Fig. 1.1
Paracentral thinning of the cornea

Fig. 1.2
Fleischer ring (white arrow) and enlargement of corneal nerves (black arrow)

Fig. 1.3
Rizzuti sign

Fig. 1.4
Fleischer ring (arrows) seen with cobalt blue light (a, b). Note the correlation between Fleischer with axial curvature (c, d) and elevation maps (e, f) from the front surface

Fig. 1.5
Vogt striae (arrow)
Hydrops and scarring may also occur. Corneal hydrops occurs due a rupture in Descemet’s membrane, resulting in the sudden development of corneal edema. The break in the posterior cornea usually heals in 6–12 weeks, leading to stromal scarring, which may be beneficial in some cases. The final CDVA following the resolution of hydrops depends largely on the extent and location of the resulting corneal scar [6].
The following sections describe different corneal imaging approaches for KC assessment.
Corneal Topography
“Topography” derives from Greek words “to place” (topo) and “to write” (graphein), which means to describe a place. The term “corneal topography” has been classically used for the reconstruction of the front (anterior) corneal surface [20]. In the mid-1980s, Stephen D. Klyce, Ph.D., is recognized for first having developed algorithms for surface reconstruction of the acquired reflection image from Placido-based videokeratoscopy, allowing color-coded maps and quantitative data of the front surface of the cornea [21]. Corneal topography represented a true revolution in the diagnosis and management of corneal disease [20]. It has been found to be sensitive for detecting subclinical changes of KC prior to loss of CDVA and the development of typical slit lamp biomicroscopy findings [17]. The need for detecting these cases early in the disease process represents an unquestionable argument for topography to be considered as an essential test prior to laser vision correction (LVC) procedures since screening for such conditions is mandatory for avoiding major complication of keratectasia [3].
Like we already mentioned above, KC is classically defined as the topographic pattern of inferior steepening, but different patterns are also identifiable [22, 23] (Fig. 1.6). In general, higher corneal curvature values over 47.2 D are suspected cases of KC [22–24]. Additionally, the asymmetry between the values in the 3 mm radius in the upper and lower regions (or between the nasal and temporal regions) is suspected cases of KC when greater than 1.4 D. Such parameters are integrated in the calculation of the KISA index, described by Rabinowitz and Rasheed [25].


Fig. 1.6
Curvature patterns in KC
Corneal Tomography
The term “tomography” also derives from the Greek, as the combination of “to cut or section” (tomos) and “to write” (graphein). It is related to the three-dimensional (3-D) reconstruction of the cornea characterizing the elevation of the front and back surfaces of the cornea along with pachymetric mapping. Different technologies, such as horizontal slit scanning, rotational Scheimpflug, very-high frequency ultrasound, and optical coherence tomography (OCT) are available in many commercial instruments [20].
Elevation maps represent the difference from the examined corneal surface (anterior or posterior) compared to a chosen reference surface. Typically, the reference is calculated to have more coincident points (best-fit) with the examined surface [4, 26]. For ectasia screening purposes, our preference is to fix to the central 8 mm zone for calculating the best-fit sphere (BFS), since this zone is available for the majority of examined eyes. Concerning the elevation maps, different geometric bodies can be used as reference. The clinician should understand the impact of selecting different geometric bodies along with the zone diameter to calculate the best-fit [4]. For example, the BFS allows for the identification of astigmatism, while the best-fit toric ellipsoid (BFTE) facilitates the evaluation of irregular astigmatism. Interestingly, one study reported similar performances for the elevation values at the thinnest point of the posterior surface using BFS and BFTE (8 mm zone) [4].
The evaluation of the pachymetric distribution allows an understanding of the structural stability of the cornea. Since there is an increase in thickness from the center to the periphery, this gradual thickening ratio has a normal range. The ectatic cornea has changes in this pattern of pachymetric spatial distribution with a steeper increase of the thinned area to the periphery [27, 28].
The Belin-Ambrósio enhance ectasia display (BAD) is a comprehensive display that enables a global view of the tomographic structure of the cornea and is available on the Pentacam (Oculus Optikgeräte GmbH, Wetzlar, Germany). Deviation of normality values was implemented for the front (df) and back (db) enhanced elevations, thinnest value, pachymetric distribution (dp), and vertical displacement of the thinnest in relation to the apex (dy). The “d” values are calculated so that a value of zero represents the average of the normal population and 1 represents the value of one standard deviation towards the disease (ectasia) value. A final “D” is calculated based on a regression analysis that weights differently each parameter. Each parameter is indicated in yellow (suspicious) when it is ≥1.6 SD from the mean and turns red (abnormal) at ≥2.6 SD from the mean (Fig. 1.7). Values below 1.6 SD are reported in white and are viewed as within the normal range [4, 7, 29, 30].


Fig. 1.7
The D index in red showing ectasia (a–c) and suspicious in yellow (b–d)
Regarding KC diagnosis, previous studies have demonstrated higher accuracy of tomographic indices in comparison to front-surface derived parameters [31]. Corneal tomography has also proven to be more effective for enhancing specificity among patients with mild ectasia. For example, there are cases with subtle ectatic disease in which corneal topography appears normal since the ectatic change is not yet present on the front surface [20, 28, 30]. We refer to these patients as with high susceptibility or predisposition to developing ectasia, but they may be also referred as forme fruste keratoconus (FFKC), a concept introduced by Amsler in 1961 [32]. Clinical examples of such patients include the contralateral eyes with normal topographies from patients with very asymmetric KC and cases with natural progression of KC, which have been documented to earlier have normal anterior curvature exams, in the other eye. It is also essential to recognize that keratoconus suspect (KCS) is a topographic diagnosis and does not always imply an ectatic disease (i.e., lower specificity), while FFKC (ectasia susceptibility) is a pre-topographical condition, which may be present despite normal topography and central corneal thickness (CCT) [4, 24, 29].
Wavefront Analysis
KC creates significant visual problems derived from the corneal irregular astigmatism. Corneal aberration evaluation helps to describe the optical quality of the cornea and the measurement of the total ocular wavefront aberration provides a reliable tool to detect early KC and to follow its progression [33, 34]. It is important to note that each instrument will reconstruct Zernike terms differently, using smoothing functions from the acquired data. Thereby, the clinical guidelines for interpretation of the data should be used accordingly to studies from each instrument.
The anterior surface of the cornea is the most important refracting element of the eye. Corneal high-order aberrations are significantly increased in KC compared to normal corneas (Fig. 1.8). However, there are some discrepancies on the performance of such parameters to discriminate KC compared to normals. Gobbe and coworkers showed that the best detector to differentiate between suspected KC and normal corneas was vertical coma with a specificity of 71.9 % and sensitivity of 89.3 % [35]. Alio and Shabayek also proposed a modified Amsler-Krumeich classification for KC, which integrates corneal aberrometry, mainly from the magnitude of coma-like aberrations [36].


Fig. 1.8
Clinical example of a moderate keratoconus. Ocular aberrometry (a), Placido-disk based topography (b, d) and corneal tomography (c, e)
Total ocular wavefront was also analyzed in KC eyes. In a prospective observational case control study, Maeda and coworkers reported the corneal and ocular wavefront aberrations of normal and keratoconic eyes, using a Hartmann-Shack sensor combined to a Placido’s disc-based topography (KR-9000 PWc – Topcon, Corporation, Tokyo, Japan). There were significantly more total ocular higher-order (HOA) aberrations, such as coma, which was found to be 2.32 times higher than spherical-like aberrations in keratoconic eyes [37].
As mentioned before, this technology can assist refraction in KC patients. This approach also can improve CDVA in cases with advanced KC [19].
Segmental Tomography with Corneal Epithelial Mapping
The study of the epithelium thickness, which was only possible with very-high frequency ultrasound [38, 39], is now available with spectral-domain or Fourier-domain OCT [38, 40]. The epithelium reacts to underlying stromal protrusion in such a way that the knowledge of its thickness and pattern is very important for the evaluation of KC suspects or FFKC patients [38]. Analyzing the corneal epithelial thickness profile over a central 10-mm diameter area in a population of normal eyes, Reinstein demonstrated in normal eyes that epithelium is significantly thicker inferiorly than superiorly and also significantly thicker nasally than temporally [39]. The corneal epithelium has the ability to mask the presence of a stromal irregularity by changing its thickness profile. The epithelial pattern found in keratoconic eyes is distinct from the pattern found in normal corneas (Fig. 1.9). Known as the “doughnut pattern” of corneal epithelial compensatory changes, the epithelium appears to remodel to reduce the anterior stromal surface protrusion and to smooth the anterior corneal surface by thinning over the cone and thickening around the cone [38]. In mild KC, it is also possible to see a similar pattern with the thinnest epithelium point coincident with the point of maximum protrusion on the elevation maps. Therefore, in the presence of a normal front surface topography, an epithelial doughnut pattern often times can indicate the presence of very mild KC. As the disease becomes more severe, the difference between the thinnest and thickest epithelium increases. Thus, epithelial thickness profile changes with the progression of the disorder [38, 41].


Fig. 1.9
Example of a very asymmetric ectasia case with Placido-disk based topography (a, b), OCT segmental tomography (c, d) and corneal tomography (e, f)
Corneal Biomechanics
As an attempt to increase the accuracy for diagnosing corneal ectasia and its susceptibility, it is important to characterize the cornea beyond its geometry [42]. The biomechanical analysis can be performed with dynamic systems of noncontact tonometry, which monitor the deformation of the cornea by means of infrared reflection (Ocular Response Analyzer; Reichert Inc, Depew, NY) or by Scheimpflug imaging (Corvis ST; Oculus Optikgerate GmbH, Wetzlar, Germany) [43, 44].
The ocular response analyzer measures corneal hysteresis (CH) and corneal resistance factor (CRF) from the applanation pressures. Both CH and CRF have a statistically different distribution among normals and KC. However, there is a significant overlap among the groups for CH and CRF, which limits the value of such parameters for KC diagnosis [45, 46]. Interestingly, new parameters derived from the waveform signal of the corneal reflex during the noncontact tonometry (NCT) provide further biomechanical characterization resulting in a higher diagnostic performance.
The CorVis ST (Oculus Gmb, Wetzlar, Germany) uses an ultrahigh speed (UHS) Scheimpflug camera to monitor corneal response to the air pulse in an NCT system. Besides the intraocular pressure, this device provides the deformation amplitude, the radius of curvature at highest concavity, the applanation lengths, and the corneal velocities during the ingoing and outgoing phases [44, 47]. Studies comparing keratoconic and normal corneas found statistically significant differences for most parameters derived from this device, but again with a relatively high overlap among the groups, which limits diagnostic applications [5]. The combination of parameters using linear discriminant analysis and other artificial intelligence techniques has been the subject of intense studies by the Brazilian Study Group of Artificial Intelligence. For example, the “Corvis Factor 1” was efficient for enhancing the ability of distinguishing normals from ectatic corneas, including cases of FFKC (P < 0.001; Kruskall–Wallis test with Dunn’s post hoc test; Ambrósio et al. unpublished data 2011). There are already peer-reviewed published studies showing that corneal tomographic evaluation along with corneal biomechanical analysis lead to a more sensitive and specific methodology to detect KC.
Other tests such as the Brillouin microscopy revealed notable differences between healthy and keratoconic corneas in vivo and in vitro [48]. Interestingly, Brillouin imaging demonstrated that the mechanical weakening is focused within the area of the protrusion, while Brillouin shift was comparable with that of healthy corneas in the opposite area from the cone [48]. These findings go in agreement with the concept from Roberts and Dupps which proposes that there is focal biomechanical modification rather than an uniform generalized weakening, so that the focal reduction in elastic modulus precipitates a cycle of biomechanical decompensation [5].
Corneal biomechanics will be discussed in detail in Chap. 3.
Confocal Microscopy
Corneal confocal microscopy is a relatively new corneal imaging technique, which allows the study of corneal cellular structure. As a noninvasive procedure, it provides images of every corneal layer, providing to clinicians the possibility to investigate and to detect numerous corneal diseases at the cellular level [49].
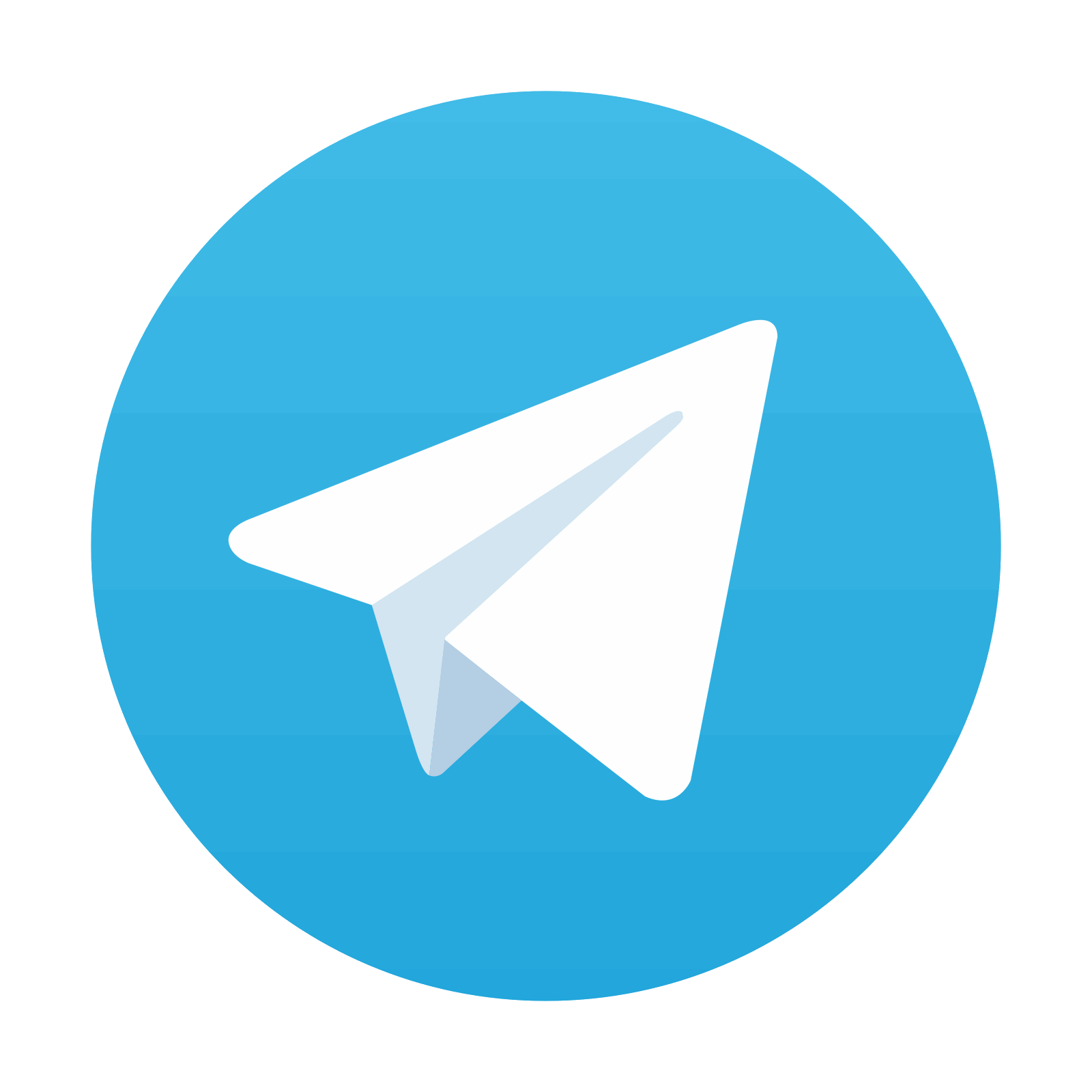
Stay updated, free articles. Join our Telegram channel

Full access? Get Clinical Tree
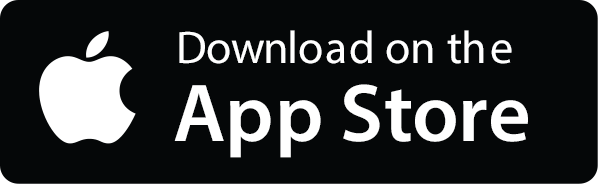
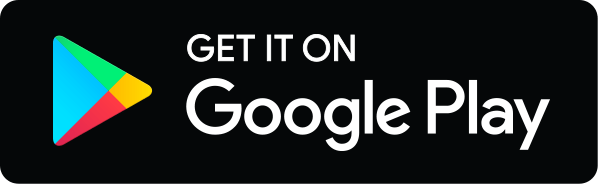