(1)
Department of Otlaryngology Head and Neck Surgery, Graduate School of Medical Sciences Kumamoto University, Kumamoto, Japan
Abstract
The detailed effects of denervation on the intrinsic laryngeal muscles of rats were investigated. After denervation, the entire thyroarytenoid (TA) muscle and individual muscle fibers thereof gradually reduced in size over the next 10 weeks, but unlike muscles of the extremities, the dimensions then remained unchanged to 58 weeks of observation. Although nerve terminals disappeared within 24 h of nerve transection, approximately 70 % of the acetylcholine receptors (AchRs) were preserved at 10 weeks post-denervation. Subsequently, AchR numbers gradually decreased to 35 % of the initial value by 58 weeks after denervation. Nerve–muscle pedicle (NMP) flap implantation into the denervated TA muscle facilitated recovery of bulk muscle size, individual muscle fibers, neuromuscular junctions (NMJs), and function as reflected by evoked electromyography (EMG) testing. Such positive effects were noted even when the NMP procedure was performed 48 weeks post-injury, although treatment effects were less prominent as the time to treatment became extended. The NMP method also yielded favorable results in aged rats and animals in which the TA muscle became partially reinnervated after nerve injury.
Keywords
DenervationReinnervationNerve–muscle pedicle flapMuscle atrophyNeuromuscular junction3.1 Historical Review
3.1.1 Histological Changes in the Laryngeal Muscles After Denervation
Denervation of skeletal muscle decreases the mean cross-sectional areas of individual muscle fibers and reduces muscle size because of degradation, reduced protein synthesis, and apoptosis of muscle fibers [1]. Similar atrophic changes are also evident in the intrinsic laryngeal muscles, although they are not identical to changes in limb muscles. For example, 10 months after denervation, the rat soleus muscle decreased to 3 % of the muscle size on the normally innervated side, and few muscle fibers with transverse striations were evident [2]. Furthermore, prolonged denervation (for up to 7 months) was accompanied by a pronounced decline in the number of myonuclei per muscle fiber in the rat extensor digitorum longus muscle [3]. However, atrophic changes in laryngeal muscles after denervation are considered to be less severe. Shindo et al. [4] explored the changes in canine laryngeal muscles after excision of a segment of the recurrent laryngeal nerve (RLN). The muscles initially underwent atrophy and fibrosis over the next 3 months, after which time partial reinnervation was noted as exemplified by the presence of hypertrophic muscle fibers and scattered areas of atrophy at 9 months. In the interval between 4 and 9 months, vocal fold movement was noted when the severed cranial end of the RLN was stimulated. It was believed that the reinnervation was attributable to regeneration of RLN nerve fibers. Shinners et al. [5] sectioned the RLN of rabbits and found that the number of bromodeoxyuridine (BrdU)-positive myonuclei in laryngeal muscles increased 1 week after denervation. In addition, the level of neonatal myosin heavy chain (MyHC) expression significantly increased until 24 weeks. It was suggested that these observations reflected an increase in satellite cell numbers, contributing to the ability of the laryngeal muscles to survive for extended periods following interruption of the nerve supply.
The process of degeneration has been examined at the neuromuscular junction (NMJ) level in muscles of the trunk and limbs. Winlow and Usherwood [6] reported that motor axon terminals disappeared 26 h after denervation of the mouse phrenic nerve. The number of AchRs in the soleus muscle of adult rats remained normal at 18 days but then fell to 54 % and 35 % of the initial value by 33 and 57 days, respectively. Thus, AchRs in NMJs persisted longer than motor axon terminals [7, 8].
3.1.2 Role of Basic Fibroblast Growth Factor in the Nucleus Ambiguus
The cell bodies of motor fibers innervating the intrinsic laryngeal muscles are located in the nucleus ambiguus (NA) [9–11]. Komori et al. [12] explored regeneration of motor fibers after RLN injury accompanied by administration of nerve growth factor, ciliary neurotrophic factor, or basic fibroblast growth factor (bFGF) at the site of injury and found that bFGF afforded the greatest protective effects in terms of regeneration of myelinated fibers. Moreover, bFGF prevented a decrease in the size of NA motor neurons that was noted in the controls. Sanuki et al. [13] measured bFGF expression levels in the NA after infliction of three different types of nerve injury on adult rats, which included nerve crush (Group A of Fig. 3.1; [Sunderland Grade II injury [14]]; Fig. 3.2) and nerve transection (Grade V injury, Fig. 3.2) without and with placement of caps on the nerve stumps to prevent regeneration (Groups B and C, respectively, of Fig. 3.1). The bFGF level in the NA of Group A was maximal 7 days after injury, whereas that of Group B peaked on day 14. Furthermore, Group B animals exhibited significantly greater immunoreactivity for and a longer duration of bFGF than those in Group A. However, the bFGF expression level in Group C was significantly lower than that in Groups A and B. It was concluded that endogenous bFGF in the NA might contribute to prevention of neuronal death induced by peripheral nerve injury and that blockage of axonal regeneration might suppress such bFGF production. Huber et al. [15] found that the level of messenger RNA (mRNA) encoding bFGF was higher in the hypoglossal nucleus following hypoglossal nerve injury caused by nerve transection rather than nerve crush. It was also found that local administration of bFGF at the site of nerve injury or at the NA prevented neuronal death after nerve transection. Fujimoto et al. [16] found that regenerating axons and Schwann cells promoted local bFGF production at the site of injury. Thus, blockage of axonal flow suppressed bFGF expression in the NA and may negatively affect neuronal survival in general.



Fig. 3.1
Three different nerve injury models: group A, axonotmesis; group B, neurotmesis; and group C, neurotmesis featuring the use of silicone caps covering the severed ends of the recurrent laryngeal nerve (Citation: Ref. [14])

Fig. 3.2
Classification of peripheral nerve injuries according to Sunderland [14]. Grade I injury is sustained when conduction along the axon is interrupted at the site of injury, but no break in the continuity of structures comprising the nerve trunk is evident. Grade II injury is a situation in which the axon is severed, or the axonal mechanisms are so disorganized that the axon fails to survive below the level of the injury. Grade III injury is caused by more severe trauma, which, in addition to causing axonal disintegration and Wallerian degeneration, disorganizes the internal structure of the funiculi. Grade IV injury involves the entire funiculus; all bundles are so breached and disorganized that they are no longer sharply demarcated from the epineurium. Continuity of the nerve trunk is preserved, but the involved segment is ultimately converted into a tangled mass of connective tissue, Schwann cells, and regenerating axons. Grade V injury implies loss of continuity of the nerve trunk, associated with complete loss of motor, sensory, and sympathetic functions in the autonomous distribution region of the severed nerve
3.1.3 Atrophic Changes in Laryngeal Muscle Fibers After Denervation
In general, striated muscles exhibit rapid atrophic changes after denervation [3, 17]. For example, Viguie et al. [3] reported that 2 months after denervation, the weight and contractile force of the rat extensor digitorum longus muscle fell to 31 % and 2 % of preinjury values, respectively. Kobayashi et al. [17] used a rat hind limb model to measure the extent of neuromuscular recovery at different times after nerve reconstruction. Although excellent axonal regeneration was noted irrespective of the denervation period, profound decreases were evident in the recovery of both muscle mass and integrated motor function if reconstruction was delayed for more than 1 month post-injury. It was noted that the extent of progressive change was not proportional to the length of the denervation period, but rather, that significant and more discrete changes developed later than 1 month after denervation and these changes precluded full recovery of muscle mass. Such findings have created discussion on the optimal timing and efficacy of reinnervation procedures used to treat chronic vocal fold paralysis.
Johns et al. [18] measured the isometric contraction force and the mass of the feline TA muscle 6 months after excision of a 3 cm long segment of the RLN and the external branch of the SLN. Isometric force and muscle mass decreased to 93 % and 85 %, respectively, compared to sham-treated animals, but no significant difference in either parameter was evident when denervated and sham-treated animals were compared. Such results suggest that it is possible to restore functional innervation of the TA muscle when reinnervation surgery is performed, even months after primary injury. The cited authors hypothesized that misdirected random regeneration occurred after denervation and that such synkinetic reinnervation may be helpful in preservation of muscle fiber structure and contractile capacity.
3.1.4 Changes in the Human Laryngeal Muscles and the Cricoarytenoid Joint After Denervation
A few histological reports on denervated human laryngeal muscles have appeared. Kirchner reported interesting histological findings in two patients with UVFP caused by jugular foramen syndrome and mediastinal disease who died 6 years and 7 months after the onset of UVFP, respectively [19, 20]. TA and PCA muscles that had been denervated for 6 years had disappeared and were replaced by fibrous tissue [19]. However, the interarytenoid (IA) muscle did not exhibit atrophic changes because of dual innervation from both RLNs [21]. In the patient who had experienced 7 months of denervation, the TA and PCA muscles exhibited severe atrophic and fibrotic changes [20]. Gacek and Gacek [22] histopathologically studied ten human whole-organ laryngeal specimens with histories of long-standing paralysis (from 6 months to 17 years) to determine changes in laryngeal muscles. Muscle fibers were observed in the TA, LCA, and PCA muscles, although the muscles were atrophic in patients with unilateral paralysis. As described in Chap. 1, many animal experiments have confirmed that reinnervation of laryngeal muscles occurs after denervation of the RLN. Thus, it is considered that laryngeal muscles maintain their muscle fibers to a certain extent, thereby sustaining the capacity to accept regenerating nerve fibers and form neuromuscular junctions after injury to the RLN. More work on human larynges denervated for months or years is necessary to confirm this point.
Again, there have been only a few reports on changes in the cricoarytenoid (CA) joint after onset of VFP. Contracture of joint movement develops after paralysis of the extremities if no rehabilitative intervention is applied. However, Gacek and Gacek [22] found that the CA joint maintained a normal histological structure without fibrosis, osseous obliteration of joint space, or degeneration of the articular surface in ten paralyzed human larynges. Müller and Paulsen [23] performed laser arytenoidectomy on ten patients with bilateral VFP 6 weeks to 44 years in duration and histologically examined the joint surfaces of the arytenoid cartilage. In specimens from patients with paralysis 9 and 30 months in duration, changes in the superficial layer were evident with formation of chondrocyte clusters, a decrease in the total cell counts in the upper cartilage layer, and development of a clear demarcation line between that layer and underlying cartilage of normal appearance. Such specimens also exhibited roughness of the joint surface of the cartilage. One specimen from a patient with paralysis 30 years in duration exhibited a fibrous coating in certain areas that covered the underlying cartilage. Although clear structural changes were notable on the articular surface of the arytenoid cartilage, CA joint ankylosis was absent, even in a patient with paralysis 44 years in duration. The absence of such ankylosis was attributed to continuous passive movement of the joint during swallowing and small residual contractions of the laryngeal muscles, which may receive regenerating nerve fibers.
In summary, atrophic changes in laryngeal muscles develop after VFP onset, but the muscle fibers remain intact for a relatively long period of time. The time from onset of VFP to the disappearance of muscle fibers depends on the presence and extent of laryngeal muscle reinnervation. Furthermore, CA joint ankylosis does not develop, even many years after VFP onset. Thus, surgical treatments seeking to adduct the vocal fold and reinnervate the laryngeal muscles are feasible in patients with long-standing VFP. Further work is required to identify the optimal interval from onset of VFP to surgery.
3.1.5 Reinnervation of Denervated Muscle
Functionally efficient regeneration of nerve fibers can be expected after Grade I or II nerve injuries. However, when a nerve injury is worse than Grade III, axonal regeneration is complicated by disruption of the endoneurium and local fibrosis, and functional recovery is rarely expected as the extent of nerve injury increases. In addition, if a nerve is injured at a proximal level, apoptosis of the motor neuron may develop, thereby reducing the numbers of regenerating axons.
Clinical methods used to reinnervate a denervated muscle include direct suturing of the severed nerve ends, interposition of a portion of a foreign nerve between the transected nerve ends, and nerve transfer (Fig. 2.6, Chap. 2). Because the RLN consists of a single funiculus, nerve suturing is performed using the perineural suture technique. Interposition of a piece of foreign nerve is preferable when the gap between the cut ends is longer than 3–5 mm, because tension at the suture site often causes poor axonal regeneration if the suture ruptures unexpectedly and reduces blood flow. Nerve transfer is the method of choice when the cranial end of the nerve is unavailable for reconstruction. Foreign nerve fibers regenerate into the peripheral nerve and can produce neuromuscular junctions [24]. The ansa cervicalis nerve (ACN) is utilized for such a purpose, because that nerve is of a similar diameter to the RLN and is located in proximity to the latter nerve. In addition, the ACN consists principally of motor axons. Reconstruction of the RLN during tumor extirpation from the neck favorably affects vocal function, although vocal fold movement does not recover [25–28].
Any of the abovementioned three methods can be used when the peripheral end of the RLN is available for reconstruction. Figure 3.3 shows four different reinnervation methods applicable to the treatment of denervated muscle when the peripheral end of the nerve is unavailable for reconstruction. Experimentally, implantation of a foreign motor nerve into a denervated muscle (a nerve implant, NI) has been reported to produce new NMJs [29, 30]. In 2007, Su et al. [31] performed NI on the TA muscle of ten patients, using the ACN, to treat paralytic dysphonia and reported that eight patients exhibited improvements in voice quality at the 2-year follow-up. However, the absence of further positive reports on NI has discouraged clinical utilization of the method. Muscular neurotization is a phenomenon whereby one muscle innervates an adjacent denervated muscle via collateral nerve sprouts [32, 33]. Muscle–nerve–muscle (MNM) neurotization is defined as reinnervation of a denervated muscle via axons in a nerve graft that are induced to sprout from motor nerves within an innervated muscle. Reinnervation of the target muscle occurs via these axons. Using animal models, Hogikyan et al. [34] and Debnath et al. [35] histologically and electromyographically demonstrated reinnervation of denervated laryngeal muscles after MNM neurotization. However, neither muscular nor MNM neurotization has been clinically applied for laryngeal reinnervation. Previously, a nerve–muscle pedicle (NMP) flap was used to ameliorate inspiratory distress in patients with bilateral VFP via transfer of an intact nerve with a portion of the muscle supplied by that nerve [36]. This muscle fragment, together with the associated nerve pedicle, is sutured into the paralyzed muscle. Even a small muscle fraction contains a large number of cut nerve fibers, and every cut end branches and regenerates into the denervated muscle to form new NMJs (Fig. 3.4) [37]. Tucker [38, 39] and May and Beery [40] reported improvements in postoperative voice after NMP flap implantation into the TA and LCA muscles, respectively, but the method has not been widely accepted as a treatment for paralytic dysphonia because of the lack of further favorable reports.



Fig. 3.3
Reinnervation methods for denervated muscle when the peripheral end of the nerve is unavailable for reconstruction; these are nerve implantation, muscular neurotization, muscle–nerve–muscle neurotization, and nerve–muscle pedicle (NMP) flap implantation

Fig. 3.4
Reinnervation of a denervated muscle after implantation of a nerve–muscle pedicle (NMP) flap
Frey et al. [32] compared reinnervation efficiency using three different methods (direct suturing, NI, muscular neurotization) of the rectus femoris muscle of the rabbit and showed that both nerve suturing and NI were superior to muscular neurotization. Meikle studied the relative effectiveness of the NI and NMP flap methods using denervated strap muscles of a rabbit model and obtained similar effects using the two reinnervation methods [37]. The cited authors considered that a possible bar to successful reinnervation might arise when the original nerve supply was present but nonfunctional, inhibiting development of newly implanted muscle. Zheng et al. [41] used electromyographic and histological techniques to compare the effects of nerve suturing, NI, and NMP implantation on reinnervation of the canine laryngeal adductor muscles. It was noted that nerve suturing was superior to NI and the use of the NMP technique and that little difference was evident between the outcomes of the latter two methods. In general, denervated muscle fibers can form NMJs at new sites during reinnervation [42]. However, the reinnervation process (growth of nerve fibers and formation of NMJs) may be negatively affected by subclinical regeneration of the RLN back to the laryngeal muscles, because NMJ formation in innervated muscle fibers, using foreign nerve fibers, is inhibited by continuation of the original local innervation [37]. Thus, Zheng et al. [41] suggested that the RLN should be severed prior to application of a reinnervation protocol. The present author explored the effect of NMP flap implantation into the denervated TA muscle of the rat and found that this effectively reduced atrophic changes in the TA muscle even in the presence of partial innervations thereof [43]. Details of this study will be described below, in Sect. 3.3.4.
3.2 Changes in the TA Muscle After Denervation
Injury to a peripheral nerve triggers degeneration and atrophy of muscle fibers and NMJs. Although such changes after muscle denervation have been investigated in the extremities [3, 44, 45], only a few studies on changes in laryngeal muscles after denervation have appeared. This section describes degenerative and atrophic changes in the TA muscle after denervation via transection of the rat RLN.
3.2.1 Short-Term Changes in Muscles
3.2.1.1 The Animal Model
Eight-week-old Wistar rats were anesthetized by intraperitoneal injection of ketamine (50 mg/kg) and xylazine (10 mg/kg). Under an operating microscope, the left RLN was exposed and resected at the level of the seventh tracheal ring, with removal of a 10 mm long segment. The distal end of the nerve was ligatured with 4-0 nylon and the proximal end embedded into the sternohyoid muscle to prevent contact between the two cut ends. The skin incision was closed, and the rats were allowed to recover in an approved animal care facility.
The animals were divided into eight groups defined by the length of the observation period after treatment: seven groups underwent left laryngeal nerve manipulation, and the final group was a non-treatment control group. Six animals in each group were killed by administration of an overdose of pentobarbital at 6, 12, 18, and 24 h and 2, 4, and 10 weeks after treatment. Larynges were isolated, frozen in liquid nitrogen, embedded in an OCT compound, and stored at −80 °C. Coronal sections (7–8 μm thick) of frozen larynges were prepared using a cryostat [46].
3.2.1.2 Areas of the Entire TA Muscle and Individual Muscle Fibers
To evaluate the areas of the entire TA muscle and those of individual muscle fibers, we examined sections within an area approximately 300 μm behind the front edge of the arytenoid cartilage; the sections were stained with hematoxylin and eosin. We matched sections of bilateral TA muscles using the contours of the arytenoid cartilages as guides and measured both TA muscle areas and those of individual muscle fibers (30–50) in three-to-five matched sections from each animal. We calculated the areas between the treated (left, T) and untreated (right, U) sides (deriving a T/U ratio) of the same section. In addition, in the six control animals, the entire muscle areas and those of individual TA muscle fibers were evaluated by comparing data from the left and right sides; the results are shown in Fig. 3.5. The unpaired Student’s t-test was used to perform statistical analysis, and a difference was considered significant at a P value less than 0.05.


Fig. 3.5
Temporal changes in total muscle area (left) and that of individual muscle fibers (right) of the thyroarytenoid (TA) muscle after transection of the left recurrent laryngeal nerve (RLN). All data are presented as means ± SDs (Citation: Ref. [46])
Figure 3.5 shows changes over time in total muscle area and that of individual muscle fibers in a coronal section of the TA muscle after transection of the left RLN. Figure 3.6 shows representative microscopic findings on the TA muscle (hematoxylin and eosin stained) 10 weeks after transection of the left RLN. At 24 h after treatment, neither the entire TA muscle nor that of individual muscle fibers differed significantly from those of the control. In contrast, both of these parameters decreased significantly by 2 weeks posttreatment (P < 0.05), and at 10 weeks, the T/U ratios of the entire muscle area and that of the muscle fibers were 53.2 ± 10.7 % and 55.5 ± 6.8 %, respectively (control: 100 %) (P < 0.01).


Fig. 3.6
Representative microscopic findings on the thyroarytenoid (TA) muscle (upon hematoxylin–eosin staining) 10 weeks after transection of the left recurrent laryngeal nerve. (A, C) the untreated right side and (B, D) the treated left side. Scale bars: A and B 1 mm; C, D 50 μm (Citation: Ref. [46])
3.2.1.3 The Neuromuscular Junction
We selected sections from the area embracing the middle one-third of the thyroid cartilage, because this region is rich in NMJs. An NMJ features pre- and postsynaptic structures, interrupted by a synaptic cleft (Fig. 3.7). Synaptophysin is a protein constituent of the presynaptic vesicle membrane [47], and thus, motor axon terminals can be histologically visualized via immunostaining for synaptophysin. Alpha-bungarotoxin (α-BTX) exhibits high affinity for the AchRs of skeletal muscle; therefore, motor endplates can be visualized using fluorescently labeled α-BTX [48]. The structures described above interact to form and maintain NMJs during denervation and reinnervation.


Fig. 3.7
Schematic drawing of the structure of the neuromuscular junction
The number of synaptophysin-positive nerve terminals and α-BTX-positive AchRs in the entire TA muscle from the T (left) and U (right) sides of eight randomly selected sections from a single animal were counted. Next, the ratios of the numbers of synaptophysin-positive nerve terminals or α-BTX-positive AchRs on the T and U sides were determined, and T/U ratios were calculated. The ratios obtained from all 48 sections of each experimental group were averaged.
In the normal TA muscle, synaptophysin-immunoreactive sites and α-BTX-stained sites matched well. At 24 h, the number of α-BTX binding sites did not significantly differ between the two sides. However, the number of α-BTX binding sites decreased significantly (P < 0.05) at 10 weeks posttreatment (to 70.5 ± 12.4 % that of the control) (Figs. 3.8 and 3.9). The number of nerve terminals decreased significantly (P < 0.01) by 18 h (45.2 ± 6.8 %), and such terminals disappeared by 24 h posttreatment.



Fig. 3.8
Temporal changes in the numbers of nerve terminals (black bars) and acetylcholine receptors (AchRs) (white bars) in neuromuscular junctions of the thyroarytenoid muscle in a control group and in a group that underwent recurrent laryngeal nerve (RLN) transection. All data are presented as means ± SDs (Citation: Ref. [46])

Fig. 3.9
Serial sections of the rat thyroarytenoid (TA) muscle from control animals and from test animals taken at 12 h, 24 h, 4 weeks, and 10 weeks after transection of the recurrent laryngeal nerve (RLN). Neuromuscular junctions (NMJs) are doubly stained with fluorescein isothiocyanate-labeled synaptophysin and rhodamine-labeled α-bungarotoxin (BTX) (Citation: Ref. [46])
Such rapid degeneration of presynaptic nerve terminals after denervation of peripheral nerves has been studied [6]. On the other hand, AchRs persist for a relatively long period compared to nerve terminals [7, 8]. For example, the number of AchRs in the soleus muscle of the adult rat was normal to 18 days post-denervation and then fell by 65 % by 57 days [8]. We found no significant decrease in AchR numbers until 4 weeks after RLN transection, and even at 10 weeks, approximately 70 % of AchR reactivity was preserved. Thus, compared to the muscles of the extremities, AchR degradation in the TA muscle after denervation occurs gradually over 10 weeks in rats.
3.2.2 Long-Term Changes in the TA Muscle
Only a few studies have explored long-term muscle atrophy after denervation; this is an important question, because the presence of muscle fibers is a prerequisite for successful reinnervation. In addition, since AchRs are targeted by regenerating axon sprouts during reinnervation, muscle fibers must retain AchRs to accommodate the new connections. In theory, higher numbers of preserved AchRs should lead to more efficient reinnervation. In this section, local effects upon long-term denervation of the TA muscle of rats will be described. The method used was described in Sect. 3.2.1. Observations were made 10, 18, 26, 42, and 58 weeks after denervation, and each group contained six animals [49].
3.2.2.1 Areas of the Entire TA Muscle and Individual Muscle Fibers
The T/U ratios of the entire muscle area and individual muscle fibers fell significantly by 10 weeks post-denervation (P < 0.01) compared to the control group; such changes were noted in all denervation groups. These two indices were relatively similar, regardless of the denervation period, ranging from 61.1 to 72.5 % and 45.0 to 51.9 %, respectively (Fig. 3.10). Atrophy of the TA muscle and proliferation of connective tissue around and between the fibers of that muscle were evident on the treated side (Fig. 3.11). Fibrotic connective tissue accounted for a more prominent decrease in individual muscle fibers than in the entire muscle bulk.



Fig. 3.10
Temporal changes in total muscle area and that of individual muscle fibers in coronal sections of the thyroarytenoid (TA) muscle prepared after transection of the left recurrent laryngeal nerve (RLN). All data are presented as means ± SDs (Citation: Ref. [49])

Fig. 3.11
Representative microscopic findings on the thyroarytenoid (TA) muscle (after hematoxylin–eosin staining) 58 weeks after transection of the left recurrent laryngeal nerve (RLN). (A, C) untreated right side and (B, D) treated left side. Scale bars: A and B 200 μm; C and D 20 μm (Citation: Ref. [49])
3.2.2.2 The Neuromuscular Junction
In our comprehensive study, we found that nerve terminals were not evident at the low magnification (50×) used in our short-term work, whereas such terminals were counted under a higher magnification (200×) in the long-term study. Thus, the numbers of nerve terminals evident 10 weeks after denervation were greater in the long-term study than in the short-term study. The T/U ratios of nerve terminals and AchRs were significantly lower in the denervation groups than in the control group. Both indices continued to fall after prolonged denervation, namely, at 10 and 58 weeks (being 26.3 ± 4.1 % and 76.3 ± 9.0 % at 10 weeks; 13.4 ± 11.9 % and 35.3 ± 20.2 % at 58 weeks) (Fig. 3.12). Furthermore, the number of synaptophysin-positive sites that also stained with α-BTX (i.e., AchRs) was compared to the number of total observable AchRs on the treated side. The ratios in the denervation groups were significantly smaller than that of the controls. However, the index values (which ranged from 30.3 to 35.6 %) did not differ significantly among the five denervation groups (Fig. 3.13). Figure 3.14 shows representative microscopic findings on the TA muscle, specifically stained for nerve terminals and AchRs at 10 and 58 weeks after RLN transection.




Fig. 3.12
Temporal changes in the numbers of nerve terminals (black bars) and acetylcholine receptors (AchRs) (white bars) in neuromuscular junctions of the thyroarytenoid muscle in a control group and after recurrent laryngeal nerve (RLN) transection. All data are presented as means ± SDs (Citation: Ref. [49])

Fig. 3.13
Temporal changes in the ratios of the numbers of nerve terminals compared to those of acetylcholine receptors (AchRs) in the left thyroarytenoid (TA) muscle after left recurrent laryngeal nerve (RLN) resection. Shaded bars: untreated sides. All data are presented as means ± SDs (citation: Ref. [49])

Fig. 3.14
Representative microscopic findings on the rat thyroarytenoid (TA) muscle in control animals and in test animals 10 and 58 weeks after transection of the recurrent laryngeal nerve (RLN). Neuromuscular junctions (NMJs) were doubly stained with fluorescein isothiocyanate-labeled synaptophysin and rhodamine-labeled α-bungarotoxin (BTX) (Citation: Ref. [49])
3.2.2.3 Discussion
Among the denervated groups, the average T/U ratios for the entire TA muscle area and that of individual muscle fibers did not significantly change from 10 to 58 weeks post-injury. Furthermore, the ratios of nerve terminal to AchR numbers did not vary at the different time points. However, although nerve terminals were present in the NMJs of all groups, the T/U ratios of nerve terminals and AchRs were significantly lower at 58 weeks than at 10 weeks.
We believe that a denervated TA muscle may be reinnervated by the original resected RLN [4, 18], autonomic nerves [50], superior laryngeal nerve [51], or other nerves. Such reinnervation may preserve muscle fibers both morphologically and functionally. Johns et al. [18] reported that the maximal isometric force of the feline TA muscle did not change for 6 months after RLN resection. It was hypothesized that the contractile force was preserved via spontaneous reinnervation of the resected RLN. In contrast, in the rat gastrocnemius muscle, the contractile force was significantly impaired 1 month post-injury [17]. Other studies on laryngeal muscles revealed that AchRs were preserved for several weeks after denervation in rats. However, long-term observations over 58 weeks showed that the number of AchRs decreased gradually after denervation. Thus, the ability of a denervated TA muscle to receive regenerating nerve axons may gradually decline, and a reinnervated muscle may fail to recover sufficient function after prolonged denervation. Because the life expectancy of rats is 2.5–3 years, the presence of AchRs and functional muscle fibers 58 weeks after denervation in this animal model suggests that human laryngeal muscles might retain the ability to accommodate regenerating axons with formation of functional connections for several years after VFP onset.
3.3 Nerve–Muscle Pedicle (NMP) Flap Implantation into the TA Muscle
Implantation of an NMP flap using the ACN has been clinically applied to reinnervate PCA and TA muscles when the peripheral end of the RLN could not be located because of severe cicatricial changes [38, 39, 52]. However, the question of whether NMP flap implantation can be used to reinnervate denervated laryngeal muscles remains controversial, even in the experimental context. Tucker’s group [53, 54] reported that reinnervation of denervated PCA muscles via NMP flap implantation was effective. Anonsen et al. [55] performed strap muscle NMP transfer to the contralateral denervated strap muscle of rabbits and demonstrated functional reinnervation both electromyographically and histochemically. Fata et al. [56] examined the outcomes of ansa cervicalis NMP flap implantation into denervated feline PCA muscle using a glycogen depletion method and demonstrated successful reinnervation. However, Rice et al. [57] and Crumley [58] implanted NMP flaps into the PCA muscles of cats and dogs and found no evidence of reinnervation upon histological and electromyographic examination. The ineffectiveness of NMP flap transfer was attributed to development of local fibrosis that interfered with the growth of sprouting nerve fibers toward target muscle fibers.
In this section, the outcomes of NMP flap implantation immediately after denervation of the rat TA muscle will be described. We explore three specific issues that were not evaluated in previous studies; these are modulation of atrophic processes within a denervated TA muscle, re-formation of NMJs, and changes in myosin heavy chain (MyHC) composition of the muscle.
3.3.1 Immediate Implantation
The rat RLN was transected on the left side and managed as described in Sect. 3.2.1.1 (denervation-alone animals are referred to as the DNV group). Subsequently, other animals with transected left RLNs underwent NMP implantation. Under an operating microscope, the ACN was identified and followed until the nerve entered the sternohyoid (SH) muscle. The ACN was electrically stimulated to confirm that the SH muscle was innervated by that ACN. A branch of the ACN, together with a 0.5 × 0.5 × 0.5-mm piece of SH muscle, was harvested and implanted into the TA muscle through a small window constructed in the thyroid cartilage. The NMP flap was firmly sutured to the surface of the TA muscle using 10-0 nylon (Fig. 3.15). After completion of this procedure, the skin incision was closed, and all rats were allowed to recover in an approved animal care facility. After 2, 4, and 10 weeks (six animals per group), the rats were killed by intraperitoneal administration of an overdose of pentobarbital. The muscle areas and NMJ numbers were evaluated as described in Sect. 3.2.1.


Fig. 3.15
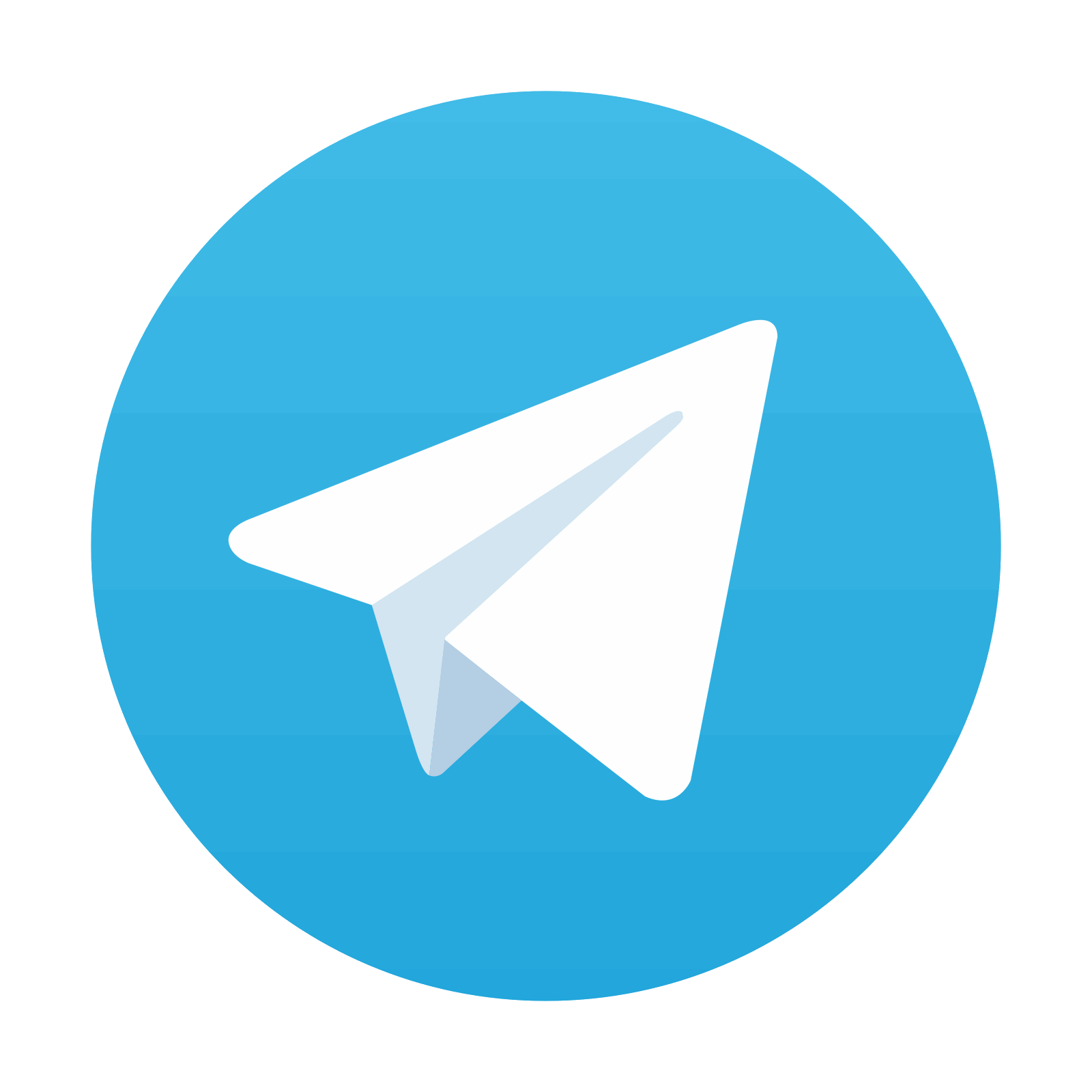
Nerve–muscle pedicle flap implantation. Left: The nerve–muscle pedicle flap is sutured with 10-0 nylon to the exposed thyroarytenoid (TA) muscle through a window (W) created in the thyroid cartilage. The implanted flap passes through the window. Scale bar: 1 mm. Right: Representative histology of a coronal section of a rat taken 10 weeks after nerve–muscle pedicle (NMP) implantation. The arrowhead indicates the site of NMP transplantation. Scale bar: 1 mm. W window made in the thyroid cartilage, SH the sternohyoid muscle (Citation: Ref. [59])
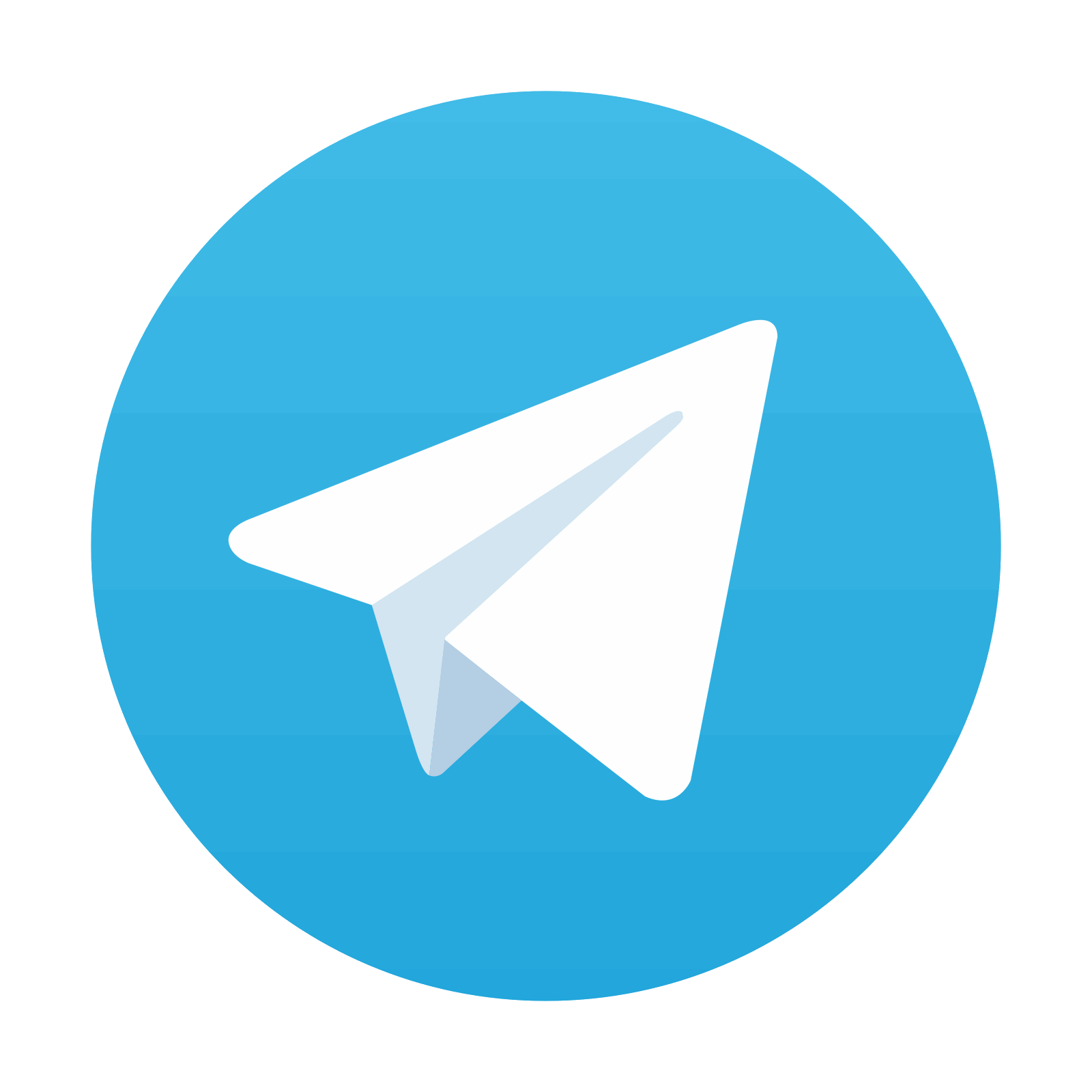
Stay updated, free articles. Join our Telegram channel

Full access? Get Clinical Tree
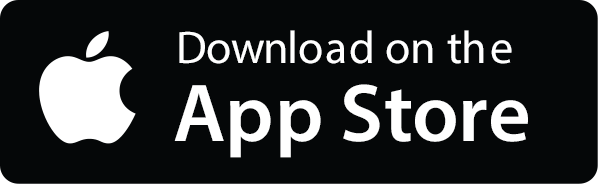
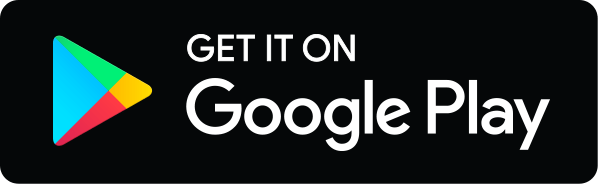
