Multimodal imaging in a healthy subject. Multicolor (a) and fundus autofluorescence (b) images show the integrity of posterior pole structure, with the physiologic distribution of autofluorescence signal coming from the retinal pigment epithelium cells. Horizontal (c) and vertical (d) structural OCT scans show the normal reflectivity properties of retinal and choroidal layers. OCTA is able to accurately reconstruct retinal vascular network, namely superficial (e), deep (f) and choriocapillary (g) plexa
Fluorescein Angiography
Fluorescein Angiography (FA) has been in use in ophthalmologic practice since 1961, when two medical students from Indiana University described and demonstrated the technique [1]. FA requires the intravenous injection of fluorescein dye, which rapidly reaches eye circulation. White light passes through a blue excitation filter, and the blue light is absorbed by fluorescein molecules, which in turn emit light in the yellow-green spectrum. A barrier filter allows capturing only light emitted from the excited fluorescein, and the images are recorded. First FA images were recorded on photographic film; nowadays images are recorded digitally, thus allowing easier data analysis, storing and sharing, which can be easily stored and shared. Newer angiography devices support also movie capturing, making the interpretation of the vascular filling details, more identifiable.

Fluorescein angiography in a case of myopic choroidal neovascularization. Multicolor (a) image shows the characteristic features of a myopic fundus, including retinal thinning and peripapillary atrophy. Moreover, an altered foveal reflex is also detected. FA examination clearly shows the presence of two classic CNV, being detectable already in early phase (b), with progressive leakage phenomena in intermediate (c) and late (d) phases
Indications of fluorescein angiography, indocyanine green angiography and optical coherence tomography angiography in macular diseases
Fluorescein angiography | Indocyanine green angiography | Optical coherence tomography angiography | |
---|---|---|---|
Indications | • Neovascular age related macular degeneration • Diabetic retinopathy • Retinal vein occlusion • Retinal artery occlusion • Genetical macular dystrophy • Central serous chorioretinopathy • Uveitic macular edema | • Occult choroidal neovascularization • Choroidal neovascularization with subretinal hemorrhage • Central serous chorioretinopathy | • Neovascular age related macular degeneration • Diabetic retinopathy • Retinal vein occlusion • Retinal artery occlusion • Genetical macular dystrophy • Central serous chorioretinopathy • Uveitic macular edema |
Indocyanine Green Angiography
Indocyanine Green Angiography (ICGA) was originally described by Yannuzzi in 1992 [3]. Indocyanine green is a water-soluble dye which is almost completely bound to serum proteins (98%), thus its diffusion through choriocapillaris is limited. The retention of the dye in the choroidal circulation makes indocyanine green angiography ideal for imaging of choroidal circulation. As well as FA, ICGA is invasive and requires intravenous injection of the dye.


Multimodal imaging of a choroidal neovascularization secondary to age-related macular degeneration. Early (a) and late (b) FA phases show the presence of a CNV with the typical pinpoints alterations. ICGA is able to better detect the entire neovascular network, respectively in early (c) and late (d) phases. Structural OCT (e) confirms the presence of a subretinal mixed reflectivity lesion, together with the presence of subretinal fluid and intraretinal cysts
Optical Coherence Tomography
Optical Coherence Tomography (OCT) is a non-invasive imaging modality which allows to acquire retinal cross-section in vivo. Since its original introduction in 1991 [5], OCT has revolutionized the retinal imaging and the evaluation of the macular pathologies. Diagnosis of maculopathy, previously based on fundus biomicroscopic examination, retinography or FA, in most of cases now relies upon OCT detailed contribution. OCT can quantitatively measure retinal thickness and evaluate qualitative anatomic changes, such as intraretinal or subretinal fluid.
Comparison between different commercially available optical coherence tomography devices
Time domain | Spectral domain | Swept source | |
---|---|---|---|
Commercialization | 1996 | 2006 | 2012 |
Acquisition (A-scans per second) | 400 | 20,000–80,000 | 100,000–400,000 |
Resolution | 10–15 μm | 2–8 μm | 2–8 μm |
Characteristics | A moving reference mirror is required, limiting the acquisition rate of the technology Mostly inadequate for current clinical use | Higher sensitivity than TD-OCT High scanning speed and axial resolution and good visualization of retinal layers It has limited penetration with noticeable signal drop-off with depth | Higher sensitivity than TD-OCT, with very high scanning speeds, and minimal signal drop-off with depth It has high spatial resolution and better tissue penetration (from vitreous to choroid) |
Optical Coherence Tomography Angiography
Optical coherence tomography angiography (OCTA) is a recent imaging technique based on OCT, which allows the study of blood vessels in the eye. OCTA uses the variation in OCT signal caused by moving particles (such as red blood cells) in order to infer blood flow. To discriminate the moving particles from static tissue, OCTA devices perform repeated scans at the same location, and the changes of the OCT signal in consecutive scans are employed to visualize the microvasculature [10].
OCTA has many applications in retinal and choroidal vascular imaging, with special focus on the macular disorders.
In age related macular degeneration (AMD), OCTA allows the detection of choroidal neovascularization (CNV), and a more detailed visualization of its structure than dye-based angiography examinations.
In diabetic retinopathy OCTA can show microaneurysms, appearing as focally dilated saccular or fusiform capillaries in the superficial and deep retinal capillary plexa. It is important to remark that not all microaneurysms seen on fluorescein angiography can be detect by OCTA, and vice-versa. OCTA can also visualize the retinal areas of non-perfusion in all the ischemic retinopathies.
OCTA is useful to confirm the clinical diagnosis of retinal vein occlusion or retinal artery occlusion, as it can identify the areas of capillary nonperfusion and retinal ischaemia, and moreover it can detect the presence of collateral vessels, capillary telangiectasia and microaneurysms.
OCTA in also used in genetical macular dystrophy in order to evaluate the damages in retinal plexa and choriocapillaris, and also to detect the presence of CNV.
OCTA could be useful in improving the knowledge of uveitic macular edema, even if there are few studies so far [11].
Comparison between fluorescein angiography and OCTA techniques
Techniques | Microaneurysms | IRMA | DME | Ischemic areas | Neovascularization | Neovascularization growth | Secondary vascular branching | Peripheral vascular arcades | Anastomotic loops |
---|---|---|---|---|---|---|---|---|---|
Fluorescein angiography | Yes | Yes | Yes | Yes | Yes | No | No | No | No |
OCTA | Yes | Yes | Yes | Yes | Yes | Yes | Yes | Yes | Yes |
Fundus Autofluorescence
Fundus autofluorescence (FAF) imaging is a rapid and noninvasive technique. It is used mainly to evaluate retinal pigment epithelial function, as the predominant source of autofluorescence in the macula is lipofuscin granules.
Blue autofluorescence is the intrinsic fluorescence emitted by lipofuscin granules when stimulated by blue light. With retinal aging, lipofuscin granules accumulate in the RPE cells. Normal macula shows a reduced autofluorescence in its center due to the blockage of luteal pigments (lutein and zeaxanthin), while the rest of the macula shows a diffuse autofluorescence signal. Other structure such as the blood vessels and the optic disc appear black because they do not have autofluorescent material [12]. In contrast to blue autofluorescence, green-light autofluorescence imaging is less affected by macular pigments, and it could probably allow a more precise evaluation of small central changes [13].
Fundus autofluorescence indications
• Age related macular degeneration – Drusen – Neovascular age related macular degeneration – Geographic atrophy • Hereditary macular dystrophy – Best macular dystrophy – Stargardt macular dystrophy – Rod-cone dystrophies – Pattern dystrophies • Areas of retinal atrophy • Retinal areas of pigment accumulation • White dot syndromes • Optic disk drusen |

Fundus autofluorescence in a myopic CNV. At baseline, fundus autofluorescence shows the presence of macular autofluorescence changes, with an increase of hypoautofluorescent signal (a); structural OCT clearly detects the presence of a subretinal hyperreflective lesion, together with the presence of subretinal fluid (b). After anti-VEGF injections, fundus autofluorescence shows an increase of hypoautofluorescent signal, interpretable as increased atrophy (c), confirmed also by structural OCT (d)

Multimodal imaging in a case of Best disease. The vitelliform material appears hyperautofluorescent (a) and it masks the signal provided by melanin in NIR-AF (b). Structural OCT (c, d) clearly shows the vitelliform acculumation, with rarefaction of outer retinal layers and normally reflective inner layers

Multimodal imaging in a case of geographic atrophy. FAF image (a) clearly shows the hypoautofluorescent region, affected by the atrophic process. The hyperautofluorescent perilesional signal is typical of GA. The posterior pole atrophy is also confirmed by multicolor image (b), showing a marked depigmentation. Structural OCT (c, d) clearly shows the atrophy of the outer retinal layers, with window effect artifact interesting the choroid, the latter caused by the lack of light absorption caused by the atrophy of the retinal pigment epithelium
Near-infrared autofluorescence imaging (NIR-AF) in another autofluorescence technique, which evaluates melanin and its distribution throughout the RPE and choroid. NIR-AF can be performed to detect melanin in different macular pathologies (AMD, inherited macular dystrophies, diabetic macular edema, central serous chorioretinopathy). This technique can extend our ways of studying the foveal involvement in macular diseases, since the higher concentration of melanin is present at the level of the fovea. Even if NIR-AF is easily performed with commercially available instruments, it has some limitations, and further research is needed to better understand and to standardize NIR-AF imaging [14].
New Perspectives: Artificial Intelligence and Telemedicine

Multimodal imaging in a case of central serous chorioretinopathy. FA shows the presence of a fugal hyperfluorescent point, increasing in dimension from early (a) to intermediate and late phases (b, c). ICGA confirms the presence of the fugal point in all three phases (d–f); moreover, it shows a masking effect secondary to the presence of massive fluid. This latter is accurately detected by multicolor image (g) and structural OCT (h), accurately documenting the loss of physiologic foveal profile. After the treatment with eplerenone, both multicolor image (i) and structural OCT (j) document the restoration of macular features
Artificial intelligence has a great potential to improve medical activity and health care quality. Several studies have demonstrated that artificial intelligence software can identify retinal diseases with the same or better accuracy than human specialists, even if their role in medical decision-making is still controversial. Nowadays, the most encouraging results are obtained for age related macular degeneration and diabetic retinopathy [15].
Another important implementation in maculopathy care could be portable devices. For example, a portable and self-measuring OCT system may reduce the cost of managing chronic maculopathy by providing easily accessible and continuous retinal monitoring [16].
Advances in Intravitreal Treatments


Multimodal imaging in a case of Stargardt disease complicated by the onset of a choroidal neovascularization. Blue (a) and near-infrared (b) autofluorescence images are very useful to document the distribution and extension of atrophic alterations, hypo- and hyperautofluorescent flecks. Early, intermediate and late phases of FA (c–e) and ICGA (f–h) exams detect the presence of a CNV, associated with sparse diffuse alterations extended also over the vascular arcades. Structural OCT shows an hyperreflective lesion associated with subretinal fluid (i), with complete recovery of exudation with stabilization of lesion size after anti-VEGF intravitreal treatment (j)
Currently the two main drugs used to treat nAMD are Ranibizumab and Aflibercept. Bevacizumab, (Avastin; Genentech/Roche), does not have approval from the US Food and Drug Administration (FDA) and the National Institute for Health and Care Excellence for the treatment of AMD. Clinical trials have demonstrated similar efficacy of Bevacizumab and Ranibizumab. However, questions remain regarding serious systemic and ocular side effects of Bevacizumab. Whereas ranibizumab is provided in single-dose vials, bevacizumab requires compounding, which may increase the risk of ocular infections [18, 19].
Ranibizumab was the first treatment for neovascular AMD that offered a realistic hope for vision improvement.
The registration studies (ANCHOR and MARINA trials) analyzed the use of Ranibizumab versus photodynamic therapy in classic membranes and versus placebo in occult neovascularization. These trials established vision improvement in Ranibizumab groups and vision loss in the comparator groups. FDA approved Ranibizumab for the treatment of AMD in 2007 [20, 21].
Aflibercept, formerly known as VEGF-Trap, is another anti-VEGF drug that was approved for the treatment of nAMD by the FDA in 2011, after the results of VIEW 1 and 2 trials [22, 23]. These studies demonstrated that aflibercept is an effective therapy for nAMD and it can be administered every 2 months [22].
Angiogenesis can be inhibited with different treatments, which have been studied with excellent outcomes in the clinical trials but with less successful results using real-world data. The targets of these new therapies are: better efficacy, longer duration of action and simultaneous effects on VEGF blockade and prevention of atrophy and scarring. Between these new drugs, Brolucizumab and Abicipar pegol showed positive outcomes in phase 2 trials and now are being studied in phase 3 trials [24].
Brolucizumab (RTH258) is a humanized single-chain antibody fragment that inhibits all isoforms of VEGF-A. It was developed by ESBATech (discovery to phase 2a), Alcon Laboratories (phase 2b) and Novartis (phase 3).This drug has a small molecular weight (26 kDa) in comparison to Aflibercept and Ranibizumab (respectively 115 kDa and 48 kDa). This enables Brolucizumab to penetrate tissues and to be removed more rapidly from systemic circulation compared to larger molecules. Two different trials (HAWK and HARRIER) were designed to compare the efficacy and safety of Brolucizumab versus Aflibercept in subjects with nAMD. These studies showed important results in term of visual acuity and duration of action. Moreover, Brolucizumab seemed to be superior to Aflibercept in reducing intraretinal and subretinal fluid by OCT at different time-points [25, 26].
Abicipar pegol (Allergan) is a recombinant protein of the designed ankyrin repeat protein (DARPin) family. DARPins are small, single-domain antibody mimetic proteins that can selectively bind to a target protein with high affinity and specificity. In comparison to Ranibizumab, Abicipar showed a higher affinity for VEGF-A and a longer half-life. SEQUOIA and CEDAR are two clinical trials designed to evaluate the safety and efficacy of Abicipar versus Ranibizumab in nAMD. After 52 weeks both studies achieved non-inferiority in vision compared to Ranibizumab. However, patients treated with Abicipar presented more ocular adverse events following the treatment, most notably in the form of intraocular inflammation as uveitis, vitritis and vasculitis. Most of these episodes were classified as mild or moderate and over 80% of them were treated and responded to topical corticosteroid [27].
Another interesting molecule with anti-angiogenic effects is Squalamine (OHR-102). This drug is delivered to the eye in the form of an eye drop. IMPACT study was performed on this new drug, comparing squalamine eye drops plus Ranibizumab versus Ranibizumab monotherapy. This study showed positive outcomes in term of visual acuity and, at present, a phase 3 study is ongoing to evaluate the efficacy and safety of squalamine eye drops [24].
Diabetic macular edema (DME) is a severe complication of diabetic retinopathy and is characterized by breakdown of the blood-retina barrier and increased vascular permeability.
Currently therapeutic strategies for DME includes focal/grid laser photocoagulation, intravitreal anti-VEGF or corticosteroid treatment [28].
Fluocinolone acetonide intravitreal implant 0.19 mg (Iluvien) is a nonbiodegradable, injectable corticosteroid implant. It has been approved in 2013 as a treatment option of DME in patients with pseudophakia who have been previously treated with corticosteroids and didn’t have a clinically significant rise in intraocular pressure. Iluvien lasts 36 months and contains 0.19 mg of fluocinolone acetonide which is released at an average rate of 0.2 μg/day [29].
The Fluocinolone Acetonide in Diabetic Macular Edema (FAME) registration studies consisted of two identical, double masked, sham-controlled, multicenter, phase 3 studies— trial A and trial B—and included 956 patients with DME. Patients were randomized to receive Iluvien or placebo. Participants who received Iluvien showed a significantly improvement in best-corrected visual acuity (BCVA) than sham injection. The Iluvien implant also significantly reduced foveal thickness at 24 months. These effects last 36 months. Consistent with corticosteroid class-specific adverse events, the most significant concerns in the use of Iluvien are ocular hypertension and cataract. Raised IOP was treated with medical therapy in most patients, with only <5% requiring incisional IOP-lowering surgery. These results have been also supported by real-world studies [30].
Advances in Other Non-surgical Treatments
New perspectives for the treatment of maculopathies regard gene therapy and new technological devices, namely artificial retinal microchips.
Many hopes dwell in gene therapy. The eye has been at the perfect candidate for translational gene therapy because of its small, enclosed structure, immune privilege, and easy accessibility. The availability of animal models and the opportunity to take in vivo imaging techniques allows for noninvasive and sensible monitoring of the effects of gene delivery. Two strategies have been adopted in gene therapy: gene augmentation or gene disrupting/silencing [31]. The former consists in an insertion of a mutant gene in the host cell through a vector. The latter employs editing tools, as RNA interference or nucleases [32, 33], for gene suppression and many studies on animal model are searching for possible applications in human ocular disease. Gene augmentation may be use for AR and XL diseases and is the most experimented strategy worldwide. The gene of interest could be delivered as DNA, or alternatively as mRNA or mRNA analog. Viral vectors represent the preferred choice to transfer nucleic acids in cells. Adenovirus and Lentivirus have the best qualities for this task. Adeno-associated virus is the more used vector in ophthalmology due to its efficiency, persistence and poor immunogenicity [34]. Adeno-associated virus (AAV) belongs to capside virus with no envelope and has small dimension (19–21 nm). AAV has low risk of mutagenesis because it remains in episomal form in the nucleus [35]. AAV is the chosen vector for RPE65 trials [36, 37]. The limiting factor in AAV use is the packaging capacity of 4.8 kb [38]. Moreover, regulatory elements are necessary for the procedure and so the capacity is further reduced to 3 kb [39]. For this reason, large genes as ABCA4 and MY07A (7 kb) could not be inserted in AAV carriers [40]. The use of dual AAV carrier has been developed to overcome the issue [41]. The genetic material is packed as two distinct fragments and delivered to the cell and then recombining through homologous recombination or trans-splicing methodic [42]. The AAV serotypes more studied and used in ocular gene therapy are AAV2, AAV5 and AAV8. Different capsid proteins confer diverse target tropism and transduction efficiency [43–45]. The AAV2 is able to efficiently transduce RPE cells, photoreceptors cells and retinal ganglion cells [43]. Recent studies suggest that the antibody response triggered by a first injection could limit the transduction in the other eye [46].
Lentiviral vectors derive form variant of human immunodeficiency virus type 1 [47]. The cargo capacity is larger than AAV viruses: 8–10 kb [48]. After transduction the gene inserted are reverse transcribed into the host genome and then expressed [49]. Insertional mutagenesis is a risk since the integration. In preclinical studies lentivirus seemed effective in targeting RPE cell, but not photoreceptors [50].
Subretinal and intravitreal injection are the strategies to deliver the virus carriers in the eye. The first method targets RPE cells, photoreceptors and Muller cells. In almost all human clinical trials has been used to deliver RPE65 [51]. Observed complications for subretinal injection are macular hole, retinal tears, inability to detach the retina whit multiple injection attempts, cataract, long recovery time because of large volume injected [36, 52, 53]. Intravitreal injection targets retinal ganglion cells and the virus molecules are directly injected in the hyaloid [54]. Immunologic and inflammatory responses, transient elevation in intraocular pressure are the main complications [55–57].
Nanoparticles (NPs) can also be used for gene therapy alternatively to viral carrier [58]. NPs, usually lipid-based, were developed to be internalized by the cell and to be capable of transferring the carried genetic material to the nucleus, escaping from the endosome. Lipid NPs are stable, biocompatible, no inflammatory-induce molecules [59]. Large plasmid DNA up to 20 kb could be transferred by NPs [60]. Two preclinical studies efficaciously treated a Rs1h deficient X-linked retinoschisis in a mouse model through NPs injections [61, 62]. NPs disadvantages compared to viral carriers are lower gene expression and lack of specificity to the different types of retinal cells [59].
Viral vectors with subretinal/intravitreal injection, and in particular AAV, and gene augmentation strategy have been used for the most completed and ongoing human trials. Voretigene neparvovec-rzyl (trade name of Luxturna) was approved in December 2017 as the first gene therapy for inherited retinal degenerations by Food and Drug Administration. It consists in AAV2 viral vectors carrying human RPE65 DNA driven by a CMV enhancer targeting RPE cells through subretinal injection [63, 64].
Retinitis pigmentosa and Leber’s Congenital Amaurosis (LCA) would benefit from Voretigene therapy. Mutations in RPE65 account for approximately 2% of autosomal recessive RP and 16% of LCA [65]. Results from the first 2 years of this clinical trial showed that RPE65 gene replacement therapy was not associated with serious adverse events, and improvement in at least one measure of visual function was observed in 9/12 subjects, with the trend for improvement being observed in the younger patients [66]. In the 3, 4, 5 years after treatment no safety issues were observed and progression of photoreceptors degeneration was slower in early age patients [67].
Gene therapy must be completed before the onset of cell death caused by the disease. Retinal degeneration progression was seen in a human LCA study with gene therapy, despite a visual acuity improvement [58]. Perfect timing is therefore crucial.
The first multicentre nonrandomised open-label clinical trial in choroideremia patients reported encouraging results using an AAV2 [68]. After 2 years, the median visual acuity increased by 4.5 letters in the treated eyes versus 1.5 letters loss in the untreated eyes [69, 70]. Association with OCTA information may contribute to a more precise localization of gene therapy in the attempt to maximize the functional improvement [71].
Gene therapy approach is an exciting researching field in IRD and an actual clinical option after Voretigene launch in RPE65 mediated diseases. Cargo capacity remains a limiting factor and new researches are needed to find new vectors or modify the current ones. We hope that new data and technological improvement will lead to perfectionated the results in treated patients and extend gene therapy effectiveness to other IRD.
Gene therapy approach may help maintain visual acuity in the early onset of the disease, while electric stimulation through electrodes attempt to a partial restoration of the vision using the remaining retinal network [72]. After photoreceptors degeneration the inner retinal cells may continue to survive and be operative for many years despite neural remodeling [73].
Pros and cons in the choice of epiretinal and subretinal implantation
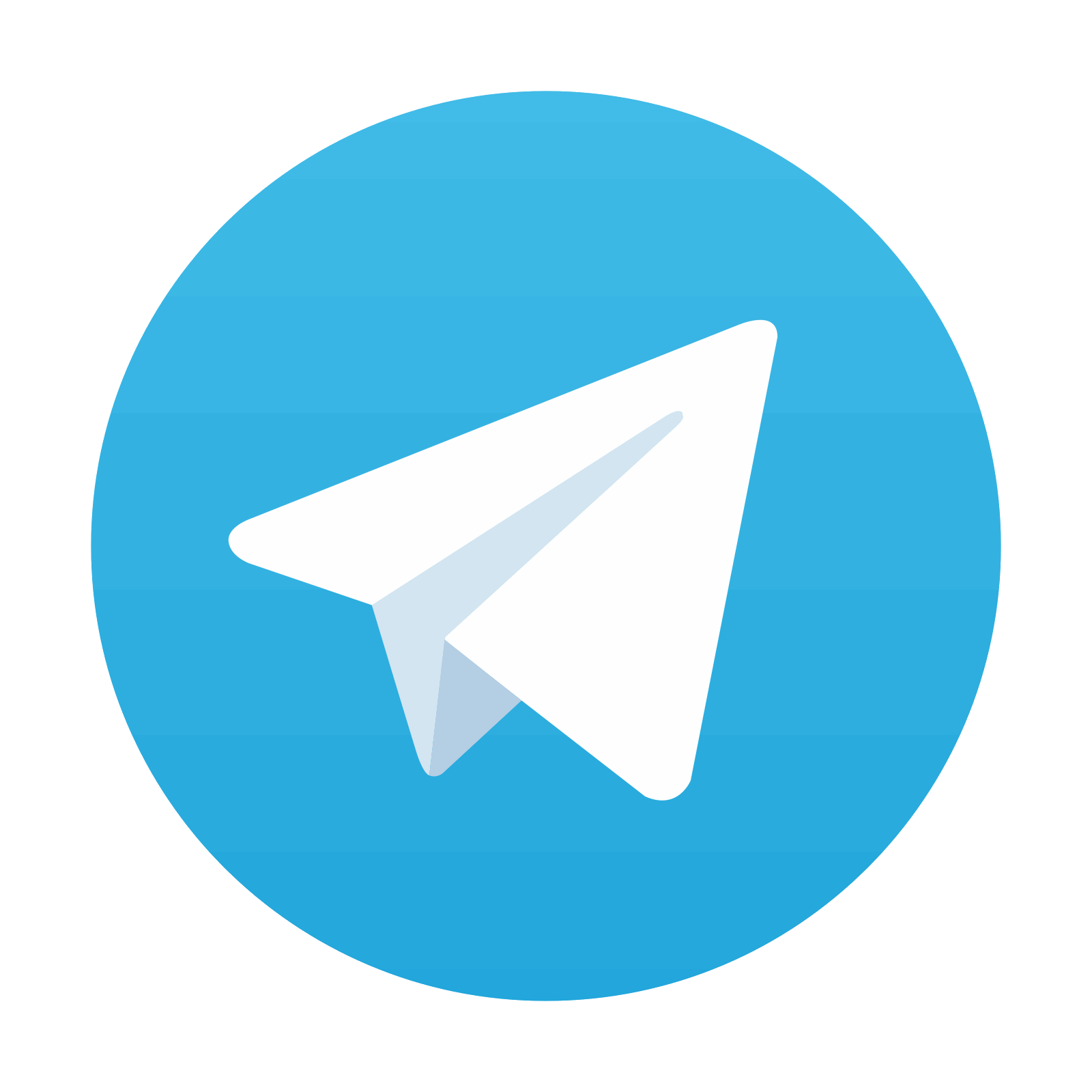
Stay updated, free articles. Join our Telegram channel

Full access? Get Clinical Tree
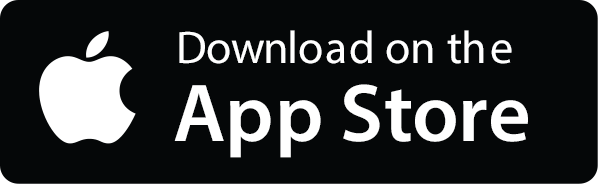
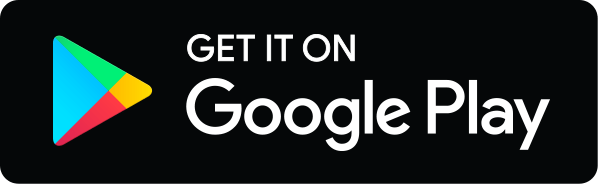