Fig. 1
Diffuse retinal thickening pattern of diabetic macular edema (DME) with increased retinal thickness and a “spongy” appearance of the retina. There is a reduction in the reflectivity of the outer retinal layers due to the overlying cystic spaces and intraretinal fluid (top). Fluorescein angiography (bottom) demonstrates generalized leakage prominent on late frames without a discretely identifiable source

Fig. 2
Cystoid macular edema (CME) pattern of DME: Retinal thickening and hyporeflective cystic spaces in the inner retina separated by hyporeflective septae with subfoveal subretinal fluid (right). Fluorescein angiogram (left) shows paravoeal petaloid leakage corresponding to the CME and leakage temporally due to microaneurysms

Fig. 3
DME with posterior hyaloid traction (PHT) without tractional retinal detachment. Top figure shows diffuse DME with large intraretinal cystic spaces and intraretinal fluid and an adherent posterior hyaloid. Following anti-vascular endothelial growth factor (VEGF) therapy, there is an improvement in intraretinal and subretinal fluid but a persistent foveal intraretinal cyst and posterior hyaloidal traction on the fovea (bottom figure)

Fig. 4
DME with a tractional retinal detachment: Intraretinal cystic spaces superior and inferior to fovea with a tractional retinal detachment in the superior and inferior macula in a peaked configuration visualized on vertical OCT scan. Hyperreflective opacities in the vitreous represent a mild vitreous hemorrhage
In addition to these descriptive classifications, various intraretinal microstructural anatomical characteristics have been described in eyes with DME. These include hyperreflective foci (HRF), a morphologic sign of accumulation of IRF and lipid extravasation, suggested to be precursors of hard exudates before they become clinically visible [11, 16, 17]. Outer retinal HRF have been associated with disrupted ELM or ellipsoid layer and decreased VA, suggesting photoreceptor degeneration in DME. Pemp et al. [18] showed that DME reduction during anti-VEGF therapy was accompanied by dynamic rearrangement of these intraretinal exudates. Type 1 diabetes patients have fewer HRF than with type 2 diabetes [19]. Gelman et al. [20] reported hyperreflective dots in a contiguous ring around the inner wall of cystoid spaces in the OPL, a pattern that they referred to as the “pearl necklace sign.” This configuration was found adjacent to hard exudates in the OPL, and the hyperreflective material was speculated to be composed of lipoproteins or lipid-laden macrophages.
Microaneurysms (MAs) in DME have also been evaluated on OCT. The OCT parameters of leaking microaneurysms include outer and inner diameter of the microaneurysm and wall thickness [21, 22]. Hyperreflective spots on OCT in microaneurysm lumens have been suggested to be cellular components such as erythrocytes, leukocytes, and lipid deposits [22]. Lee et al. characterized microaneurysm closure following focal laser photocoagulation in DME using simultaneous FA and OCT [23]. Microaneurysm closure following focal laser photocoagulation was characterized either by hyperreflective spots or complete disappearance without any hyporeflectivity. Smaller microaneurysms with a heterogeneous lumen were more likely to close [23].
It is of critical importance to identify anatomical biomarkers of DME that predict visual outcome and guide the choice of candidate drugs for interventional trials. However, a reliable anatomical biomarker of VA in patients with DME has yet to be firmly established. OCT retinal thickness measurements, although an important clinical and anatomic evaluation tool, are not an ideal surrogate for VA as a primary outcome in DME studies. Although OCT-derived central retinal thickness is commonly utilized in DME evaluation and management, central foveal thickness (CFT) explains no more than 27 % of the variation in VA [24]. There have been attempts to identify various OCT-based biomarkers that better correlate anatomic microstructure with function and predict visual recovery in eyes with DME. These microstructures include the external limiting membrane (ELM), integrity of the ellipsoid layer (formerly described as the inner segment/outer segment photoreceptor junction) [6, 8, 25], thickness of the photoreceptor outer segments, status of the cone outer segment tips (COST) [7], presence of hyperreflective foci [11, 17, 26], and subretinal fluid (SRF) [27].
Horri et al. [28] showed that after triamcinolone acetonide treatment for DME, reduced reflectivity in foveal cystoid spaces was associated with a rebound in macular thickening and visual deterioration. Cystic macular changes have been associated with photoreceptor layer damage to a greater extent than diffuse edema and serous retinal detachment. ELM breakdown has been shown to lead to subfoveal SRF [16]. Foveal photoreceptor layer status has been closely related to visual acuity in patients with DME [9, 29]. However, the influence of the foveal avascular zone size on the photoreceptor layer integrity is yet to be clearly defined. Some of the changes evaluated on OCT persist even following DME treatment. Despite treatment and resolution of DME and restoration of macular thickness, the ganglion cell inner plexiform layer thickness in eyes with resolved DME is thinner than that in eyes without DME. This difference correlates with decreased visual acuity, suggesting that inner retinal alterations in DME may lead to visual deficiency that persists after treatment [30]. Lee et al. demonstrated that ischemia in eyes with DME caused photoreceptor outer segment shortening and ellipsoid layer disruption, resulting in outer retinal layer atrophic changes and subsequent visual loss [31]. Soliman et al. [32] found that cystoid spaces, especially in the INL, were associated with worse VA outcome after macular grid laser photocoagulation for DME. Areas beneath the OPL cystoid spaces have been shown to have longer spans of disrupted ellipsoid layer and ELM [29]. There is general consensus that a correlation exists between retinal thickness and visual acuity (VA) in patients with DME [14]. The OCT pattern that was found to be associated with worse VA was “CME”; eyes with CME had a 0.40 reduction in logMAR acuity compared with eyes that had DME without this pattern [14].
Retinal inner layer disorganization within the central 1 millimeter (mm) foveal area predict worse VA in eyes with center-involved DME [33, 34]. This anatomic feature had a higher correlation with VA than central retinal thickness, large intraretinal cysts, or current glycemic status. Disorganization of the retinal inner layers could identify eyes with a high likelihood of subsequent VA improvement or decline; disorganization of the retinal inner layers affecting 50 % or more of the central 1-mm-wide zone centered on the fovea had worse VA [33]. It has been proposed that this anatomic change represents disorganization or destruction of cells within the inner retinal layers, including bipolar, amacrine, or horizontal cells, and possibly indicates a disruption of pathways that transmit visual information from the photoreceptors to the ganglion cells. Histologic assessment of these changes would help confirm this hypothesis. If true, early-stage retinal inner layer disorganization could be used as a prognostic visual acuity marker in untreated eyes with DME.
Over recent years, in addition to improved resolution, the application of image processing to OCT image interpretation has mostly focused on the development of automated retinal layer segmentation methods [35, 36]. There have been several challenges in this effort. OCT images are often corrupted by speckle noise and need to undergo noise reduction to reduce its effect on the classification results. Speckle occurs in OCT due to the random interference of waves reflected from subresolution variances within the object. Maintaining edge-like features in the image after speckle denoising is particularly important for segmentation. It is easier to segment the retinal layers in early stages before the appearance of severe pathology [35, 37].
CME comprises a contiguous fluid-filled space containing columns of tissue; these spaces may falsely appear as separated cysts when viewed by OCT. It has been therefore suggested that retinal volume may be a better predictor of VA than central macular thickness in CME [38]. Automated segmentation of the cystoid fluid volume in CME has been described to identify regions of cystoid fluid within a three-dimensional retinal stack of images. However the correlation of this total cystoid volume with VA and its ability to distinguish intraretinal cysts from other features such as SRF or an epiretinal membrane (ERM) has yet to be established. Automated layer segmentation software allows detection of relatively few anatomical boundaries, which may limit its application in “real-world” clinical OCT images which are often not of the same quality as experimental images attained through study imaging sessions.
Imaging the Choroid in DME
There has been recent interest in the role of choroidal imaging in DME. The choroidal vasculature, especially the choriocapillaris layer, is critical to maintain the neurosensory retina as it nourishes the outer retina. Definitive changes in the choroid have been confirmed on histopathology [39]. New imaging techniques including long-wavelength OCT, polarization-sensitive OCT, and standard spectral-domain (SD) OCT with an enhanced depth-imaging mode allow assessment of choroidal thickness.
The choroid of patients with diabetic retinopathy and DME is thinner than that of age-matched healthy people as well as fellow eyes without DME [40, 41]. Subfoveal medium choroidal vessel layer and choriocapillaris layer thicknesses have also shown to be reduced in DME [42]. In contrast, Kim et al. showed that the subfoveal choroid was thicker in eyes with DME than in those without and was thickest in eyes with SRD-type DME [43]. Central choroidal thickness decreases 6 months after anti-vascular endothelial growth factor (VEGF) therapy for DME [44]. Eyes with thicker baseline subfoveal choroidal thickness may have better short-term anatomic and functional responses [45].
Fluorescein Angiography in DME
While OCT provides valuable morphologic information and is useful to monitor DME and its response to treatment [46], FA offers critical biological information such as location, intensity, and leakage source. Furthermore leakage area as measured by FA continues to be a relevant secondary endpoint in major studies of DME treatment [47].
Although FA provides additional information about DME that is complementary to OCT, change in FA leakage over time is considered by many to be a more valuable metric than the absolute leakage at a single timepoint. This is partly because quantification of features on FA is typically not as reproducible compared to other imaging modalities such as OCT. Identification of DME subtypes by FA has potential to guide therapy and monitor disease activity. Various subtypes of DME have been proposed based on differences in the pattern of fluorescein leakage [48]. Focal leakage manifests as discrete foci of leakage on early FA frames and corresponds to MAs. The diffuse subtype is characterized by generalized leakage prominent on late FA frames without a discretely identifiable source (Fig. 1). The angiographic appearance in eyes with DME can include either of these two leakage patterns, or a mixture of both [49].
Correlation between the FA macular leakage pattern and the edema morphology on OCT has been shown [21, 32, 50–52]. Variability of OCT reflectivity levels in the foveal cystoid spaces that corresponds to fluorescein pooling has been shown in DME. However the clinical relevance of this finding remains to be established [53].
While reproducible quantitative and qualitative analysis of FA is possible by experienced graders in the setting of a formal image reading center, its use for subtyping in the clinical setting is hindered by the subjective nature of FA interpretation. There has been long-standing interest in objective methods to quantify FA leakage. Segmentation of leakage on fluorescein angiograms obtained in the clinic is challenging, partly due to difficulties with FA sequence registration. There have been attempts to automate MA detection [54, 55], extraction of vessels [56], foveal avascular zone (FAZ) detection [57], and even automated leakage detection or quantification [58–61]. Rabbani et al. recently described a fully automated image segmentation algorithm without manual inputs to reproducibly and accurately quantify DME leakage area [62].
Using OCT and FA, Bolz et al. [21, 63] proposed the SAVE protocol for DME categorization. “S” stands for subretinal fluid, “A” for area, “V” for vitreoretinal interface abnormalities, and “E” for etiology. Based on etiology, DME leakage was categorized as focal or multifocal (FA with definable leakage source), non-focal capillary leakage (FA without definable leakage source), macular or peripheral ischemia (ischemia anywhere on FA associated with focal or non-focal edema on OCT), and atrophic edema (cystoid swelling on OCT). Newer technologies like en face OCT, OCT angiography, and retromode scanning laser ophthalmoscope (SLO) are also being investigated in DME.
Pseudophakic Cystoid Macular Edema
CME associated with cataract extraction was initially described by A. Ray and Irvine, Jr., in patients with unexplained visual loss following intracapsular cataract extraction. Subsequently, this phenomenon was identified by Gass and Norton as macular edema with a classic perifoveal petaloid FA staining pattern and late nerve leakage [64, 65].
More recently, OCT characteristics of pseudophakic CME have been described. These features include macular thickening and cystic spaces in the OPL, occasionally with subfoveal fluid (Fig. 5). An OCT-based automated statistical classification approach to differentiate DME from pseudophakic CME has been evaluated. Grading parameters included assessment of CME pattern, cyst distribution in ETDRS grid, morphologic features, and quantitative parameters such as individual layer thickness. Munk et al. [66] showed that higher central retinal thickness/volume ratio, the absence of ERM, and solely inner nuclear layer (INL) cysts indicated pseudophakic CME; a higher ONL/INL ratio, the absence of SRF, the presence of hard exudates, microaneurysms, and ganglion cell layer and/or retinal nerve fiber layer cysts favored DME. The optical density of subretinal fluid in DME and pseudophakic CME was similar [67].


Fig. 5
Pseudophakic CME with cystic spaces and subfoveal subretinal fluid (bottom). There is parafoveal petaloid leakage on the fluorescein angiogram and mild leakage at the optic nerve (top)
Oh and associates reported the presence of vitreous hyperreflective dots following phacoemulsification. The number of hyperreflective dots detected 1 week following surgery predicted the development of CME at 1 month [68]. These vitreous hyperreflective dots were thought to correspond to lens fragments, denatured proteins, or clumps of intraocular cells. They were >20 μm in size which was larger than vitreous cavity cells seen as ~15 μm hyperreflective dots on OCT in uveitic eyes [69]. The authors concluded that despite the unclear nature of these dots, their association with pseudophakic CME suggested a relation to postoperative inflammation and vascular permeability.
Evaluation for previously undiagnosed photoreceptor disruption is important in assessment of eyes with unexplained vision loss despite resolution of pseudophakic CME. Using a 4 μm resolution OCT [70], persistent anatomic alteration of photoreceptors, described as a blurring of cone photoreceptor outer segment tips, correlated with reduced visual acuity in eyes with resolved pseudophakic CME that did not achieve 20/20 visual acuity compared with eyes that did.
Other OCT characteristics associated with pseudophakic CME include vitreomacular traction (VMT), extrafoveal vitreoretinal traction [71, 72], ERM, or ERM following prior ERM peeling [73, 74]. Odrobina et al. [75] recently suggested that a thinner choroid in eyes with pseudophakic CME compared to fellow eyes indicate that reduced choriocapillaris blood flow may be a possible CME etiologic factor. Others have reported, however, that eyes with pseudophakic CME had greater thickening of the subfoveal choroid, which preceded CME development by 1 month [76].
CME Associated with Retinal Vascular Occlusions
CME, a major cause of visual acuity loss in patients with retinal vascular occlusion (RVO) [77], is characterized by intraretinal fluid accumulation with diffuse retinal thickening or formation of cystoid spaces, SRF accumulation, or macular traction due to ERM formation (Figs. 6 and 7). OCT assessment of retinal thickness and structural changes provides useful information to determine treatment strategy for RVO-associated CME and to predict the long-term visual prognosis. OCT anatomic parameters such as foveal thickness, serous retinal detachment, central cystoid spaces, and pigment epithelial changes correlate with decreased visual recovery after RVO [78, 79].



Fig. 6
CME in non-ischemic central retinal vein occlusion (CRVO) with diffuse retinal thickening, cystoid spaces, and accumulation of subretinal fluid (top). Fluorescein angiogram shows diffuse parafoveal leakage (bottom)

Fig. 7
CME in branch retinal vein occlusion (BRVO) with intraretinal cystoid spaces, no subretinal fluid (top), petaloid leakage on fluorescein angiogram (middle), and parafoveal hyper fundus autofluorescence (FAF) pattern on fundus autofluorescence (bottom)
Various RVO anatomic biomarkers have been evaluated with OCT. In eyes with central retinal vein occlusion (CRVO), foveal thickness >700 μm should raise suspicion for an ischemic form of CRVO [80]. In BRVO, cystoid spaces >600 μm in diameter have been associated with a longer occlusion of duration and poor visual improvement with bevacizumab therapy [81, 82]. Loss of the subfoveal ellipsoid layer and the absence of the inner retinal layers on OCT are correlated with poor visual outcomes in patients with CRVO [83] and branch retinal vein occlusion (BRVO) [79, 84, 85]. In addition, loss of the inner retinal layers correlates with macular ischemia diagnosed in early FA frames [78].
Tsujikawa and associates reported that a breakdown of the ELM barrier function caused movement of IRF into the subretinal space in RVO-associated CME [86]. They also reported highly reflective vertical lines beneath the cystoid spaces that were proposed to represent tracks through which the IRF within the cystoid spaces flowed into the subretinal space [16, 86, 87]. Hasegawa et al. also observed highly reflective vertical lines termed “track lines” beneath the cystoid spaces in the OCT images similar to those described by Tsujikawa et al., but note that the lines persisted despite resolution of CME [86, 88]. It has been suggested that the track lines probably developed when the CME resolved rapidly by the treatment. They observed these track lines at the fovea after resolution of CME associated with BRVO (Fig. 8). Track lines are thought to cause the localized damage of the photoreceptors. Hyperreflective foci are track line components, and it is thought that hyperreflective foci deposited in the outer retina causes the photoreceptor damage [11, 16, 89–91]. The specific mechanism that accounts for photoreceptor damage is unknown, but it has been proposed that macromolecules in IRF pass through small ELM disruptions and cause photoreceptor damage. Therefore, the track lines may be associated with localized rather than diffuse photoreceptor damage. Hasegawa et al. [88] suggested that the track lines are associated strongly with an initially disrupted ELM and thus might not be detected in eyes with spontaneous CME resolution. Track lines may thus be a useful marker of photoreceptor damage in eyes with resolved macular edema associated with BRVO.


Fig. 8
CME in BRVO with subretinal fluid (top) and a hyperreflective track line (below, white arrow) at the fovea after resolution of CME
Another biomarker that has been evaluated is an inward curvature of the foveal ellipsoid zone, seen in normal eyes, and termed the “foveal bulge” [92]. The foveal bulge is a good marker of visual functional in eyes with resolved BRVO-associated CME. The presence of the foveal bulge indicates better BCVA after resolution of the macular edema associated with BRVO [92]. In eyes with an intact foveal ellipsoid layer after resolution of BRVO-associated CME, the retinal thickness at the foveal center was thinner, and the photoreceptor OS length was shorter in the group without a foveal bulge than in the group with it [92]. The study suggested that CME damages foveal photoreceptor outer segments resulting in the absence of a foveal bulge.
Ellipsoid layer disruption at the central fovea has been shown in eyes with poor visual acuity despite complete resolution of CME (Fig. 9) [79, 83, 85]. It has also been shown that the integrity of the ellipsoid layer correlates with VA in eyes with resolved RVO-associated CME [92–94]. An association between the initial foveal thickness and final VA is somewhat controversial. While some have reported a correlation between the initial foveal thickness and final VA in eyes with RVO and persistent CME after treatment [82, 85], others have not observed this association [82, 94].


Fig. 9
Lack of visual acuity improvement in an eye (stable at 20/60) with CME associated with non-ischemic CRVO (top) despite near-complete resolution of CME following treatment with anti-vascular growth factor injections attributed to ellipsoid layer disruption at the central fovea (bottom)
Various anatomical characteristics have been evaluated as potential prognostic indicators in RVO-associated CME. In CRVO-associated CME, the presence of SRF or the diameter of cystoid spaces has not been shown to be predictive for treatment outcome [82]. Ellipsoid layer integrity and ELM status at baseline correlate with better visual outcomes after anti-VEGF treatment for RVO- associated CME [94, 95]. Severe photoreceptor damage during the acute or chronic phase of RVO might lead to a substantial photoreceptor outer segment defect, resulting ellipsoid zone loss [79, 83]. Kang et al. suggested that hyperreflective foci detected on the baseline OCT were predictive of visual outcomes following anti-VEGF treatment [94]. Fine hyperreflective foci found on OCT, however, could not be found on fundus photographs taken simultaneously. In contrast, confluent hyperreflective foci on OCT were detected as hard exudates in the corresponding fundus photograph, and a previous study suggested that these fine foci, characterized by the same hyperreflectivity as confluent dots, might be small intraretinal protein and/or lipid deposits which are precursors of hard exudates (Fig. 10) [16].


Fig. 10
Hyperreflective foci in an eye with BRVO and CME (top) with increased subretinal lipid exudates 8 weeks later (bottom). It has been suggested that these foci could be small intraretinal protein and/or lipid deposits and precursors of hard exudates
In eyes with BRVO, ELM and ellipsoid layer were significantly more disrupted in eyes that had hyperreflective foci as part of the track lines in the outer retinal layers [88]. These findings suggested that photoreceptor status, rather than foveal thickness, was more likely correlated with the final BCVA, after CME treatment in BRVO. Asymmetric CME distribution in the vertical scan is often pathognomonic for BRVO. Because of the higher prevalence of superotemporal vein occlusions [96], vertical line scans show a superior macular edema pattern in BRVO with a higher prevalence of cysts in the inner superior ETDRS subfield (Fig. 11).


Fig. 11
CME associated with a superotemporal BRVO with intraretinal hemorrhages: fundus autofluorescence (FAF) demonstrates hyperautofluorescence in the fovea corresponding to the CME and hypoautofluorescence superiorly corresponding to the hemorrhages (top), vertical OCT scan shows a superior macular edema pattern (bottom)
CME Associated with Vitreoretinal Interface Abnormalities
An ERM results from proliferative change at the vitreoretinal interface. Tangential traction from ERM may cause macular thickening with or without fluorescein leakage. ERMs can also distort the underlying retina and create cystoid spaces.
On OCT the posterior hyaloid, a minimally reflective structure, can often be differentiated from an ERM, which is highly reflective [99]. Wilkins et al. described two patterns of ERM adherence [100]: a broadly attached ERM, which was most common (Fig. 12), and less frequently, ERM with focal attachments. OCT has also been helpful to confirm the relationship between a posterior vitreous detachment and ERM and is valuable to follow ERM natural history. Partial or complete PVD has been found in 80–95 % of eyes with idiopathic ERM [101–103].


Fig. 12
CME with intraretinal cystoid spaces and subretinal fluid associated with a broad epiretinal membrane. The epiretinal membrane has caused distortion of the inner retina
VMT is an anomalous, posterior vitreous attachment that causes macular anteroposterior traction in areas of residual vitreous adhesion. The adherent vitreous cortex results in a broad, often dumbbell-shaped region, encompassing the macula and optic nerve [104]. This traction is associated with cystoid macular thickening (Fig. 13).


Fig. 13
Vitreomacular traction resulting in increased foveal thickness, intraretinal cysts and disruption of the ellipsoid layer (top), and restoration of normal foveal contour along with resolution of cystic spaces following spontaneous release of traction (bottom)
The phase III trials of Microplasmin Intravitreal Injection for Non-surgical Treatment of Focal Vitreomacular Adhesion (MIVI-TRUST) evaluated enzymatic vitreolysis with ocriplasmin. In these trials, OCT and clinical examination was used to assess retinal morphology; these investigations confirmed the superiority of OCT to clinical examination [105]. The study described two subclasses of VMT: focal (≤1500 μ) and broad (>1500 μ) adhesion [106–108]. Koizumi et al. [107] showed that eyes with focal VMT had a foveal cavitation, whereas eyes with broad VMT had more widespread CME. In VMT, the posterior hyaloid usually appears hyperreflective and thickened on OCT. Yamada and Kishi [109] described two types of partial PVD patterns – incomplete vitreous detachment nasally and temporally causing a V-shaped pattern with attachment only at the fovea and the second type showing persistent nasal attachment and detachment temporal to the fovea. The first type of PVD had postoperatively better visual outcomes compared with the second type.
CME Associated with Uveitis
Hassenstein and colleagues were the first to describe the use of OCT in uveitis. This group found that OCT was useful in detection of early CME and monitoring of treatment efficacy, especially when vitreous cells were present [110, 111]. Specific OCT patterns have been identified in CME associated with uveitis, similar to those reported in DME: diffuse macular edema (characterized by increased retinal thickness, disturbance of the layered retinal structure or sponge-like low reflective areas) (Fig. 14), cystoid macular edema (characterized by clearly defined intraretinal cystoid spaces) (Fig. 15), and serous retinal detachment (characterized by a clean separation of the neurosensory retina from the RPE/choriocapillaris band) (Fig. 16) [13, 111, 112]. Iannetti et al. imaged 43 eyes and found that 58 % had cystoid macular edema, 42 % had diffuse macular edema, and 28 % of all cases had serous retinal detachment. The relative frequency of the three different patterns in uveitis varies depending on the patient selection criteria [111, 113, 114].




Fig. 14
Diffuse pattern of uveitic CME associated with sarcoid. The retina has inner and outer plexiform layer cystoid spaces, hyperreflective foci, and subretinal fluid

Fig. 15
CME associated with autoimmune retinopathy with parafoveal hyperautofluorescence on FAF (top). There is retinal thickening in the fovea with intraretinal cystoid spaces and diffuse retinal thinning with outer retinal loss in the surrounding temporal area without edema (bottom) and a corresponding hypo autofluorescent pattern on FAF

Fig. 16
Increased fundus hyperautofluorescence on FAF upon development of intraretinal cystoid spaces and subretinal fluid (bottom) in CME associated with birdshot chorioretinopathy
OCT also detects ERM, often with concurrent vitreoretinal traction in a higher percentage of uveitic CME eyes compared to ophthalmoscopy [112]. A tractional mechanism has also been hypothesized as a cause of or contributor to uveitic CME. The presence of an ERM is independent from the site of inflammation, type of edema, and macular thickness [112].
A ringlike non-cystic thickening that surrounds the foveal center has been seen in eyes with iridocyclitis. It has been theorized that the same pathophysiological mechanism that underlies this non-cystic ringlike thickening accounts for the CME in eyes with anterior uveitis. This thickening, lasting <6 months after an acute episode, has been reported in 45 % of acute anterior uveitis cases [115], and normal macular volumes are restored by 6 months after the uveitis flare-up [116].
A negative correlation between central subfield thickness and VA has been described in uveitic CME [111, 112, 114, 117, 118]. Microperimetry has also been shown to correlate with central subfield thickness and VA in eyes with uveitic CME [119]. Cystoid changes in OPL and INL and the presence of ERM were associated with poor VA. OCT has also been used to monitor the therapeutic response in uveitic CME [120–122]. Lehpamer et al. [123] demonstrated that SRF in uveitic CME was associated with increased central subfield thickness and worse VA at presentation. However, eyes with SRF responded well to treatment at 3 and 6 months, achieving greater rates of improvement than eyes without SRF and recovered to a similar level of final VA. An initial increase in SRF may occur during the process of macular edema absorption [124].
Markomichelakis et al. evaluated the prognostic significance of OCT patterns of macular edema. These coworkers found that eyes with a diffuse pattern of macular edema often have good visual acuity and do not need to be aggressively treated. This undertreatment, however, makes them prone to an increase in macular thickness and decreased VA during an inflammatory relapse [124]. They also found the presence of an ERM to be a poor prognostic factor in uveitic CME and associated with medical treatment failure [124]. IRF in uveitic CME may be dynamic; retinal thickness changes can be seen seconds after a change in patient position [125]. CME also has a diurnal variation with the main decrease in retinal thickness occurring before noon [125].
CME seen by OCT in patients with juvenile idiopathic arthritis (JIA)-associated uveitis has been reported in 84 % of eyes [126], a figure higher than that observed in previous ophthalmoscopy-based reports [127, 128]. JIA-associated uveitic OCT changes include perifoveal thickening, CME, foveal detachment, and atrophic changes. Duration of JIA-uveitis correlated with the development of CME [128].
Central subfield thickness is an important endpoint for various clinical trials and is an important parameter in the clinical management of uveitic CME. The Multicenter Uveitis Steroid Treatment (MUST) trial [129] evaluated a clinically meaningful OCT-determined thickness threshold in eyes with uveitic CME. A 20 % change in retinal thickness in eyes with uveitic CME (defined as retinal thickness at the central subfield >260 μm) was optimal to predict more than a 10-letter change in VA, with 77 % sensitivity and 75 % specificity. This threshold is important for uveitis trials wherein uveitic CME improvement is monitored through changes in central subfield thickness and associated with clinically meaningful VA changes. The MUST trial also showed that OCT and FA only agreed moderately to identify uveitic CME in eyes with intermediate, posterior, and panuveitis, probably because macular thickening on OCT (time domain) and macular leakage are related but nonidentical pathologic characteristics [130]. Fluorescein leakage indicates pathological leakage from blood vessels, which is often but not always associated with macular thickening. Reasons for lack of thickening when there is fluorescein vascular leakage include the following non-mutually exclusive mechanisms: severely damaged atrophic maculae, with superimposed ongoing inflammatory leakage, a macula with very recent leakage that has preceded retinal thickening, macular distortion secondary to ERM without associated thickening, or a steady state whereby macular leakage is balanced by physiologic fluid egress from the macular retina [130]. The MUST trial also showed that the presence of macular cysts on OCT was associated with increased retinal thickness by OCT, hyperfluorescent cystoid spaces on FA, and macular leakage on FA [130]. Small cysts and ERMs involving the center were common in intermediate and posterior/panuveitis and required systemic corticosteroid therapy [131]. The MUST reading center methodology defined ERM as a hyperreflective layer with a bridging effect over the inner retinal layers, thus potentially excluding broadly adherent ERMs if their reflectivity merged with the nerve fiber layer. Corrugation of inner retinal layers was also considered insufficient to identify an ERM [131]. These results suggest that in uveitic CME, FA and OCT offer related yet unique clinically important information on macular pathologic features.
Similar to DME, the relationship between VA and CME in uveitis is imperfect. Payne et al. [132] determined the utility of logarithmic transformation of OCT retinal thickness data to assess clinically meaningful changes in uveitic CME. Log scale OCT thickness correlated with logMAR visual acuity suggesting its use as an objective measure in uveitic CME [132]. These researchers also showed that in uveitic CME, the volume between the plexiform layers was the best indicator of visual function at baseline [38]. Brar et al. reviewed FA and OCT images of 87 patients with CME due to diabetes, ERM, uveitis, pseudophakia and vein occlusion. They concluded that while cystoid leakage on FA was always associated with cystic OCT changes, diffuse non-cystoid leakage on FA was associated with thickening and distortion of the retinal layers without cyst formation [133]. Diffuse uveitic CME has been associated with a poor visual prognosis and a poor prognosis for vision recovery [124]. SRF, however, is associated with a high probability of vision recovery in uveitic CME [134]. This is in contrast to SRF in DME that is associated with a poor prognosis for visual recovery [32].
FA is useful in differentiating active from inactive uveitis and also to confirm a CME diagnosis, choroidal neovascularization, and subtle retinal vasculitis, to monitor response to therapy, and to identify areas of capillary non-perfusion and retinal neovascularization. The small molecules of free, unbound fluorescein dye leak out even from minimally inflamed retinal vessels [135]. The characteristic appearance in eyes with uveitic CME is a “petaloid” pattern of parafoveal hyperfluorescence [135]. CME has been angiographically graded as [136] Grade 0, no sign of fluorescein leakage; Grade I, slight fluorescein leakage into cystic spaces but not enough to enclose the entire fovea centralis; Grade II, complete circular accumulation of the fluorescein in the cystic space but its diameter is smaller than 2 mm; and Grade III, the circular accumulation of fluorescein is larger than 2.0 mm in diameter.
There have been attempts to describe OCT anatomical characteristics that can identify CME of different etiologies. Microfoci, thought to be caused by lipid-rich and lipoprotein-rich deposits or lipid-laden macrophages [16], were found to be one such differentiating characteristic on OCT [137]. These foci are characteristic for DME and RVO, although they differ in location and presentation according to the underlying disease [16, 138]. Munk et al. reported that microfoci were found in 100 % of CME eyes with CRVO, in 98 % of the eyes with DME, and in 65 % of eyes with BRVO, but in no eye with pseudophakic CME or uveitic CME [137]. IRF accumulation occurs in CME irrespective of the disease entities and differences in morphologic and spatial presentation, although previous histologic reports indicate that IRF may vary according to its underlying pathology [26, 139, 140].
Posterior uveitis is accompanied by choroidal thickening especially in an acute phase [141, 142]. With this technique, macular choroidal changes in eyes with anterior and intermediate uveitis are less marked compared to posterior uveitis and panuveitis [143].
CME is related to the use of drugs like prostaglandin analogues, epinephrine and epinephrine-like drugs, nicotinic acid, pioglitazone, rosiglitazone, docetaxel, and paclitaxel presumably by inducing an inflammatory reaction that causes breakdown of the blood retinal barrier and can also be monitored using OCT [144–149].
Role of Fundus Autofluorescence Imaging in CME
Fundus autofluorescence (FAF) is determined by the lipofuscin distribution in the RPE and is also influenced by macular pigments in the INL, ONL, and OPL [150, 151]. RPE autofluorescence depends on outer segment renewal and can be affected by the RPE’s ability to clear lipofuscin. Lipofuscin accumulation leads to reduced RPE phagocytic capacity which in turn can lead to RPE cell death and photoreceptor loss. Increased FAF is seen with RPE dysfunction and decreased FAF with loss of photoreceptors or the RPE [152].
CME is associated with increased FAF, thought to be due to macular neurosensory retinal tissue stretching that displaces macular pigments laterally thereby reducing the density of macular pigments, which increases the autofluorescence signal (Fig. 10) [153–155]. In eyes with CME, there have been attempts to correlate FAF with OCT parameters and VA and to predict restoration of photoreceptor integrity and subsequent visual recovery [156].
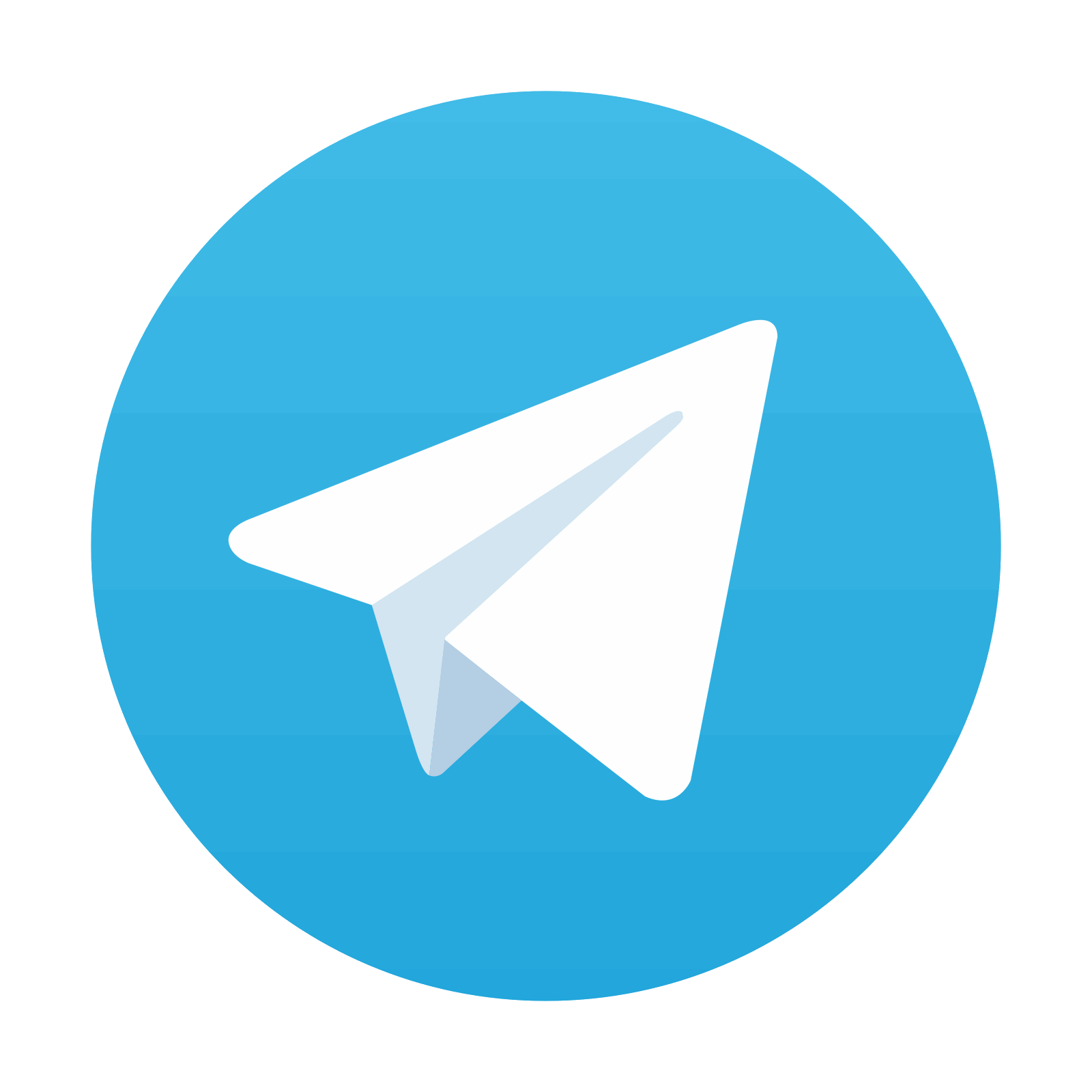
Stay updated, free articles. Join our Telegram channel

Full access? Get Clinical Tree
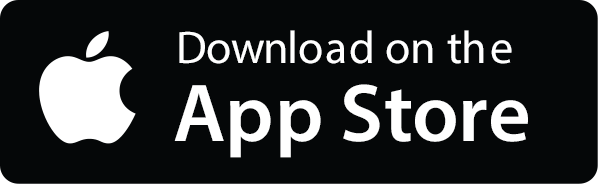
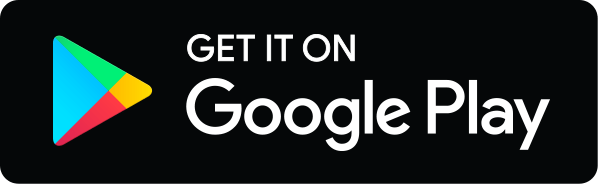