20 Crucial Steps II: Lens Fragmentation
Summary
Nuclear fragmentation and softening offer a new option for assisting with cataract surgery. Multiple studies show that less ultrasound power is utilized in femtosecond laser–assisted cataract surgery compared to manual phacoemulsification, and there is evidence of a modest reduction in endothelial cell loss. This could lead to improvement in patient outcomes and safer surgery, although additional studies are required.
Keywords: lens fragmentation, femtosecond laser, cataract surgery, femtosecond phacoemulsification, corneal surgery, OCT
20.1 Introduction
Femtosecond lasers possess a range of incisional capabilities for corneal surgery to assist in placement of inlays, keratoplasty, and keratomileusis. These lasers also exhibit the ability to cut deeper structures such as the anterior capsule and the crystalline lens to assist with phacoemulsification during cataract surgery. The goals of femtosecond laser–assisted cataract surgery (FLACS) include more precise capsulotomy, reduction in phacoemulsification energy, and ultimately better outcomes compared to manual phacoemulsification.
One key step in FLACS is the fragmentation of the nucleus. Phacoemulsification utilizes ultrasound energy to emulsify and aspirate lens pieces. Higher ultrasound energy can result in damage to surrounding structures. 1 Changes in manual techniques such as chopping may reduce ultrasound energy and preserve endothelial cells. 2, 3 Improvement in phacoemulsification technology and setting also may serve to protect surrounding ocular structures. 4, 5 Femtosecond cataract surgery utilizes the laser to fragment the lens prior to entrance to the eye in order to reduce ultrasound energy and possibly improve safety.
20.2 Intraoperative Imaging of the Lens
Femtosecond laser for cataract surgery utilizes proprietary intraoperative imaging of the structure of the anterior chamber. Live optical coherence tomography (OCT) images of the crystalline lens allow for visualization of important landmarks such as the anterior and posterior capsules. Based on this image, capsulotomy and lens fragmentation patterns are determined by the machine, with the ability of the surgeon to check the image and change the parameters depending on factors such as tilt and image quality.
The surgeon then verifies the treatment and femtosecond laser proceeds with the capsulotomy, allowing the capsule to be cut cleanly without obstruction of laser energy from air bubbles that would be released during nuclear fragmentation. Next, the lens is fragmented in a posterior to anterior direction once again to allow for optimal transmission of laser pulses. Lastly, the cornea incisions may be constructed, including arcuate incisions and wounds.
Each step has safety margins and allows for surgeon verification and adjustments. For the fragmentation in particular, the safety margin from the posterior capsule is essential with alerts to the surgeon if there is concern that pulses might strike the capsule. In addition, surgeon may choose to change where the anterior capsule and posterior capsule margins are planned based on docking, tilt, and movement of the patient. Lasers’ software has a programmable “safety margin” with a typical offset of 500 to 700 µm from the posterior capsule. We find that the 500-μm margin has the advantage of softening much of the epinucleus, so that the epinucleus is largely removed and the surgeon rarely has to deal with a thick epinuclear plate. However newer surgeons may want to utilize a larger safety margin especially with patients who are unable to stay still. As femtosecond technology has improved, fewer adjustments are required from the surgeon during this process.
Limitations to intraoperative imaging of the nucleus include poor dilation, poor view through the cornea, and poor docking. Patient movement may also limit imaging, which can be minimized with light sedation to decrease patient anxiety (▶ Fig. 20.1).
Fig. 20.1 Intraoperative imaging of the nucleus.
20.3 Laser Energy
20.4 Fragmentation Patterns
Segmentation patterns include division of the lens nucleus into quadrants, sextants, or octants. In addition, cylindrical patterns may be constructed based on the device used and surgeon preference. Grid spacing patterns can be made to soften the lens into cubes of the desired size, with vertical cuts combining with pneumodissection of lens layers to create the cube shapes.
A softened lens can lead to reduction of phacoemulsification energy (effective phacoemulsification time [EPT]) required to remove a cataract. Lower EPT often results in improved visual acuity earlier in the postoperative period. 6 Prior studies have showed use of phaco-chop techniques compared to divide-and-conquer techniques results in less ultrasound energy and even reduction in endothelial cell loss. 2, 7
In femtosecond phacoemulsification, studies have shown an absolute decrease in EPT, comparable to reducing lens density to a lower grading level. 8 In a retrospective comparative case series, Mayer and colleagues showed a statistically significant reduction in loss of endothelial cells as well as EPT from 4.17 to 1.58 seconds in FLACS group compared to controls. 9 Abell et al showed a reduction of over 80% EPT in FLACS compared to standard surgery, with complete elimination of phaco power in 30% of the FLACS cases. 10
Matching the fragmentation pattern to the density of the lens may play a role as well. Typically, larger cube sizes (e.g., 750-μm sides) are used for softer lenses and smaller sizes (e.g., 250–200 μm) are used for denser nuclei, depending on surgeon preference and device capability. Obviously, the smaller the cubes, the more energy put into the lens, resulting in longer treatments and generation of more air bubbles. Comparing 350- to 500-μm-fragmentation grids made with the AMO Catalys system, Conrad-Hengerer et al showed that the smaller grid size resulted in lower EPT in moderate and advanced cataracts. 11 Other studies have shown reduction in endothelial cell count using combinations of cross-sections and cylinders. 9 Newer devices and software can determine the density of the lens during intraoperative OCT imaging and determine the optimal grid pattern or fragmentation pattern (▶ Fig. 20.2).
Fig. 20.2 Fragmentation patterns.
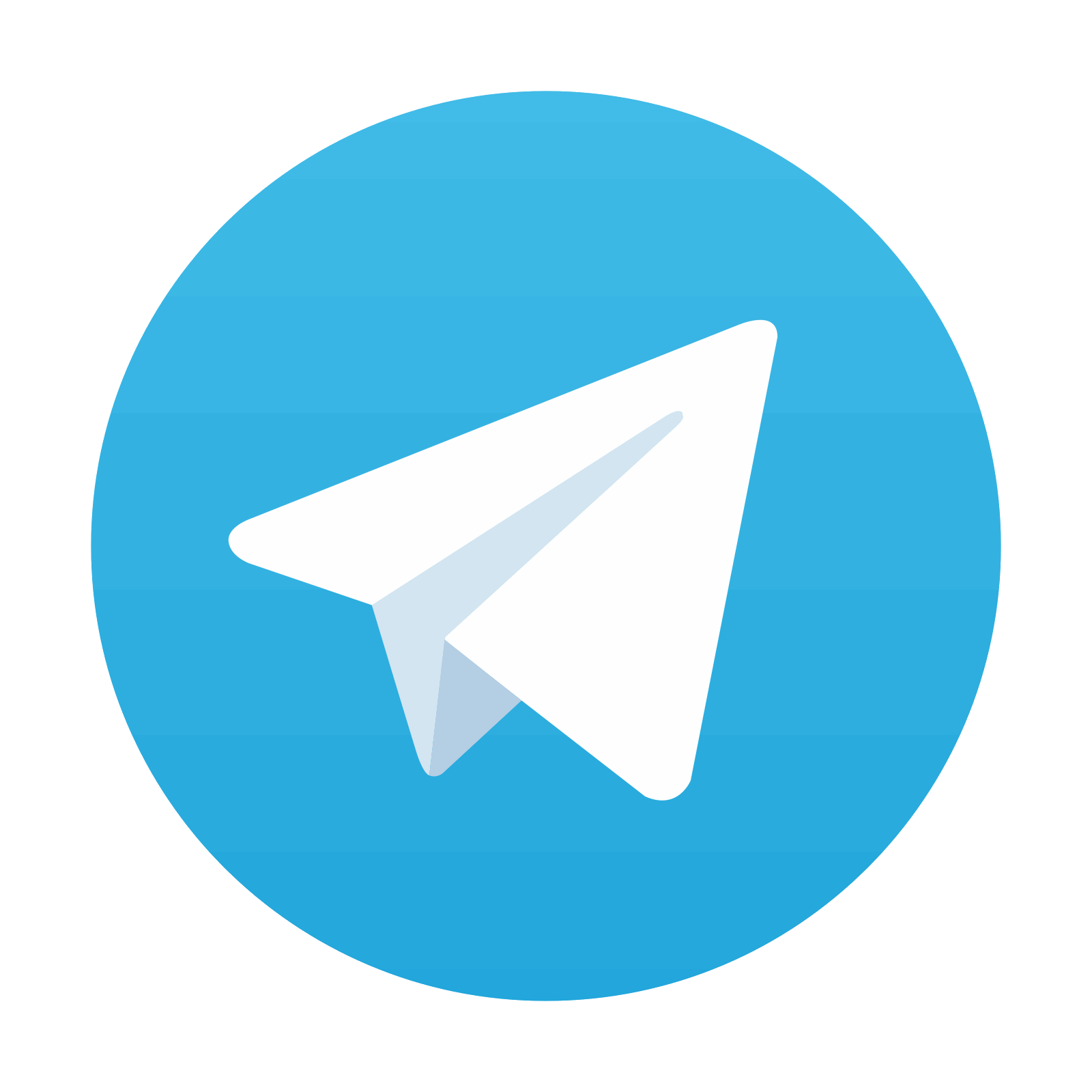
Stay updated, free articles. Join our Telegram channel

Full access? Get Clinical Tree
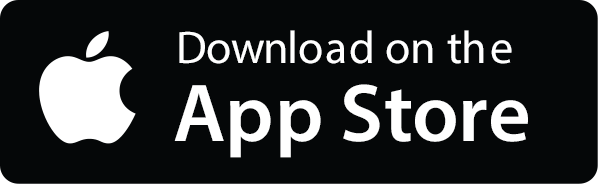
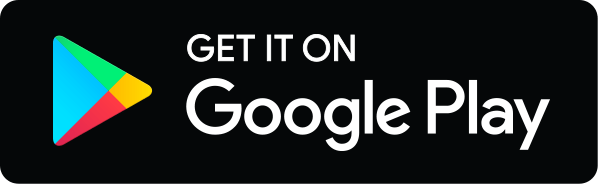