Orbital Tumors
Lymphoid tumors (e.g., lymphoma or leukemia) and metastases can involve extraocular muscles in the orbit and can present as a subacute or acute orbital syndrome associated with pain. An orbital biopsy should be considered in all patients with presumed “myositis” and a known history of cancer.
Metastases of breast cancer are commonly associated with enophthalmos rather than proptosis, presumably because of associated orbital fat atrophy and fibrosis (▶ Fig. 13.50).
Fig. 13.50 a, b (a) Painful diffuse ophthalmoplegia of the right eye, which is also mildly enophthalmic. This patient has a history of breast cancer. (b) Axial computed tomographic scan of the orbits with contrast showing enhancement of the entire right orbit. An orbital biopsy confirmed a metastasis from breast cancer.
Pearls
An orbital biopsy should be considered in all patients with presumed “myositis” and a known history of cancer and in patients with atypical orbital pseudotumor (e.g., no or mild pain, recurrence).
By compromising free movements of extraocular muscles in the orbits, orbital tumors can cause various degrees of ophthalmoplegia (▶ Fig. 13.51).
Fig. 13.51 a, b (a) Restriction of the eye movements in the left eye. There is very subtle left proptosis, and visual function is normal. (b) Axial T1-weighted magnetic resonance imaging of the orbits with contrast and with fat suppression showing a mass in the left orbit.
Trauma
Orbital trauma can restrict free movements of the globe in the orbit and often results in diplopia. Sudden orbital compression (e.g., tennis ball hitting the eye) can produce a blowout fracture of the orbital floor, entrapping the inferior rectus and other orbital tissue in the fracture. Elevation of the eye is restricted by the entrapped muscle, and depression of the eye is also generally poor because the muscle cannot constrict normally (fig ▶ Fig. 13.43).
Orbital fracture can also result in contusion of the extraocular muscle; hemorrhage in the extraocular muscle, with subsequent fibrosis; or orbital hemorrhage. Acute (and also sometimes delayed) enophthalmos and inferior orbital nerve damage (responsible for hypoesthesia and numbness of the inferior orbital rim and of the ipsilateral cheek) commonly accompany orbital floor fractures. A medial blowout fracture is also possible and results in entrapment of the medial rectus with an abduction deficit that may mimic a sixth nerve palsy (▶ Fig. 13.52).
Fig. 13.52 a, b (a) Left enophthalmos with mild periorbital ecchymosis and ptosis after trauma to the left orbit. (b) Coronal computed tomographic scan of the orbits without contrast showing a left orbital fracture (medial wall and floor). There is blood in the maxillary and ethmoid sinuses.
Other mechanisms of diplopia after head trauma include trauma to a cranial nerve (fourth nerve palsy most common, followed by sixth and third nerve palsies), carotid-cavernous fistula, brainstem lesion, subarachnoid hemorrhage with cranial nerve palsies, and raised intracranial pressure with sixth nerve palsy.
Giant Cell Arteritis and Orbital Ischemia
Chronic orbital ischemia (e.g., patient with common or internal carotid occlusion with poor collateral circulation, or patient with systemic vasculitis such as giant cell arteritis) can result in ischemia of an extraocular muscle, thereby producing diplopia, usually with pain. The diplopia is often transient and can be horizontal or vertical. At least 10% of patients with permanent visual loss from giant cell arteritis report having episodes of transient diplopia within the weeks preceding loss of vision (see Chapter ▶ 20).
Pearls
Giant cell arteritis needs to be ruled out in all elderly patients with transient or permanent diplopia.
Bony Deformation of the Orbit
Bony deformations change the shape of the orbit and also change the orientation of the orbital walls. In addition to compressing intraorbital structures, these changes modify the way the extraocular muscles move in the orbit and often produce diplopia.
Fibrous Dysplasia
Fibrous dysplasia is a benign bone condition in which normal bone is replaced by immature bone and osteoid in a cellular fibrous matrix. This results in expansion of the bone, further resulting in pain; deformation of the face, orbit, and cranium; and compression of adjacent structures (▶ Fig. 13.53). Diplopia is common secondary to displacement of the globe and of the ocular muscles, as well as cranial nerve palsies. Associated visual loss happens when there is compression of the optic nerve (usually at the level of the optic canal).
Fig. 13.53 a, b (a) Fibrous dysplasia with abnormal external appearance. Note the deformation of the left side of the face with elevation and distortion of the left orbit. (b) Axial computed tomographic scan of the brain (bone window) showing fibrous dysplasia involving the frontal bone and the cheeks.
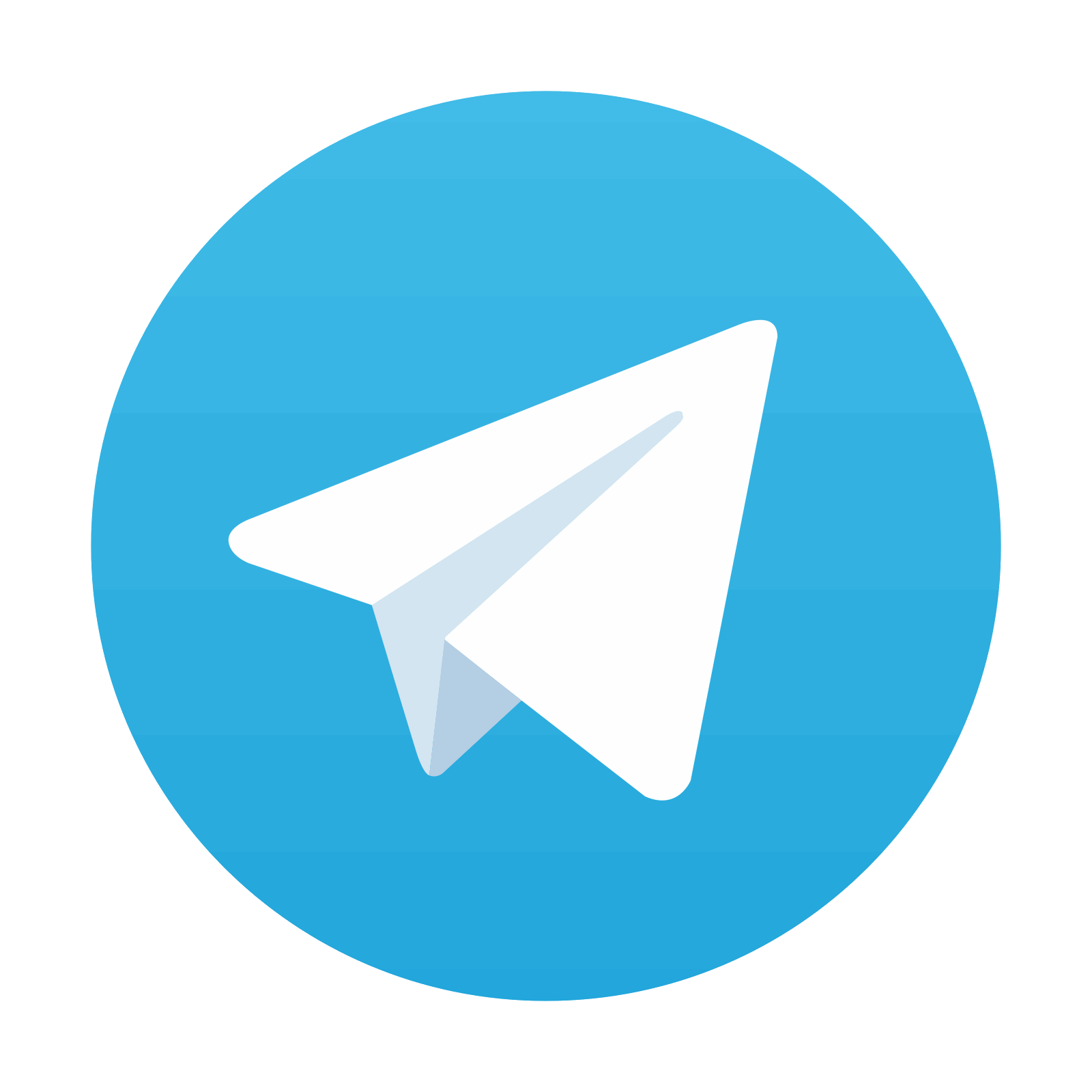
Stay updated, free articles. Join our Telegram channel

Full access? Get Clinical Tree
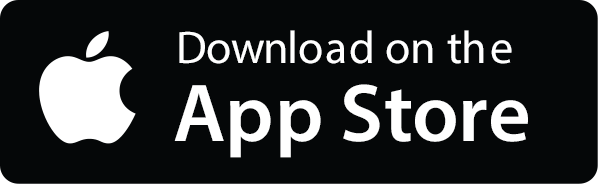
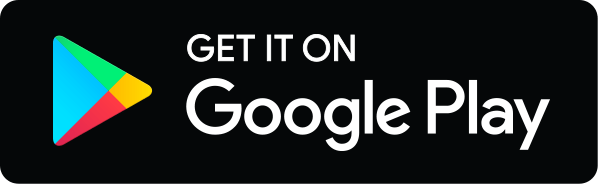