(1)
Department of Otlaryngology Head and Neck Surgery, Graduate School of Medical Sciences Kumamoto University, Kumamoto, Japan
Abstract
Three-dimensional (3D) computed tomography is used to assess the 3D configuration of the laryngeal lumen. We propose a novel classification of 3D glottal configuration, in which the thickness of the affected vocal fold during phonation is the key determinant. In the type A configuration, the thickness is nearly equal to that of the unaffected vocal fold. In the type B situation, the affected fold is thinner than the other fold during both phonation and inhalation. In the type C condition, the affected fold is thinner than the other during phonation but, paradoxically, exhibits adduction and an increase in thickness during inhalation. Vocal function in patients with the type A configuration is significantly better than in those with type B or C configurations, whereas no significant difference in vocal function is evident between patients with configurations of types B and C. Over-adduction of the unaffected vocal fold may not compensate for vocal function. Further studies are necessary to associate glottal configuration types with reinnervation patterns evident upon electromyographic analysis and to determine why some patients with unilateral vocal fold paralysis exhibit over-adduction of the unaffected fold during phonation whereas others do not, despite the presence of a posterior glottal gap.
Keywords
Three-dimensional computed tomographyGlottal configurationVocal functionOver-adduction of the unaffected vocal foldElectromyographic recruitment4.1 Introduction
The presence of unilateral vocal fold paralysis (UVFP), determined by videoendoscopy of the larynx, has prompted a search for the underlying cause. The investigations routinely performed at the author’s institution are shown in Fig. 2.1. When the cause was established, treatment followed. Later, the symptoms of VFP1 were addressed. This chapter will deal with the diagnostic battery available for patients with breathy dysphonia caused by UVFP. In addition, the clinical characteristics of UVFP studied at the author’s institution will be described.
4.2 The Vocal Function Test Battery
Voice production is a complex process that involves appropriate coordination of many organs. Respiratory organs provide airflow, which is the driving force behind vocal fold vibration. The vocal fold plays an essential role in the production of glottal sound. Such sound is resonated as the sound traverses the vocal tract and is finally radiated through the mouth and nose. In this book, the laryngeal aspects of sound production are discussed; it will be assumed that the respiratory and articulatory systems are functioning well.
Figure 4.1 shows a flow chart of the vocal function tests in the test battery administered to UVFP patients in the author’s institution. First, history taking explores the patient’s occupation and hobbies, which may be associated with vocal usage. Also, the patient’s attitude to breathy dysphonia is subjectively assessed using a voice handicap index (VHI [1], VHI-10 [2]) and a voice-related quality-of-life (V-RQOL) instrument [3]. Videostroboscopy is the most useful tool for observation of vocal fold vibration. Figure 4.2 shows the items that must be evaluated videostroboscopically. Although the technique does not permit analysis of every vibratory cycle, average patterns of vocal fold vibration can be observed. However, if the voice is severely breathy, strobe illumination is not synchronized and irregular mucosal movement is observed. Furthermore, in-depth information such as differences in the thicknesses of and the vertical distance between the vocal folds is not readily obtainable using this method.



Fig. 4.1
Flow chart showing how to administer a vocal function test battery to patients with unilateral vocal fold paralysis

Fig. 4.2
Parameters to be evaluated during videostroboscopy
The aerodynamics of voice production involve differences between subglottal and supraglottal pressures. Imperfect closure of the glottis during phonation by UVFP patients decreases glottal resistance, elevates supraglottal pressure, and reduces the vocal efficiency index. [4] Such glottal insufficiency is reflected by an increase in the mean airflow rate (MFR) and a reduction in maximum phonation time (MPT), as measured via sustained vowel phonation at a comfortable pitch and loudness. A voice profile is also obtained to detect pathological changes in the voice for which other parameters remain within normal ranges. Titze [5] originally defined a voice range profile as a comparison of vocal intensity versus fundamental frequency (F0). The voice profile most commonly used in Japan displays not only vocal intensity versus F0 but also a combination of three parameters: intensity, F0, and MFR. [6] The auditory impression of dysphonia is evaluated using the GRBAS scale, which is an acronym for grade overall, rough, breathy, asthenic, and strained [7]. Objective voice assessment evaluates acoustic parameters including jitter [8], shimmer [9], and the harmonics-to-noise ratio (HNR) [10].
4.3 Image Analysis of Unilateral Vocal Fold Paralysis
Videostroboscopy of the larynx cannot be used to assess differences in the thicknesses of and the vertical distance between the vocal folds. Hong and Jung [11], using stroboscopic images, evaluated the height of the affected fold compared to that of the unaffected fold based on the contact pattern of the vocal processes and the brightness of the vocal fold during phonation. It was found that the height of the affected fold was nearly equal to that of the unaffected fold when the affected fold was located at the midline. Furthermore, they reported that in half of UVFP patients in whom the affected fold was located off the midline, the affected fold was lower than the other. Upon analysis of stroboscopic images, Inagi et al. [12] found that UVFP patients exhibiting off-plane vocal fold closure during phonation developed hyperfunctionality (increased effort to compensate for the deficit). The cited authors stressed the importance of the shape of the subglottis and the glottal plane of closure, in determining convergence of the vocal fold during phonation. Gray et al. [13] also emphasized the importance of the infraglottal shape in terms of ease of phonation. Inagi et al. [12] suggested that radiographic techniques should be used to complement videostroboscopy to allow depth information to be obtained more readily.
Isshiki and Ishikawa [14] used conventional X-ray tomography to observe the laryngeal lumen during both phonation and inhalation in patients with UVFP. They found that the affected vocal fold was thinner than the healthy fold in 38 % of patients and that in 46 % of patients the affected vocal fold was higher during phonation. Although differences in the vertical thicknesses of and the distance between vocal folds were assessed, they failed to relate the findings with vocal function. Computed tomography (CT) and magnetic resonance imaging (MRI) have also been used to assess the persistence of fat injected into the vocal fold [15–17] and to help design the window position of and the implant size required for type I thyroplasty [18]. However, the authors of the cited reports did not investigate vocal fold configuration during phonation. Yumoto et al. [19, 20] were the first to successfully use a 3D CT endoscopic technique to assess the normal laryngeal structure and pathological changes in patients with laryngeal cancer, UVFP, and vocal fold atrophy. Laryngeal images viewed from both the oral and tracheal sides were obtained, as were two vertically split hemilaryngeal images. With the exception of images from the oral side, reconstructed images cannot be obtained via endoscopic observation. It was found that a combination of 3D endoscopic and coronal multiplanar reconstruction (MPR) imaging yielded more diagnostic information on patients with UVFP than did axial images alone, although scanning was performed during breath hold; the report was inconclusive in terms of vocal function. The later development of multi-slice helical CT allowed high-speed data acquisition without affecting the resolution of the final image. The associated short scan times permit the use of the method for observing laryngeal structures in 3D during both phonation and inhalation [21–23]. The details of how the larynx is scanned to produce 3D images will be described in the next section.
4.3.1 Three-Dimensional Computed Tomography (3D CT)
4.3.1.1 Production of 3D Endoscopic and Coronal MPR Images
Figure 4.3 shows the body position of and the task performed by a patient, the model used for scanning, the features thereof, the method used to detect the mucosal surface of the laryngeal lumen, and the images that are produced. Each subject is scanned during phonation and, after a short rest, during inhalation. Pre-scanning practice is necessary to enable satisfactory task performance. Axial images are generated at 1-mm intervals by overlapping each adjacent image by 0.5 mm, and the images are sent to a workstation together with voxel data. A volume-rendering technique is used in the 3D endoscopic mode. Supraglottal structures evident in the oral view are removed to enable visualization of the vocal folds when supraglottal constriction occurs [21–23].


Fig. 4.3
Body position of, and task performed by, a subject; the model utilized for scanning; the setting thereof; the method used to examine the mucosal surface of the laryngeal lumen; and images produced with the aid of multi-slice helical computed tomography
The CT dose index (CTDi) is calculated to estimate the approximate radiation dose given during the laryngeal scan. The total CTDi value of scanning during both phonation and inhalation is 25.45 milliGray (mGy). This value was compared to those associated with the scanning of other regions of the head and neck. The CTDi values for the paranasal sinuses and temporal bone, based on the settings used at Kumamoto University Hospital, are 30.25 mGy and 63.57 mGy, respectively. A temporal bone scan requires the collection of thin slices (0.5 mm thick), and the CTDi is more than double that associated with a laryngeal scan. Although the CTDi is relatively low, care should nonetheless be taken when scanning the larynges of UVFP patients.
4.3.1.2 3D Endoscopic and Coronal MPR Images from Patients Exhibiting Normal Vocal Fold Movement
Figures 4.4 and 4.5 show endoscopic and coronal MPR images from a 55-year-old male exhibiting sulcus vocalis during both inhalation and phonation. His vocal fold movement was normal. Abduction of the vocal folds during inhalation and adduction during phonation were apparent on CT endoscopic images. During inhalation, the vocal folds were barely recognizable in either hemilaryngeal or coronal MPR images. The oral and tracheal views revealed that the vocal processes met at the midline during phonation. Coronal MPR images revealed that the vocal folds were symmetrical in terms of both thickness and the contours of the subglottic vault during phonation.



Fig. 4.4
CT endoscopic and coronal multiplanar reconstructed images of a 55-year-old male with sulcus vocalis and normal vocal fold movement, taken during inhalation. A Oral view, B Tracheal view, C Left hemilarynx viewed from the right, D Right hemilarynx viewed from the left, R Right, L Left, Ant Anterior, Post Posterior, PS Pyriform sinus (Citation: Ref. [22])

Fig. 4.5
CT endoscopic and coronal multiplanar reconstructed images taken during phonation of the subject mentioned in Fig. 4.4. A Oral view, B Tracheal view, C Left hemilarynx viewed from the right, D Right hemilarynx viewed from the left, R Right, L Left, Ant Anterior, Post Posterior, VF Vocal fold (Citation: Ref. [22])
4.3.1.3 Representative Images of Unilateral Vocal Fold Paralysis
Figure 4.6 shows a representative UVFP case in whom the affected fold was as thick as the other fold and was located at the same level during phonation. The affected fold was directed medially, not superomedially, during phonation (Fig. 4.6, thin arrow), and remained adducted during inhalation (Fig. 4.6, thick arrow). A small glottal gap was evident between the vocal processes during phonation. These images suggest that synkinetic innervation was in play: the TA/LCA complex seemed to have received regenerated nerve fibers that originally innervated the posterior cricoarytenoid (PCA) muscle, because TA/LCA muscle contraction was evident during inhalation.


Fig. 4.6
CT endoscopic and coronal multiplanar reconstructed images of a 66-year-old female taken 7 months after onset of right vocal fold paralysis caused by thyroid cancer surgery. A Images taken during inhalation, B Images taken during phonation, *Affected side (Citation: Ref. [24])
Figure 4.7 shows a representative case in whom the affected fold was thinner and was located at a higher level than the healthy fold during phonation (thin arrow). Over-adduction of the healthy vocal fold was obvious during phonation. The affected fold remained thin and was directed superomedially during both phonation (thin arrow) and inhalation (thick arrow). A wide glottal gap between the vocal processes was evident during phonation, at which time the vocal folds could not be visualized videostroboscopically because of anterior-to-posterior constriction. Furthermore, the arytenoid portion of the healthy fold moved anteromedially during phonation, obstructing the view of the affected side (Fig. 4.8).



Fig. 4.7
CT endoscopic, coronal multiplanar reconstructed, and videostroboscopic images of a 68-year-old male taken 11 months after onset of right vocal fold paralysis caused by surgery to treat esophageal cancer. A Images taken during inhalation, B Images taken during phonation, *Affected side (Citation: Ref. [24])

Fig. 4.8
Videostroboscopic images taken during inhalation (left) and phonation (right) by a 68 year-old male 11 months after onset of right vocal fold paralysis caused by surgery to treat esophageal cancer. This is the same subject as featured in Fig. 4.7, *Affected side, Ary Arytenoid portion on the affected side (Citation: Ref. [24])
Figure 4.9 shows a representative case in whom the CT endoscopic and coronal MPR images were suggestive of the presence of paradoxical vocal fold movement. The affected fold was abducted during phonation and was barely recognizable in the coronal image. Furthermore, the fold was adducted (black arrows) and was thick during inhalation (thin white arrow). A wide glottal gap was evident between the vocal processes during phonation. Figure 4.10 shows videostroboscopic images taken during inhalation and phonation. The vocal process on the affected side could not be visualized during inhalation because of anterior tilting of the arytenoid portion. However, CT endoscopic and coronal images revealed that the affected fold was positioned near the midline during inhalation (Fig. 4.9, left). Thus, the location of the vocal fold could not be assessed in the absence of videostroboscopic visualization of the vocal process.



Fig. 4.9
CT endoscopic and coronal multiplanar reconstructed images of a 66-year-old male taken 13 months after onset of left vocal fold paralysis caused by surgery to treat thyroid cancer. A Images taken during inhalation, B Images taken during phonation, *Affected side (Citation: Ref. [24])

Fig. 4.10
Videostroboscopic images taken during inhalation (left) and phonation (right) of a 66-year-old male 13 months after onset of left vocal fold paralysis caused by surgery to treat thyroid cancer. This is the same subject as featured in Fig. 4.9, *Affected side, Ary Arytenoid portion of the affected side
4.3.2 Glottal Configuration During Phonation and Vocal Function
Videostroboscopy is the standard means used to evaluate the vocal folds of patients with UVFP. Glottal configuration varies in such patients. However, determination of the 3D configuration of a unilaterally paralyzed larynx during phonation remains diagnostically challenging. Such an examination should establish the location of the affected fold, the presence or absence of fold flaccidity, and the extent of the glottal gap during phonation. However, supraglottal constriction, specifically over-adduction of the ventricular fold, anterior-to-posterior shortening of the glottis, and anterior tilting of the arytenoid portion, may occur during phonation. These factors prevent visualization of the entire larynx, especially the vocal process and posterior glottis. Furthermore, videostroboscopy cannot be used to evaluate differences in the thicknesses and vertical positions of the vocal folds. Such in-depth information is important when seeking to determine the extent of convergence of the vocal folds during phonation [12].
This section will describe the glottal configuration of UVFP patients based on the thicknesses and locations of the vocal folds during both phonation and inhalation, utilizing 3D CT imaging. We propose a novel classification of 3D glottal configuration, which features four grades, each of which is compared to the associated vocal functionality [24]. Such a classification may enhance our understanding of glottal configuration in UVFP patients in terms of vocal function.
4.3.2.1 Subjects, Evaluation of Glottal Configuration, and Assessment of Vocal Function
Fifty-nine consecutive patients with paralytic dysphonia, who had experienced UVFP onset more than 6 months prior, underwent 3D CT between February 2002 and January 2010. Of the 59 patients, 22 were excluded. The exclusion criteria were poor respiratory or swallowing function (which might interfere with sustained phonation) in eight patients, poor performance of the phonatory or inhalatory task during CT scanning in seven patients, intracranial lesions in four patients, a past history of phonosurgery in two patients, and unknown time of UVFP onset in one patient. Thus, 37 patients were included in the study. Table 4.1 summarizes the demographic characteristics thereof, and the causes of UVFP are listed in Table 4.2.
Table 4.1
Subject profiles
Gender | 24 males, 13 females |
Age | 27–82 years (56.6 ± 15.0) |
Affected side | Left: 26, right: 11 |
Period from onset to CT scanninga | 6–480 months (51.8 ± 105.6), median value: 11.0 |
Table 4.2
Causes of unilateral vocal fold paralysis
Postoperative | Thyroid gland surgery | 13 |
Aortic aneurysm | 9 | |
Mediastinum tumor | 4 | |
Esophageal cancer | 2 | |
Lung cancer | 1 | |
Idiopathic | 3 | |
Othera | 5 | |
Total | 37 |
Details of the method used for CT scanning and production of CT endoscopic and coronal MPR images are described in Sect. 4.3.1.1. Two experienced laryngologists (E.Y. and T.S.), who were blinded to patient data, evaluated all of the CT endoscopic and coronal MPR images displayed on a monitor. Differences in fold thicknesses, and the vertical and horizontal positions assumed during phonation and inhalation, were evaluated. Differences in vertical levels were assessed with the aid of coronal MPR images taken just anterior to the vocal process. It was specifically noted whether the affected fold was directed medially or superomedially during phonation. Furthermore, changes in glottal configuration suggestive of paradoxical movements were carefully observed; these included an increase in thickness, adduction of the affected fold during inhalation, and abduction during phonation.
Maximum phonation time (MPT) and mean airflow rate (MFR) during sustained phonation were measured using a phonation analyzer (SK-99; Nagashima, Tokyo, Japan) within 2 weeks before the day on which 3D CT scanning was performed. Each patient was asked to sustain the vowel “a” at a comfortable pitch and loudness, for as long as possible. Of two consecutive measurements taken, the larger value was included in analysis. The data were compared to the glottal configuration types that were identified via 3D CT image analysis. The unpaired t-test was used to explore the significance of differences in MPT and MFR among patients with various types of glottal configurations. Fisher’s exact test was employed to examine the significance of differences in the vertical positions of affected vocal folds during phonation (StatView; SAS Institute, Cary, NC). A P value <0.05 was deemed to indicate statistical significance.
4.3.2.2 Evaluation of Glottal Configuration Based on 3D CT Images
The vocal folds on the affected sides of 32 (86.5 %) of the 37 subjects were thinner than those on the healthy sides during phonation. In 18 subjects (48.6 %), the affected vocal fold was located at a higher position during phonation (Table 4.3). Figures 4.6, 4.7, and 4.9 show the three different types of glottal configuration (described in Sect. 4.3.1.3).
Table 4.3
Numbers of subjects with regard to thickness and vertical position of the vocal fold on the affected side during phonation compared to those on the healthy side in the three types of glottal configuration
Glottal configuration type | Thickness | Vertical position | |||
---|---|---|---|---|---|
Thinner | Equal | Thicker | Higher | Equal | |
A (10) | 5a | 3 | 2 | 1 | 9b |
B (12) | 12 | 0 | 0 | 7 | 5 |
C (15) | 15 | 0 | 0 | 10 | 5 |
Total | 32 | 3 | 2 | 18 | 19 |
Differentiation of abduction during phonation from adduction during inhalation was not feasible because only static 3D CT images were available for evaluation. An increase in subglottal pressure during phonation may push up the affected fold if that fold has reduced muscle tonus. Therefore, the numbers of subjects who exhibited abduction during phonation or adduction during inhalation are listed in Table 4.4. Because the vocal folds on the affected sides of three subjects exhibited both types of paradoxical movement, an increase in thickness during inhalation and abduction during phonation or adduction during inhalation, a total of 19 of 37 subjects exhibited either one or two paradoxical movements. Figure 4.9 shows a representative case. The affected fold was abducted during phonation and was barely recognizable in the coronal image. The affected fold was adducted (Fig. 4.9, black arrows) and became thick during inhalation (Fig. 4.9, thin white arrow). A wide glottal gap developed between the vocal processes during phonation. Figure 4.10 shows videostroboscopic images taken during inhalation (left) and phonation (right) of a 66-year-old male with left vocal fold paralysis (the same subject discussed in Fig. 4.9) exhibiting left-sided abduction on phonation.
Table 4.4
Numbers of subjects showing paradoxical movement of the vocal fold on the affected side in the three types of glottal configuration
Glottal configuration type | Thicker during inhalation | Abduction during phonation or adduction during inhalation |
---|---|---|
A (10) | 0 | 0 |
B (12) | 0 | 4 |
C (15) | 6a | 12a |
Total | 6 | 16 |
Because achievement of glottal closure and a symmetrical configuration of the vocal folds are important in terms of voice production, the thickness of the affected vocal fold during phonation was considered to be the critical issue when deriving the new classification. In the type A configuration, the thickness of the affected fold during phonation is equal to, or slightly less than, that of the healthy vocal fold. In this type of configuration, the affected fold is directed medially, not superomedially, during phonation (Fig. 4.6, thin arrow). Only a small glottal gap was evident between the vocal processes during phonation. Subjects in whom the affected fold was thinner than the other during phonation were further classified into types B and C. In the type B configuration, the affected fold remained thin during both phonation and inhalation (Fig. 4.7) and was directed superomedially on phonation (Fig. 4.7, thin arrow). In the type C configuration, the presence of one or two paradoxical movements of the affected fold caused the fold to be directed medially, not superomedially, during inhalation (Fig. 4.9, thin white arrow), and the fold was barely recognizable in the coronal image (Fig. 4.9) during phonation. A prominent glottal gap was evident between the vocal processes during phonation in patients with type B or C glottal configuration (Figs. 4.7 and 4.9). Figure 4.11 shows a schematic representation of the laryngeal lumen of a normally innervated larynx and those of the three different types of unilaterally paralyzed larynges (types A, B, C).

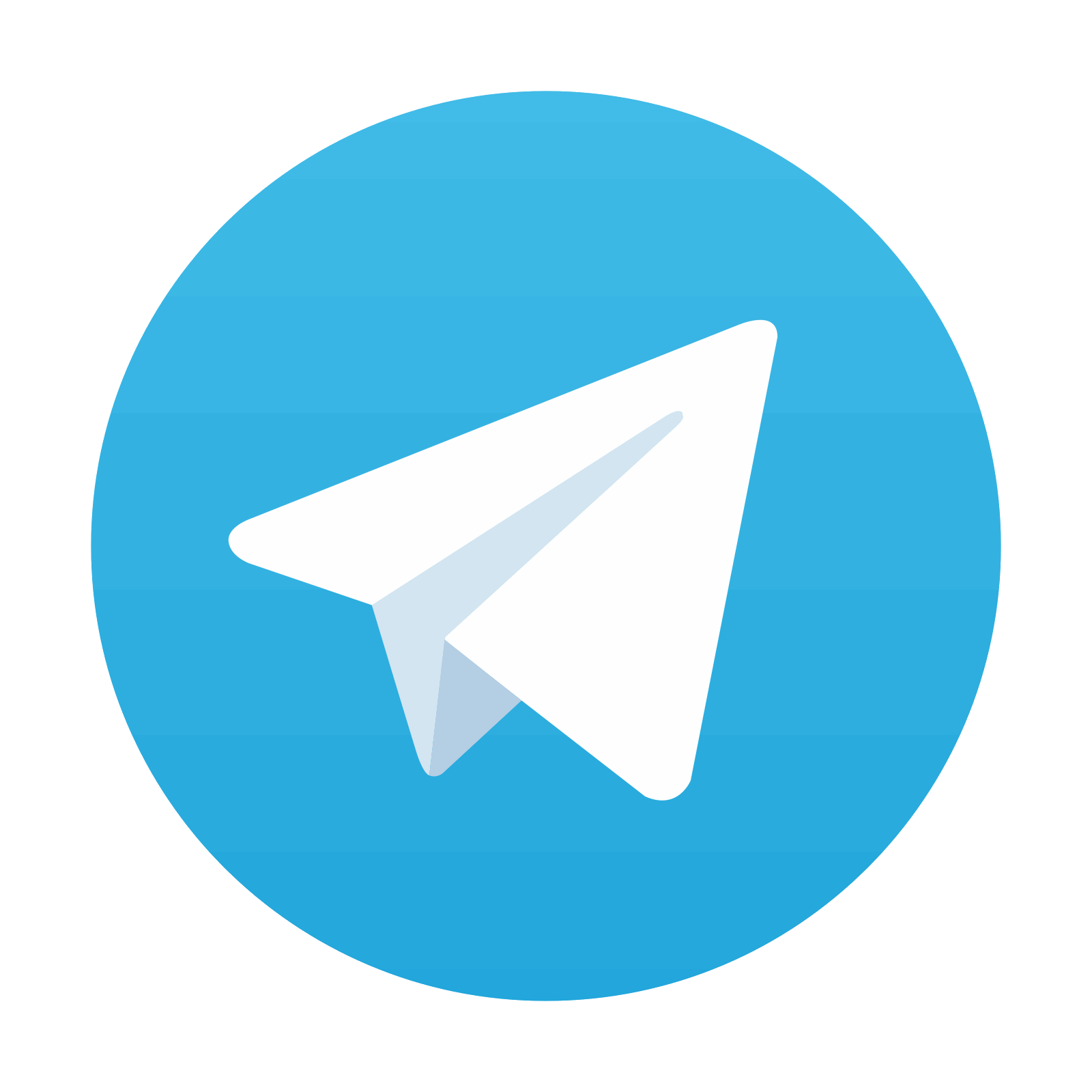

Fig. 4.11
Schematic representation of the laryngeal lumens of the normally innervated larynx and three different types of unilaterally paralyzed larynges (types A, B, and C). Upper row oral views, Lower row coronal images, Left inhalatory phase, Right phonatory phase, *Affected side (Citation: Ref. [24])
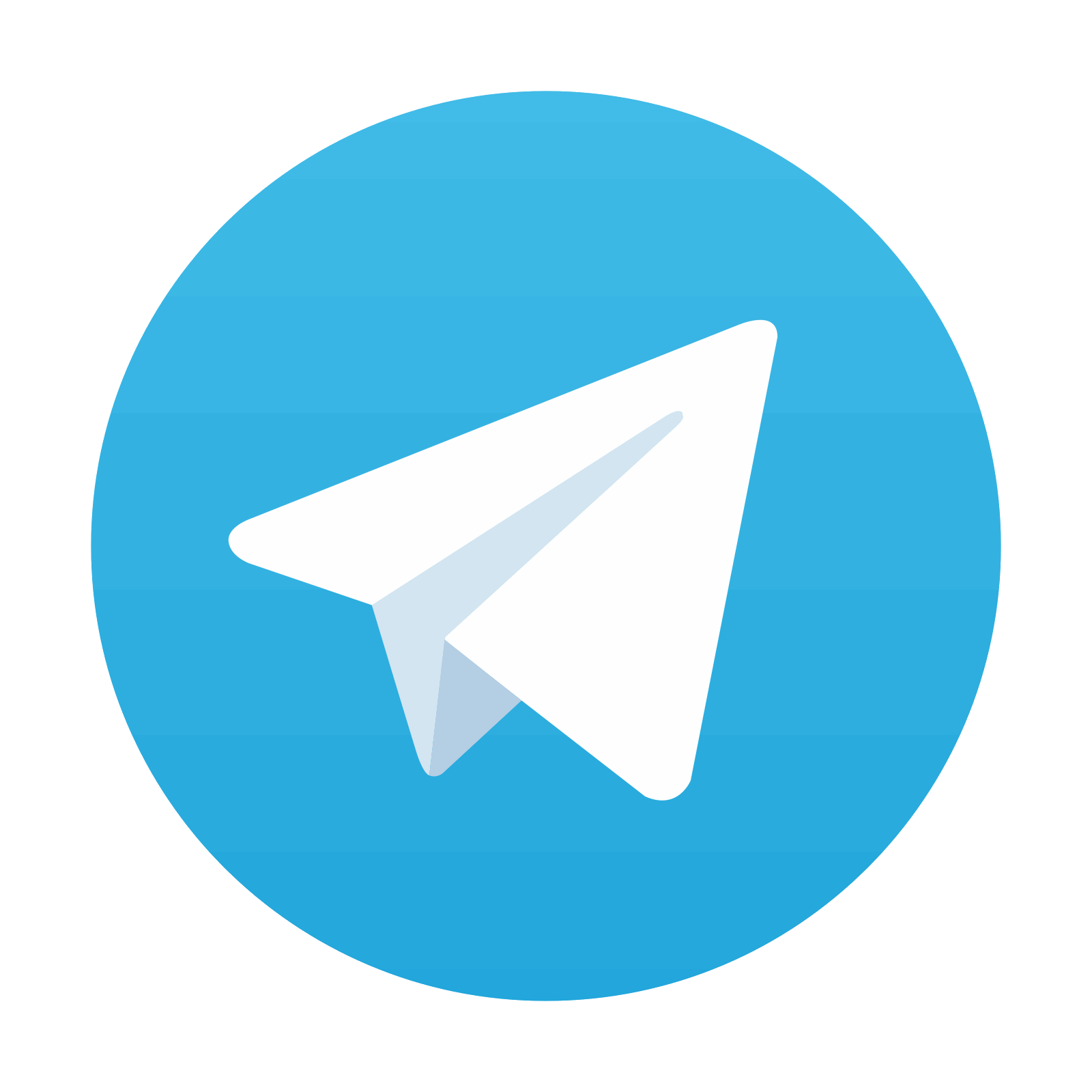
Stay updated, free articles. Join our Telegram channel

Full access? Get Clinical Tree
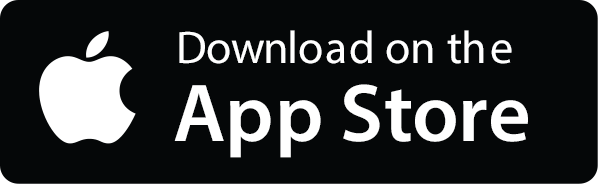
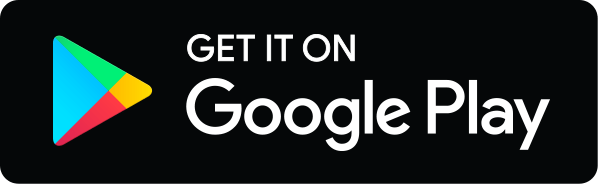
