7 Electrophysiology and Miscellaneous Noninvasive Tests (Including Scanning Laser Ophthalmoscopy, Fundus Autofluorescence, and Adaptive Optics)
7.1 Introduction
Noninvasive testing of the retina is essential for the diagnosis of retinal disorders. Such noninvasive tests may include electrophysiologic testing, psychophysical testing, and other imaging techniques.
Electroretinography (ERG) and electrooculography (EOG) have classically constituted electrophysiologic testing. Dark adaptometry and color vision testing are forms of psychophysical testing. Both electrophysiologic and psychophysical testings are used to evaluate the functional integrity of the retina, and they are discussed in the following paragraphs. Visually evoked cortical potential (VECP) recording is a technique used to measure a gross electrical signal generated at the visual cortex in response to visual stimulation and, therefore, records activity of the entire sensory visual pathway from the retina to the occipital cortex. Because it is administered infrequently by ophthalmologists, VECP will not be discussed here. Imaging modalities such as scanning laser ophthalmoscopy (SLO), fundus autofluorescence (FAF), and adaptive optics (AO) are newer techniques that are useful for the diagnosis and monitoring of a variety of retinal conditions, and they will be discussed in this chapter.
7.2 Electroretinography
ERG measures the electrical response generated by the retina following stimulation with light. The two most commonly used types of ERG are full-field ERG and multifocal ERG (mfERG). Full-field ERG employs diffuse illumination to elicit a response from the entire retina. Ganzfeld dome stimulation illumination remains the standard technique for eliciting a full-field ERG response. mfERG utilizes a flickering array of hexagonal elements to generate multiple (typically 61 or 103) local ERG responses within the cone-dominant retina; a topographic map which reflects local ERG responses is then created. Other specialized forms of ERG which are beyond the scope of this discussion include macular or focal ERG, pattern ERG, early receptor potential, scotopic threshold response, direct-current ERG, long-duration light-adapted ERG, double-flash ERG, chromatic stimulus ERG, dark-adapted and light-adapted luminance-response analyses, and saturated a-wave slope analysis.
7.2.1 Full-Field Electroretinography
Introduction
Full-field ERG measures the summative electrical response generated by the whole retina following exposure to a light stimulus. Full-field ERG responses are described according to two principal parameters: (1) state of light adaptation and (2) strength of flash stimuli. 1 The International Society for Clinical Electrophysiology of Vision (ISCEV) has standardized full-field ERG to include a total of five responses 2 :
Dark-adapted 0.01 ERG (rod response)
Dark-adapted 3.0 ERG (combined rod–cone response; maximal response)
Dark-adapted 3.0 oscillatory potentials
Light-adapted 3.0 ERG (cone response)
Light-adapted 3.0 flicker (30-Hz flicker)
Practical Considerations
Pupillary dilation should be performed prior to ERG testing, and maximal pupil size should be noted before testing begins. Dark adaptation for a period of at least 20 minutes should be achieved by darkening the room and placing multiple adhesive patches over both eyes to achieve total occlusion of light. Ideally, it is best to avoid ERG testing following fluorescein angiography and fundus photography, but if this is not possible, dark adaptation for at least 60 minutes should be performed following photography.
After a topical anesthetic is placed on the eyes, “active” electrodes that contact the cornea or limbal bulbar conjunctiva should then be placed underneath the eyelids; this can be performed under dim red illumination. Some consider contact lens electrodes such as the Burian-Allen or ERG-jet electrodes to provide the most stable recordings, but these also require use of nonviscous ionic conductive solution (no more than 0.5% methyl cellulose). Alternative electrode materials include conductive fibers and foils, conjunctival loop electrodes, and corneal wicks. A single ground electrode is placed with electrode paste either on the patient’s forehead or ear. A reference or “inactive” electrode may be contained within each contact lens–speculum unit or may be placed temporally to the lateral orbital rims. The “active,” ground, and reference electrodes all connect to a junctional box. The difference in voltage between the “active” corneal electrode and the reference electrode is then recorded by a differential amplifier.
The Grass xenon arc photostimulator with flash duration of 10 µs has been commonly used to obtain ERGs over a range of stimulus intensities. A Ganzfeld dome is necessary to standardize the white flash stimulus for full-field ERG. 3 The strength of flash stimuli, as measured at the surface of the Ganzfeld dome, is measured in units of candela-seconds per meter squared ([cd·s]/m2). Although chromatic stimuli may be used in certain cases, white flashes should be used for standard ERG. Protocol dictates whether a stimulus may be repeated and, if so, at what interval between stimuli.
Under scotopic conditions, a series of ERG recordings is obtained using different light intensities, denoted by the number ([cd·s]/m2) in the ERG description: 0.01 ERG, 3.0 ERG, and 3.0 oscillatory potentials. The patient is then light-adapted, which is defined as exposure to white background luminance of 30 candela per meter squared (cd/m2) for at least 10 minutes, and this standardized luminance is delivered using the Ganzfeld dome. Under photopic conditions, the 3.0 ERG and 3.0 flicker are then completed.
The full-field ERG can be affected by a variety of conditions unrelated to the function of the retina. It is important to be aware of these confounding variables when establishing the normalcy or abnormalcy of an ERG test result. The type and position of the corneal electrode being used can affect the quality of the ERG. Media opacities, including cataract, vitreous hemorrhage, and vitreous debris, can cause both a reduction in ERG amplitude and a prolonged implicit time. Age and myopia can decrease the ERG amplitude but will not typically affect implicit time. Pupil size can also affect the amplitude of the ERG and may account for the decline in ERG amplitude associated with age. Patient compliance and the presence or absence of sedation or anesthesia may also impact test results and should, therefore, be documented.
Purpose
Full-field ERG testing is particularly useful in determining the abnormalcy of what appears to be a “normal” retina on examination. ERG testing may elucidate whether visual dysfunction can be attributed to rods, cones, or both, and it can readily document the extent of visual dysfunction while tracking changes over time. In this fashion, ERG testing can distinguish between stationary forms of night blindness and progressive degenerations such as retinitis pigmentosa. ERG may prove essential in establishing the etiology of retinal degeneration due to hereditary, toxic-metabolic, retinal vascular, or inflammatory etiologies. ERG testing may also be particularly instrumental in investigating specific patient complaints such as difficulty with peripheral vision, unexplained loss of central vision, and night blindness. Additionally, full-field ERG testing may help establish underlying retinal function in pediatric or nonverbal patients as well as in eyes in which the media is obscured.
Beyond its use in establishing pathologic conditions, ERG also reassures both the patient and physician when normalcy of retinal function is demonstrated. In such cases, alternative explanations for visual loss such as the optic nerve, central nervous system, and nonorganic visual loss should be entertained.
While instrumental in defining loss of function, a proper diagnosis can only be made when ERG testing is performed in conjunction with a complete ophthalmic examination and thorough medical evaluation. Genetic testing may be a useful adjunct in further classifying certain degenerative conditions.
Special Considerations
Only in conjunction with a careful history and ocular examination does full-field electroretinographic testing provide for a complete evaluation and accurate diagnosis.
Interpretation
A normal ERG consists of three major components: a-wave, b-wave, and c-wave. The photoreceptors generate the initial, cornea-negative component or a-wave of the ERG, and the Müller’s cells and bipolar cells are responsible for the later, cornea-positive b-wave of the ERG. The c-wave appears to be generated from the retinal pigment epithelium (RPE), although it also depends on rod photoreceptor activity. Measurement of the c-wave is difficult and highly variable; thus, it has very limited clinical application.
The character of the waveform should be examined closely and compared with an example of normal ERG, ideally one generated by the same ERG system. An identifiable a-wave and b-wave may not always be distinctly present, but when possible, the amplitude and implicit time of the a-wave and b-wave should be measured. The a-wave amplitude is measured from baseline to a-wave trough. The b-wave amplitude is measured from a-wave trough to b-wave peak. Implicit time for a-wave and b-wave is measured, respectively, from the time of the flash to the trough of the a-wave and to the peak of the b-wave. Physiological significance should be taken into account in all cases, also bearing in mind the possibility of erroneous measurements due to poor electrode contact with the cornea or improper calibration.
Normal values for ERG results depend on stimulus conditions and patient variables, as discussed earlier. These considerations need to be factored into proper interpretation of an ERG. As an approximation, a bright white flash intensity should result in a dark-adapted b-wave amplitude of at least 350 µV in patients younger than 50 years and with normal axial lengths. The normal amplitude of the 30-Hz flicker stimulus is at least 50 µV. For patients with dramatic visual dysfunction, computer-averaging techniques and narrow bandpass filtering allow for the detection of ERG responses below 1 µV.
When evaluating patients with retinal degeneration, it is important to quantify the function of both the rod and cone systems. The full-field ERG is used to establish generalized loss of rod or cone function, or both. In general, diseases associated with predominantly abnormal rod responses include congenital stationary night blindness, early retinitis pigmentosa, rod dystrophies, and rod–cone dystrophies. Diseases associated with predominantly abnormal cone responses include achromatopsia (both rod monochromatism and blue cone monochromatism), cone dystrophies, and cone–rod dystrophies. Conditions such as advanced retinitis pigmentosa, Leber’s congenital amaurosis, gyrate atrophy, choroideremia, total retinal detachment, and retinal aplasia cause dramatic abnormalities in both cone and rod function.
The rod-isolated response is achieved by dark-adapting the patient, and a dim white (or blue, although this is not a part of the standardized recommendations) flash below cone threshold is used to stimulate the retina, resulting in a waveform that has almost no detectable a-wave but a large b-wave (Fig. 7-1a). Using a bright white flash in the dark-adapted state causes maximal stimulation of both rods and cones and results in large a- and b-waves with oscillatory potentials in the ascending b-wave (Fig. 7-1b). Although this combined rod–cone response is both rod and cone mediated, 80% of this response is attributable to rods, and the remaining 20% results from cones. Thus, if cone dysfunction is mild, the combined scotopic rod–cone response waveform, amplitude, and implicit time may all appear normal. Oscillatory potential (Fig. 7-1c) is also assessed in a state of dark adaptation, but oscillatory potentials are less critical in assessing rod function. Rather, oscillatory potentials are believed to be the result of inhibitory influences effected by amacrine cell input on the b-wave and are characteristically diminished when retinal ischemia is present. Reduced oscillatory potentials may also be associated with some forms of congenital stationary night blindness.

Cone responses are obtained in a light-adapted state, as light adaptation suppresses rod responses such that they do not contribute to the waveforms generated by the 3.0 ERG and 3.0 flicker (30-Hz flicker) (Fig. 7-1d, e). Both the 3.0 ERG and 3.0 flicker use a white flash at a strength of 3.0 ([cd·s]/m2). A red flash may also be used to isolate a cone response, but this is not a part of standardized recommendations. Mild reductions in cone amplitudes may be observed when macular scarring covers an area of at least four disc diameters, although the scotopic 0.01 ERG, 3.0 ERG, and 3.0 oscillatory potentials will be affected minimally in such cases of macular disease because of the disproportionate number of rods as compared to cones in the human retina.
Pearls
To observe a reduction in a-wave or b-wave amplitude, a large area of retinal tissue must be affected. Isolated macular disorders do not alter the full-field electroretinogram. Optic nerve disorders and cortical conditions likewise do not affect the full-field ERG.
Many conditions display loss of retinal function. Therefore, a careful history and ophthalmoscopic examination are necessary for establishing a diagnosis in conjunction with ERG testing. Testing retinal function is critical when considering the diagnosis of the following conditions (see the following list): hereditary retinal degenerations (such as retinitis pigmentosa, cone–rod dystrophies, stationary forms of night blindness), metabolic disorders, acquired retinal ischemia (due to retinal artery occlusion, retinal vein occlusion, or diabetic retinopathy), toxic retinopathy (such as siderosis or medication-related toxicity: chloroquine, hydroxychloroquine, chlorpromazine, thioridazine, quinine), autoimmune/paraneoplastic retinopathy (including cancer-associated retinopathy and melanoma-associated retinopathy), vitamin A deficiency, retinal detachment, media opacities, and unexplained vision loss.
Disorders Affecting Full-Field Electroretinography
Photoreceptor degenerations
Stationary night blindness
Hereditary vitreoretinopathies
Retinal artery and vein obstructions
Diabetic retinopathy
Toxic retinopathies
Cancer-associated retinopathy
Vitamin A deficiency
Retinal detachment
Media opacities, including cataract and vitreous hemorrhage
Laboratory testing is often needed to confirm the presence of an inherited or acquired metabolic condition (e.g., hypovitaminosis A) or to rule out the possibility of cancer-associated retinopathy. Genetic testing may be useful to confirm the presence of an inherited retinal degeneration.
A comprehensive discussion of characteristic ERG abnormalities is beyond the scope of this chapter, but a few representative points are discussed here. In early retinitis pigmentosa, individuals may display delayed cone b-wave implicit times suggestive of more diffuse retinal dysfunction; b-wave amplitudes are variably affected in early stages of retinitis pigmentosa. 4 Cases of advanced retinitis pigmentosa are usually accompanied by markedly reduced rod and cone responses to a bright flash (Fig. 7-2a) and markedly reduced cone responses to a 30-Hz flicker as well as a light-adapted 3.0 ERG (Fig. 7-2b). The ERG of patients with advanced choroideremia may similarly show striking reduction in rod and cone responses to a bright flash (Fig. 7-2c).

Pearls
Delayed cone implicit times are suggestive of widespread retinal disease even when cone amplitudes are normal.
The term “negative ERG” specifically refers to an abnormal waveform variant of the combined rod–cone response in which the amplitude of the b-wave is smaller than that of the a-wave (Fig. 7-2d). A negative ERG can be recorded in the setting of acquired severe retinal circulatory disturbance (such as central retinal artery occlusion, ischemic central retinal vein occlusion, or proliferative diabetic retinopathy), acquired retinal diseases (such as melanoma-associated retinopathy, birdshot choroidopathy, ocular siderosis, quinine retinopathy, or methanol toxicity), or inherited retinal diseases (such as complete- and incomplete-type congenital stationary night blindness, X-linked juvenile retinoschisis, juvenile-onset neuronal ceroid lipofuscinosis, Goldmann–Favre’s syndrome, and infantile Refsum’s disease). 5 An extinct combined rod–cone response can be seen in advanced stages of retinitis pigmentosa, gyrate atrophy, choroideremia, and cancer-associated retinopathy.
ERG testing can play a role in the evaluation of retinal ischemia resulting from central retinal vein obstruction and diabetic retinopathy; it may be useful in deciding which patients with these conditions are the best candidates for panretinal laser photocoagulation in attempting to prevent iris or retinal neovascularization. ERG can also be helpful in differentiating an old central retinal artery obstruction from an old optic neuropathy. The ERG will show a reduced b-wave on the affected side with the former condition but be totally normal with the latter condition.
7.2.2 Multifocal Electroretinography
Introduction
Sutter and Tran developed the multifocal ERG (mfERG) in 1992, and this enabled the measurement of multiple isolated focal ERG responses. 6 In contrast to full-field ERG, mfERG measures retinal responses generated by the 40 to 50 degrees surrounding central fixation. This translates into a focus on cone-predominant retina within the macula. Depending on the clinical indication, mfERG may represent a useful primary or adjunctive test for measuring macular function. When conditions such as hydroxychloroquine toxicity, Stargardt’s disease, acute zonal occult outer retinopathy, and occult macular dystrophy are suspected, mfERG is helpful for diagnosis.
Practical Considerations
mfERG is performed on light-adapted patients after pupillary dilation. Refractive error should be taken into account prior to beginning testing, and, if need be, appropriate correction should be provided. It is best not to perform mfERG following ophthalmoscopy or fundus photography, but a recovery period of 15 minutes after either may be used if necessary. mfERG is performed on one eye at a time, and the eye not being tested should be patched to minimize blinking.
After topical anesthetic is instilled, active, reference, and ground electrodes are placed in the fashion described for full-field ERG. As is the case for full-field ERG, the active electrode must contact the cornea or adjacent bulbar conjunctiva, and contact lens, foil, or fiber electrodes may be employed.
Either a cathode ray tube or a liquid crystal display may be used to generate the mfERG stimuli. The stimuli consist of alternating light (white) and dark (black) hexagonal elements that are smallest centrally (surrounding fixation) and larger peripherally within the overall arrangement. The luminance of the light elements should be at least 100 cd/m2, with the dark elements dim enough to provide at least 90% contrast. 7 At any point in time, approximately 50% of the hexagonal elements are illuminated. Traditionally, either 61 or 103 element arrays are used, with testing of one eye taking at least 4 minutes to complete when 61 elements are being used and at least 8 minutes to complete when 103 element arrays are being used. Testing with 103 element arrays provides better spatial resolution than testing with 61 elements, but it takes longer to perform and results in a decreased signal-to-noise ratio.
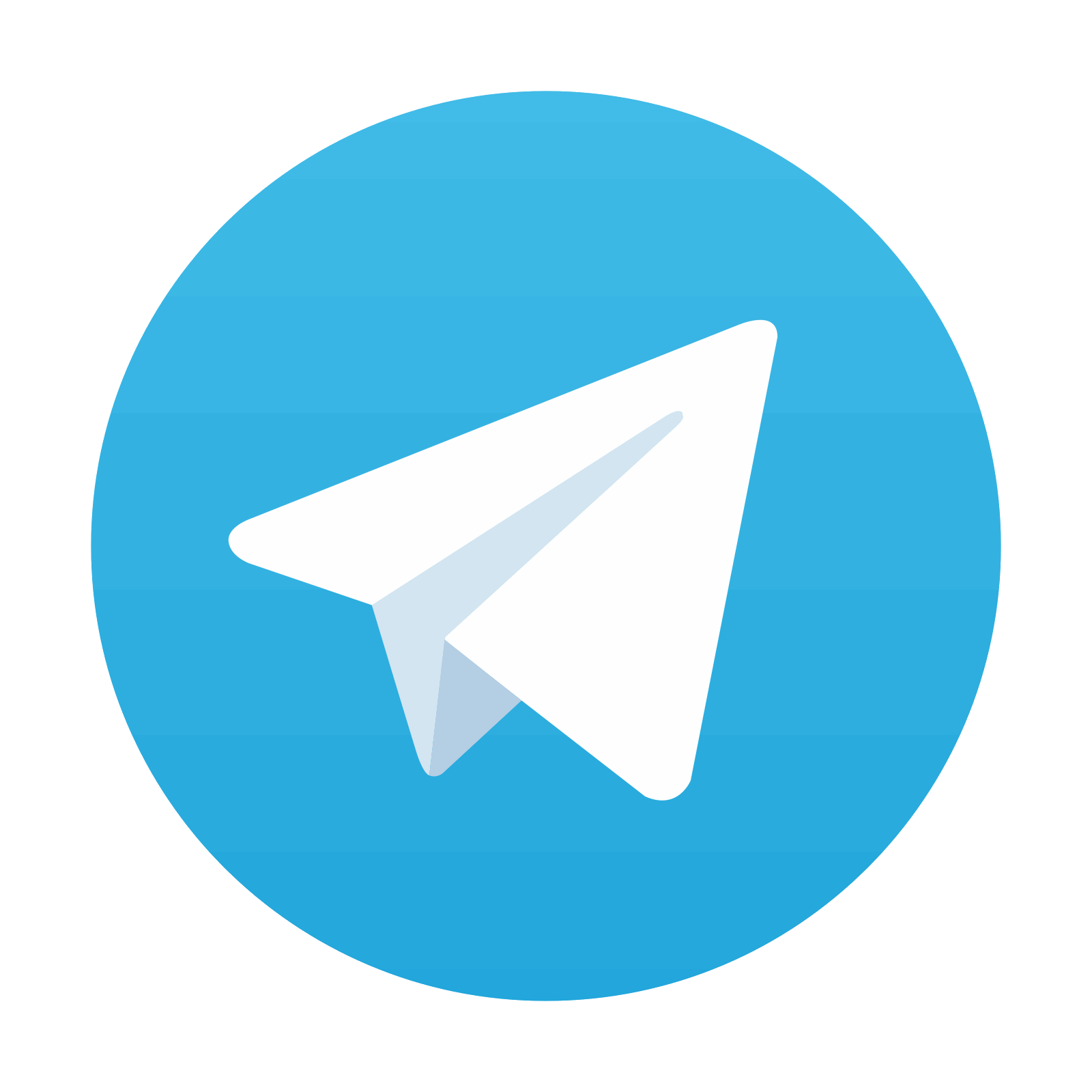
Stay updated, free articles. Join our Telegram channel

Full access? Get Clinical Tree
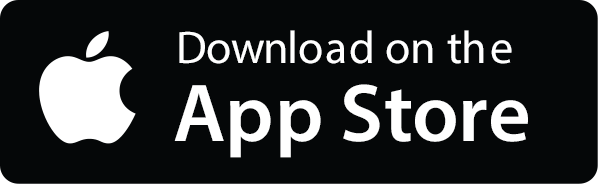
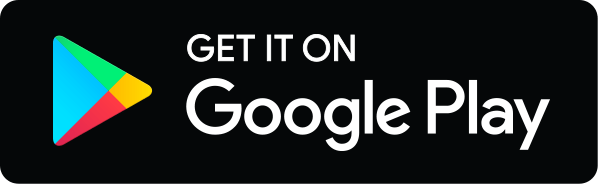
