Fig. 1.1
Tissue Engineering Triad
1.2 Approaches to Research in Regenerative Medicine
There are several approaches to research in regenerative medicine but this paper will categorize them into in vitro and in vivo research. Tissue engineering began in the 1970s and its concept was established in the early 1990s [1–3]. The original concept was based on the in vitro approach. In other words, the three aforementioned elements of tissue engineering were prepared and cultured in vitro. The target tissue was regenerated ex vivo up to some stage and then implanted in the living body. This method can be considered the ultimate regeneration method because mass production will be possible if the process is established. Presently, this method has been implemented only for regeneration of skin [4] and cornea [5]. It is difficult to use this method in some organs, particularly structurally large solid organs (such as liver, kidney, and pancreas). Although there is research in hybrid artificial organs using xenogeneic and allogeneic functional cells, such organs have not been used in clinical application. Okano et al. developed cell sheet engineering [6, 7] using temperature-responsive polymers. Cell sheet engineering is a field of study which enables regeneration of organ with local damage and has wide-ranging applications. Conventional methods posed many difficulties for tissue repair, including the need for enzymatic treatment to collect cultured cells, resulting in the destruction of adhesion between cells and therefore of the network of cells. Thus, cell clusters had to be implanted in pieces, and the cells needed to reconstruct a network at the recipient site. When cells are cultured as a cell sheet, tissue repair can be performed by affixing the sheet to the injury site. The application of this method has been studied for tissue repair of cornea, periodontal tissue, esophagus, lung, heart, liver, and pancreas.
Regeneration using an in vivo approach involves direct implantation of the aforementioned three elements in a living body. This method is divided into two categories depending on the size (presence or absence) of the morphologically damaged site. In other words, if there is a small or no defect, the scaffold still remains. Therefore, it is unnecessary to implant extracellular matrix as a scaffold. Thus, only implantation of cells, or implantation of cells and administration of regulatory factors, is needed. This situation applies to bone marrow transplant [8] and transplant of embryonic nigral cells (dopamine-producing cells) for Parkinson’s disease [9, 10]. It also applies to cell transplantation for myocardial infarction [11]. If there is a large defect, regeneration is required not only at the cellular level but also at the level of organ structure. That is, a scaffold is needed for cell growth as well as for structure to maintain morphology of the organ itself. For example, tracheal morphology is maintained in tracheal regeneration using the basic structure of a polypropylene Marlex mesh reinforced with a spiral of polypropylene wire. This basic structure is coated with collagen sponge as the artificial extracellular matrix [12].
1.3 Field Theory and In Situ Tissue Engineering
As mentioned previously, a living body has a natural ability for regeneration. However, if this ability is inhibited due to some reason, then regeneration ceases. For example, a living body responds to an injury to a tissue or organ, resulting in a partial defect. The injury triggers a signal for regeneration, and simultaneously the following urgent responses occur; through these responses, the body tries to protect itself by quickly isolating the injured site from its surroundings:
1.
Hemostasis by platelet aggregation and vasoconstriction, and phagocytosis of necrotic tissue by macrophage
2.
Contraction of granulation tissue composed mainly of collagen secreted by fibroblasts
3.
Changing of granulation tissue into scar tissue, and the resulting stability of the wound
These responses degrade the scaffold for regeneration depending on the site and depth of the defect. As a result, regeneration of tissue that normally occurs does not. In such a case, if cells of the target tissue or organ still remain, then regeneration can proceed by securing a space for the scaffold before invasion of fibrotic connective tissue. That is, while isolating the defect site from its surroundings, an extracellular matrix is supplied from an external source to secure a scaffold for regeneration. If the amount of cells is insufficient, then cells are also supplied from an external source. In any case, it is essential for regeneration to have a site with a favorable environment, namely a rich blood supply, and to have a certain amount of time. In other words, it is important to create an optimal regenerative environment that allows the damaged tissue or organ to show its maximal, natural regenerative ability. The optimal environment enables regeneration to proceed in accordance to the situation. This concept is the “field theory.” The method of in situ tissue engineering is based on this “field theory.” In this method, it is not always necessary for all three aforementioned elements (cells, scaffold, and regulatory factors) to be supplied from external sources. An external source can supply only one or two elements to the regeneration site in the living body. For example, an external source can supply scaffold alone, scaffold and cells, or cells and regulatory factors. The remaining element or elements can be provided by the body itself, enabling tissue regeneration [12–15]. However, it does not mean that all tissues and organs can be regenerated by this method, and this method is indicated only in local regeneration of specific organs. In many cases, regenerative therapy that is currently performed is based on this field theory.
1.4 Cells
Cells are the main players in regenerative medicine. Since cells are the basis of tissue regeneration, they are essential in regeneration. Cell transplantation is not always necessary if the amount of cells is sufficient at the site for regeneration. However, if one cannot expect sufficient cells to be supplied by their surroundings, then one must administer the cells supplied by an external source. The methods of administration are local administration and systemic administration. Local administration includes a method in which the cells are cultured in vitro and tissue regeneration is allowed to proceed to a certain degree before implantation into the body. The cells for implantation must be cells that actively grow in the living body and perform tissue regeneration. If cells are completely mature and cannot grow, they cannot fulfill this role. Therefore, cells suitable for implantation are stem cells and tissue stem cells. Stem cells have two basic characteristics, but there are various types of stem cells, differing in the degree of differentiation:
1.
Ability to differentiate into two or more different types of cells
2.
Self-renewal ability
Embryonic stem (ES) cells are the most upstream stem cells and are totipotent. In principle, a single ES cell can give rise to all cells, and thereby all tissues of the organism. Somatic stem cells (tissue stem cells) are slightly more downstream compared to ES cells and have a considerably clearer direction of differentiation.
1.4.1 ES Cells
Human ES cells are cultured from tissue called the inner cell mass of the blastocyst (5 days after fertilization), which is formed by division of a fertilized egg. In 1998, Thomson et al. successfully isolated human ES cells [16]. It is very difficult to maintain human ES cells compared with ES cells of other animals such as mice. When ES cells are maintained, the cells do not merely grow but they maintain the totipotency of the original ES cells as they continue to be cultured. ES cells divide spontaneously at a certain rate under normal culture conditions. Therefore, they cannot be maintained in their undifferentiated state [15]. A cytokine that maintains the undifferentiated state of ES cells, called a leukemia inhibitory factor (LIF), has been identified in mice. However, this type of factor has not yet been discovered in humans. Presently, undifferentiated human ES cells can be maintained by culturing them on a feeder cell layer or by culturing them in laminin-coated cultureware containing culture supernatant of feeder cells [17]. Cells such as mouse embryonic fibroblasts (MEFs) have been used as feeder cells.
When ES cells are used for tissue regeneration, they give rise to teratoma if the cells are transplanted as they are. Therefore, induction of differentiation must be performed to a certain extent, and subsequently the target cells are selectively harvested, grown, and eventually transplanted. In mice, the methods for induction of differentiation include a method that involves embryoid body (EB) formation and a method that involves coculturing with stromal cells. ES cells have a characteristic of adhering to walls of cultureware. However, these cells form a mass when grown in suspension culture that does not allow them to adhere to cultureware. The method of EB formation uses such a tendency of ES cells. Differentiation in this mass spontaneously progresses. This mass includes tissue progenitor cells of all germ layer lineages (ectoderm, mesoderm, and endoderm) and stem cells [18]. This mass is called an embryoid body, which differentiates into specific cells after stimulation with growth factors and cytokines. These cells are selectively grown and used in cell transplantation [19, 20]. The method of coculturing with stromal cells involves bone marrow stromal cells that maintain differentiation and growth of hematopoietic cells. When ES cells are cocultured with these stromal cells, induction of differentiation can occur in a certain direction [21, 22]. This technique to induce differentiation is still insufficient for humans. However, there have been reports on induction of differentiation of human ES cells into cardiomyocytes [23], neural stem cells [21], and vascular endothelial cells [24]. In the future, techniques will likely be established to produce progenitor cells and tissue stem cells for various organs.
1.4.2 Mesenchymal Stem Cells
Mesenchymal stem cells (MSCs) are a type of somatic stem cells. MSCs together with vascular and hematopoietic stem cells were discovered in bone marrow stroma. Human MSCs are known to express the following surface protein markers: CD106, CD166, CD29, CD105 (SH2), CD73 (SH3/4), CD44, CD90 (Thy-1), CD71, and Stro-1. However, MSCs are negative for CD31, CD18, CD56, CD45, CD34, CD14, and CD11.
Mesenchymal stem cells can be isolated from bone marrow, which is collected by bone marrow aspiration. The primary culture is grown from the bone marrow aspirate and the suspension cells are removed to obtain cells that adhere to and grow on the bottom surface of the cultureware. The resulting cells are bone marrow stromal cells (BSCs). These cells support the microenvironment in the bone marrow and control the differentiation and growth of hematopoietic cells. MSCs are included in BSCs. MSCs can differentiate into appropriate progenitor cells, which in turn differentiate into mesodermal tissues such as bone, cartilage, muscle, adipose tissue, tendon, ligament, connective tissue, and cardiac muscle [25, 26]. Depending on the conditions of induction, MSCs also have the ability to differentiate into ectodermal tissue such as neurons [27] and epithelial cells, or into endodermal cells. MSCs are present in multiple tissues in the body. In recent years, MSCs have been isolated from various sites (other than from bone marrow) such as adipose tissue, amniotic fluid, periosteum, and fetal tissue [28, 29]. MSCs are known to differentiate into various cells, including cells of mesodermal tissues, in response to a trigger such as trauma, and there are reports that MSCs are involved in tissue regeneration [29–31]. It has also been reported that when bone-marrow-derived mesenchymal stem cells are implanted into mice, the cells differentiate into various organs and become engrafted [27]. These findings indicate that MSCs derived from bone marrow or adipose tissue can be selectively grown, cultured under certain conditions, and induced to differentiate into specific tissue, enabling tissue regeneration. In addition, successful regeneration has been reported in which multipotency of MSC was used and cells were directly implanted in the injured tissue to simultaneously regenerate multiple tissues (vocal cords) [32].
The greatest advantage of mesenchymal stem cells is that they are easy to harvest from autologous tissue. These cells can be said to be the easiest stem cells to use in local regeneration of injured tissue.
1.4.3 iPS Cells
In 2006, Yamanaka and Takashi et al. created undifferentiated pluripotent cells, iPS cells. They generated iPS cells from mouse skin fibroblasts by the retroviral transduction of four genes (Oct3/4, Sox2, Klf4, and c-Myc) called Yamanaka factors [33]. The iPS cells have been considered to be comparable to ES cells. Subsequently, Yamanaka et al. also generated human iPS cells [34]. Thereafter research on iPS cells has rapidly advanced. These cells have been generated by insertion of different types of genes, the use of different number of genes, and the use of different methods for gene transduction. As a result, the efficiency of iPS cell generation has improved compared with the initial method. Malignant transformation has been the greatest problem when the cancer-related gene c-Myc has been used. A method was developed which used the Glis1 gene and all Yamanaka factors, except for c-Myc. This method avoided the problem of c-Myc-associated malignant transformation and improved the efficiency of iPS cell generation [35].
The greatest significance of successful iPS cell generation is that it broke the preconceived notion that “completely differentiated somatic cells cannot be reprogrammed.” Since the generation of iPS cells, there has been advancement in research on their clinical application. In particular, Takahashi et al. has successfully induced differentiation of autologous iPS cells into retinal pigment epithelial cells. This effort was the first clinical application of iPS cells, and was used for the treatment of age-related macular degeneration (wet type). The study used a cell sheet composed of morphologically and functionally mature retinal pigment epithelial cells, which were derived from human iPS cells [36]. There has been much anticipation in the field of drug discovery, involving disease models using iPS because results can be obtained quickly.
A current approach to clinical application of new drugs involves finding drug candidates that target the molecules causing the disease, performing molecular modifications, and further narrowing down the candidates. This process uses mainly animal-derived cells. However, the use of human iPS cells enables researchers to narrow down drug candidates to effective compounds at the initial stage of this process and enables faster drug development. In addition, the use of human iPS cells enables accurate and efficient evaluation of the effectiveness and safety of the drug candidates. For example, studies have already begun using cardiomyocytes generated from iPS cells to lower the risk of side effects. There is also a report on anti-amyloid-beta drug screening using human iPS-derived neurons for the treatment of Alzheimer’s disease. Amyloid-beta is a protein considered to be the causative agent of Alzheimer’s disease, and this screening detects the accumulation of amyloid-beta [37]. More applications of iPS cells are expected in the future [38].
1.5 Problems of Cell Transplantation
As mentioned above, if stem cells are used in regenerative therapy, it is necessary to use appropriate cells as a source depending on which tissue needs to be regenerated. However, not all somatic stem cells and progenitor cells have been discovered. In the preliminary step of cell transplantation, it is generally necessary to harvest the target cells and allow them to differentiate and grow to generate the required amount of cells.
The differentiation and proliferation potential is more inferior in the existing somatic stem cells and progenitor cells than in ES cells. Thus, it is difficult to obtain sufficient amounts of somatic stem cells and progenitor cells for tissue regeneration. If ES cells can be induced to differentiate using various methods to generate somatic stem cells or tissue-progenitor cells of the target organ, tissue regeneration can be performed efficiently and on a large scale by implanting these cells. However, there are problems that need to be resolved, including immunological rejection and ethical issues associated with the harvesting and use of ES cells. ES cells can be said to be the source of life and enable the creation of a complete human being. The current source of human ES cells is the surplus embryos that have become unnecessary after infertility treatment. The use of such embryos has been approved by considering this situation as a separate issue from abortion and questions such as at what point life begins. Thus, major issues remain such as the significance of debating the use of ES cells. Another major problem is immunological rejection that is associated with the use of ES cells harvested from surplus embryos. A method has been devised to generate ES cells from cloned embryos to address these issues [39]. Such cloned embryos are created by transfer of the recipient’s somatic nucleus into the enucleated unfertilized egg, and ES cells are obtained from these cloned embryos. However, there is no guarantee that these ES cells are the same as ES cells obtained from a conventional method. That is, the ES cells might not be the same because the transplanted somatic nucleus might not be completely reprogrammed in the process of establishing the ES cell system from cloned embryos. Furthermore, there are still bioethical issues associated with the use of ES cells obtained from cloned embryos and such use is prohibited in many countries.
These problems can be avoided in the transplantation of mesenchymal stem cells directly harvested from autologous bone marrow. Thus, these mesenchymal stem cells are promising, and various research studies are in progress on induction of differentiation into various tissues [40–42]. Bone marrow is the source of mesenchymal stem cells, and there are various cells in the harvested bone marrow. Mesenchymal stem cells need to be selected from these cells and transplanted after they are grown or after induction of differentiation. A problem that arises at this point involves how to select mesenchymal stem cells. The most common method is selection by cell surface antigen and involves cell sorting using a fluorescence-activated cell sorter (FACS). In this method, cells with fluorescently labeled antibodies are irradiated with a laser beam and the emitted fluorescence is detected. An oscillograph is used to show the distribution of cells by the difference in the intensity of fluorescence, and this method enables fractionation of cells. However, the most significant drawback of this method is that surface markers of mesenchymal stem cells have not yet been completely established. Although opinions differ by author, the established surface markers of mesenchymal cells are considered to be those previously mentioned [43–45]. Mesenchymal stem cells can be purified by long-term selective culture under certain conditions. However, this method is still in the experimental stage, and it is unknown whether it can be applied clinically.
Another problem has to do with the low number of mesenchymal stem cells. The proportion of mesenchymal stem cells among bone marrow stromal cells is said to be 1/10,000 in newborns and 1/400,000 in adults in their 50s, and 1/2,000,000 in adults in their 70s. Thus, the number of mesenchymal stem cells is very low in the bone marrow itself. Furthermore, mesenchymal stem cells undergo senescence, in which the cells no longer proliferate, after continued subculturing. Although ES cells have infinite division potential, senescence occurs in somatic stem cells which are downstream of ES cells [46]. As mentioned earlier, tissue regeneration using somatic stem cells can be performed by different methods. One method involves transplantation of stem cells as they are in the tissue or transplantation by systemic administration. Another method involves induction of differentiation of a certain cell lineage (targeting) and transplantation of these cells. In general, clinical application requires a large amount of progenitor cells of the specific target lineage. Thus, the aforementioned senescence becomes a problem.
When cells divide, they replicate chromosomal DNA. Normal DNA replicase cannot completely replicate the telomeric sites at both ends of the linear DNA genome. Therefore, the telomere length becomes shorter as cells repeatedly divide, and cell division ends when the telomere is no longer maintained. That is, cell proliferation ends at this point, and the number of cells does not increase thereafter. To maintain infinite division potential of cells, one can immortalize the cells by inserting the telomerase reverse transcriptase gene into the gene that controls this replication. Telomerase reverse transcriptase increases telomerase activities [47, 48]. When immortalized cells are generated in this way and transplanted, there is a risk of tumorigenesis, including cancer, in the long term. Furthermore, it has been reported that cells from long-term culture of mesenchymal stem cells themselves became cancerous [49]. Therefore, evaluation of safety for clinical application has become difficult.
iPS cells have been generated from autologous skin, muscles, and fat as research also advanced in ES cells and mesenchymal cells. The efficiency of iPS cell generation is low and these cells are difficult to maintain. These problems are similar to those in ES cells. While the greatest disadvantages of ES cells are ethical issues and immunological rejection, iPS cells do not have these issues. However, iPS cells have problems such as malignant transformation in the process of differentiation and a low efficiency of iPS cell generation. More research needs to be done. ES cells, mesenchymal stem cells, and iPS cells have been discussed above, and each type of cell has advantages and disadvantages. It is important to use appropriate cells for a particular disease and regenerative environment.
1.6 Scaffold
In vivo, cells exist in an extracellular matrix called scaffold. The exceptions are cells that exist singly such as hematopoietic cells. That is, cells adhere to the extracellular matrix and maintain tissue and organ morphology. In many cases, when tissue is injured, a defect of its scaffold develops. Thus, it is necessary to provide a scaffold for regeneration to occur. An ideal scaffold has the following characteristics:
1.
Has high tissue compatibility and does not cause immunological rejection as a foreign substance
2.
Is porous to facilitate invasion of cells and supplying of oxygen and nutrients
3.
Maintains its shape for a certain period of time, and prevents invasion of tissue (such as connective tissue) from the surroundings which would deprive the cells a place for regeneration
4.
Degrades when tissue regeneration is complete
Current scaffolds are made of biopolymers, synthetic polymers, and inorganic compounds and include the following:
Biopolymeric materials: collagen, gelatin, glycosaminoglycan, and chitosan
Synthetic polymeric materials: polyglycolic acid, polylactic acid, poly(lactic-co-glycolic) acid, poly(lactic acid-co-ε-caprolactone), and poly-ε-caprolactone
Inorganic compounds: hydroxyapatite, tricalcium phosphate, and calcium carbonate
Among the aforementioned materials, collagen is a protein that is widely distributed in the body as an extracellular matrix. Collagen is a superior material as a scaffold for cell adhesion and growth and has wide-ranging applications. It has begun to be used clinically as artificial dermis [4], artificial tracheas [12], and artificial nerve conduits [13, 50]. Polyglycolic acid has been used as a protective membrane to prevent invasion of the surrounding tissue and to secure a space for regeneration. It has been applied clinically in artificial nerve conduits [13, 50] and guided tissue regeneration (GTR) membranes for periodontal tissue regeneration [51]. It has also been used in membranes for immunoisolation [52].
As mentioned earlier, a cell sheet developed by Okano et al. is composed of cells cultured on a scaffold of temperature-responsive polymers, indicating advancement in scaffold development [6, 7
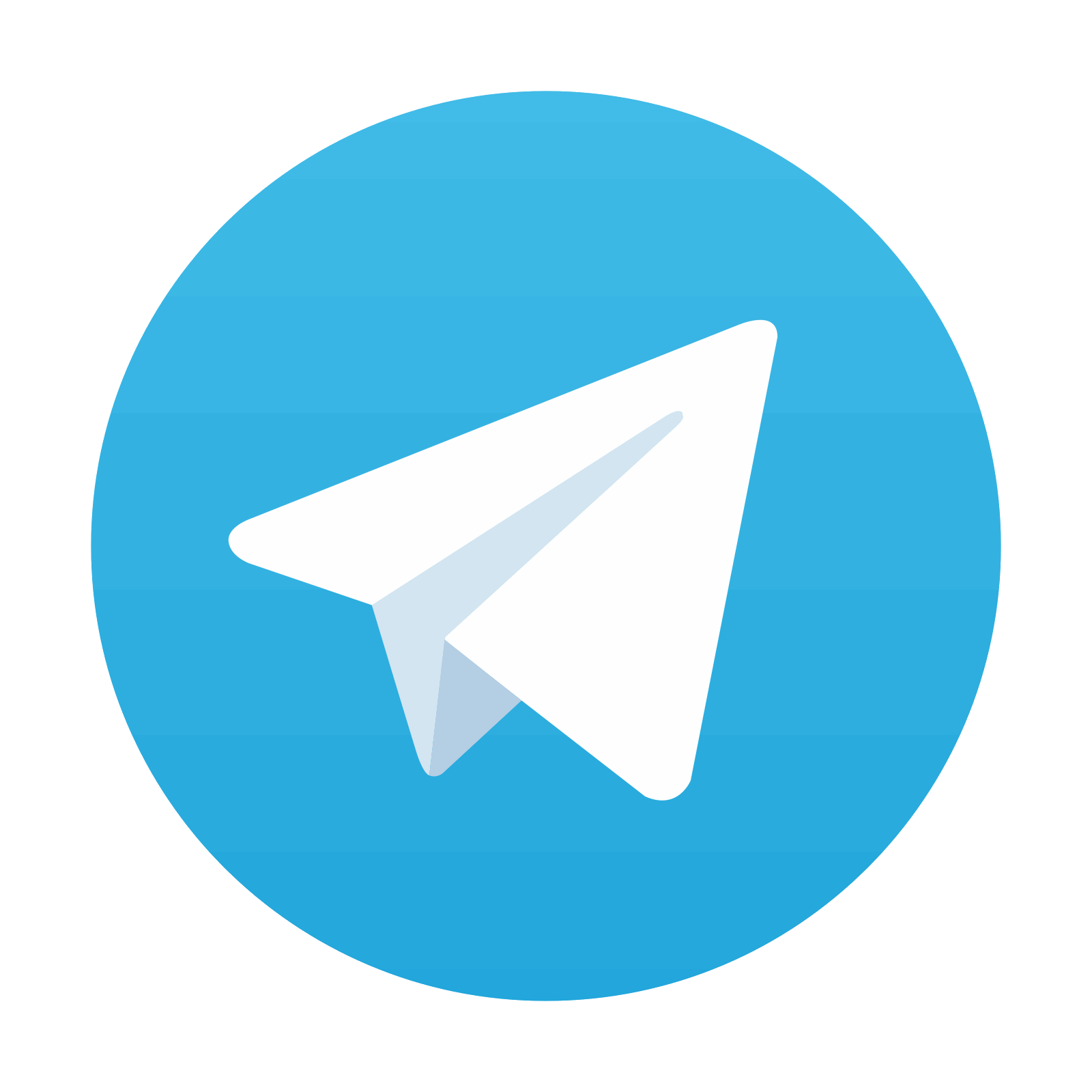
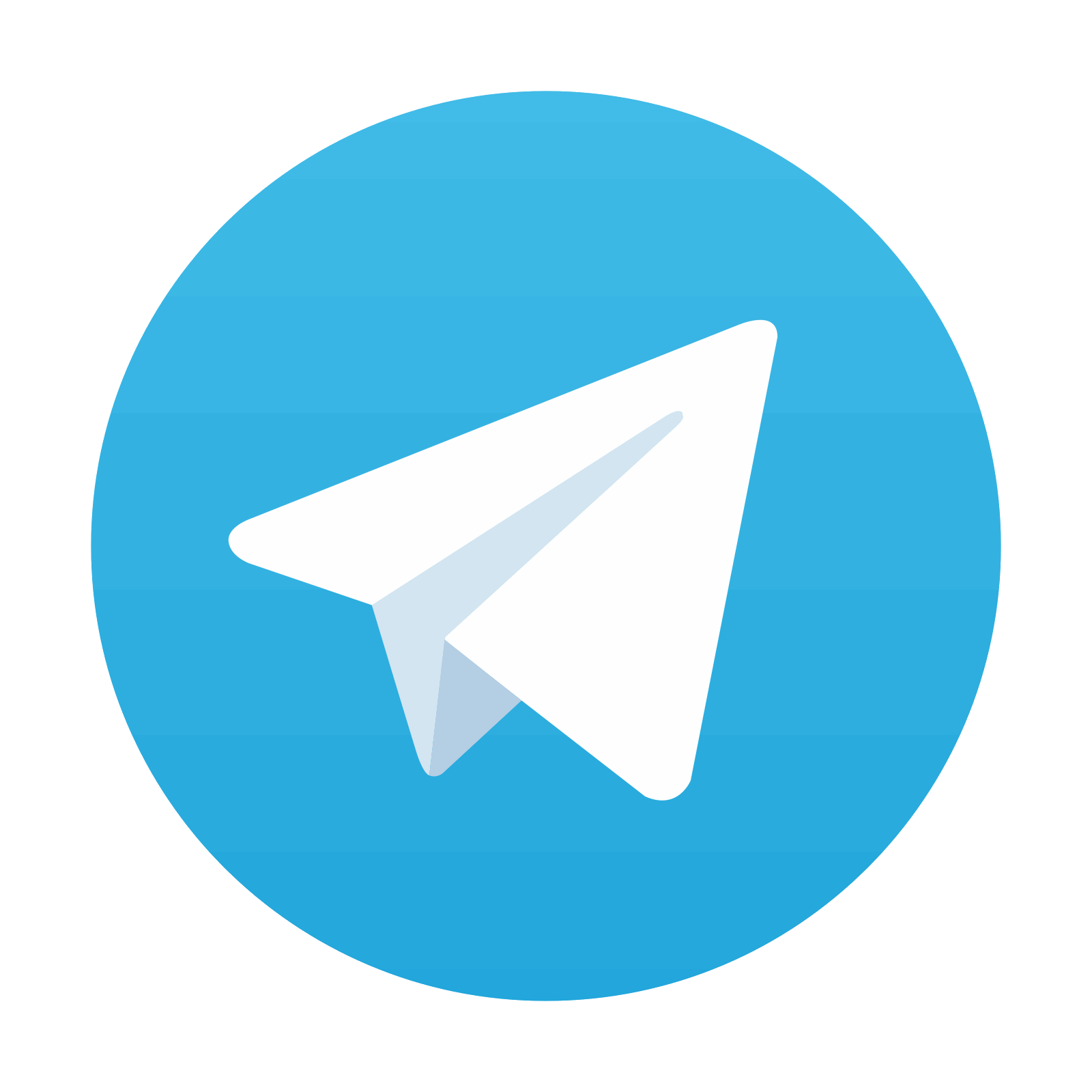
Stay updated, free articles. Join our Telegram channel

Full access? Get Clinical Tree
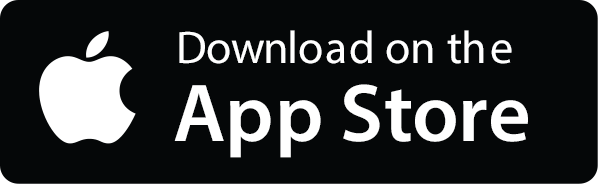
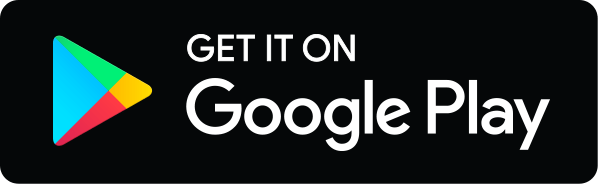