Fig. 3.1
Schematic representation of the anatomy of corneal nerves. Nerve fibers enter the cornea at the limbal region and then run radially toward the center as stromal fibers. These fibers tend to reach the stromal superficial layers and perforate the Bowman’s layer at different topographical locations (different colors). The sites of Bowman’s membrane perforation are depicted by colored dots. After gaining the superficial location, they give origin to the subbasal fibers
It is of interest to consider that when surface refractive ablations (i.e., PRK) are performed, the epithelial removal and the superficial ablation remove the central Bowman’s layer and anterior stroma, therefore eliminating the epithelial, subbasal, and most anterior stromal nerve fibers. In case of LASIK surgery, although the epithelium, Bowman’s zone, and very anterior stroma are preserved from ablation in the flap, the nerve fibers are resected peripherally due to the flap circumferential side cut, while deeper stromal fibers are damaged by the ablation under the flap. When a femtosecond refractive lenticule is dissected and removed, in the SMILE technique, stromal nerve fibers within the lenticule are resected, but the absence of surface damage and of the flap creation represent the anatomical basis for a different pattern of corneal nerve damage that will be discussed further in this chapter.
3.2.2 Stromal Keratocytes
Corneal keratocytes (corneal fibroblasts) are specialized fibroblast cells residing in the stroma. These cells are interspersed within the structure of the stroma that is built up from highly regular orthogonally arranged collagenous lamellae and extracellular matrix components. Keratocytes play a key role in maintaining the stromal structure and transparency of the cornea, being the source of stromal collagen and proteoglycans. They also mediate corneal wound healing and tissue repair and are capable of undergoing phenotypic transformations in wounds due to the influence of growth factors and cytokines. These specialized cells are therefore involved in healing corneal wounds and synthesizing its components. In the unperturbed cornea, keratocytes are normally quiescent, but they can rapidly respond and change into repair phenotypes following injury [14, 15]. Stromal keratocyte density has been evaluated both ex vivo and in vivo, and it is known to be higher in the anterior third of the stroma, while it progressively decreases in the mid and rear stroma (Fig. 3.2).


Fig. 3.2
Schematic representation illustrating the keratocyte distribution within the corneal stroma and the surgical anatomy of three different corneal refractive surgical techniques: PRK, LASIK, and SMILE (section)
In excimer laser surface ablation techniques, the very anterior keratocytes (depending on the ablation depth) are damaged, and apoptosis mechanisms, with subsequent wound healing cascade, are triggered in the most keratocyte-populated area of the stroma. In LASIK technique the keratocyte and stromal healing phenomena occur at a deeper level (in the residual stromal bed under the flap-stroma and the ablated tissue), where the keratocyte density is lower. In SMILE and to some extent in FLEx techniques, the unique use of femtosecond laser dissection with no photoablation induces a different pattern, of keratocyte changes and wound healing (in the anterior-mid stroma), characterized by less apoptosis and inflammation, as already discussed in the previous chapter.
3.2.3 In Vivo Confocal Microscopy of the Cornea: An Imaging Technique for Nerves and Keratocytes
In vivo confocal microscopy (IVCM) has gained a notable popularity and consensus as a powerful imaging tool among cornea specialists in the last two decades. It is a noninvasive diagnostic imaging system that allows viewing of tissues, in particular the cornea at its microscopic details, one depth level at a time [16–19]. This kind of microscopes is designed for noninvasive, real-time, in vivo examination of tissues without the need of stains or dyes but does require operator-dependent expertise and skill in optimizing the quality of the obtained images and their interpretation. Currently two different confocal technologies are available for clinical use: the laser scanning and the white-light scanning confocal microscopy. These two confocal systems clearly differ in the capacity of ocular tissue imaging depending on the type of light source used. White-light confocal microscopy offers a detailed view of the corneal layers, provides an optical pachymetry of the entire cornea or of selected sublayers, and allows for automatic cell counts (i.e., keratocytes and endothelial cell density), but the imaging quality is affected by tissue transparency and stromal edema more than laser scanning confocal systems. Due to the fact that the cornea is generally a nearly transparent tissue, with minimal light absorption, the increase in axial resolution allows confocal microscopy to scan optical sections of the entire corneal thickness producing high magnification (between 600 and 1,000×) “en face images” of the cornea (maintaining lateral and axial resolution within values comprised between 1 and 3 μm and 5 up to 10 μm, respectively). Almost all anatomical corneal layers are clearly imaged by confocal microscopy: superficial epithelium, basal epithelium, Bowman’s membrane, subepithelial nerve plexus, corneal stroma with resident keratocytes, deep nerve fibers, Descemet’s membrane, and endothelium. Figure 3.3 presents a composition of images illustrating confocal microscopic anatomy of the central human corneal layers. As visible in the figure, in vivo confocal images are oriented parallel to the microscope objective and to the corneal surface, differently than histological specimens, which are sectioned perpendicular to the surface of the cornea, and thus the observer has to become familiar with the imaging in the coronal plane.


Fig. 3.3
Composition of images illustrating confocal microscopic anatomy of the central human corneal layers, with emphasis on visualizing nerve fibers and keratocytes. (a) Basal epithelium. (b, c) Different pattern of subepithelial nerve plexus. (d) High density of anterior stromal keratocytes. (e) Mid-stromal keratocytes present a lower density. (f) Normal endothelial cell mosaic
3.3 Nerve Fibers Change After ReLEx Refractive Surgery: Morphology and Clinical Meaning
3.3.1 Nerve Fibers and Tear Film Alterations After Excimer Laser Surgery
Any kind of subtractive laser refractive surgery has the disadvantages of inducing a variable degree of damage to the corneal nerve fibers. It is well known that the integrity of nerve fibers is fundamental for the physiological maintenance of the ocular surface tear film dynamics and corneal epithelial homeostasis. Tear secretion is regulated through a neural reflex initiated by trigeminal primary afferent neurons innervating the corneal epithelium. Dry eye phenomena may be induced by a dysfunction in the tear-secreting glands or in the neuronal circuit regulating these glands [20].
Patients’ complain of dry eye symptoms and related epitheliopathy commonly occur after traditional refractive surgery techniques. The most frequently reported manifestations are ocular dryness that takes place in almost 40 % of LASIK (laser-assisted in situ keratomileusis) and PRK (photorefractive keratectomy) treated eyes, but also nonspecific ocular surface discomfort associated with mild corneal epitheliopathy and reduced lubrication may occur [21]. At the present time LASIK (either performed with microkeratomes or with Fs-Laser) is considered as one of the gold standard refractive procedures, supported by numerous investigations, for its visual outcomes and rapid postoperative recovery. The LASIK technique induces morphological and functional effects on the corneal surface. Changes in corneal shape, tear film dynamic, and subepithelial innervation after surgery exert a significant influence in ocular discomfort syndrome onset. The impact of these factors produces detrimental effects to visual results including fluctuation of vision quality and decrease of contrast sensitivity and, in some cases, of best spectacle-corrected visual acuity [22]. One of the key points to consider in the occurrence of these phenomena is the fact that different patients may present a variable spectrum of symptoms to identical surgical stimuli and that preexisting dry eye before surgery can be a risk factor for severe ocular discomfort after refractive surgery.
As explained, excimer laser-based procedures (either LASIK or surface ablation techniques such as PRK) result in a sudden central corneal nerve fiber damage related to the flap cut, in LASIK, and to the excimer photoablation of the anterior stroma containing nerve fibers, that takes time to recover, often only partially. The wound healing of central corneal subepithelial nerve fibers has been reported to be only slightly different between LASIK and similar flap-based procedures, regarding the time-to-recovery and the morphological appearance of the regenerated nerve plexus. At 1 month after surgery, the central cornea appears devoid of nerve fibers in conventional LASIK procedure, sub-Bowman’s keratomileusis (SBK), and femtosecond laser-assisted LASIK (Fs-LASIK) [23]. The process of reinnervation begins in the early period after surgery in the peripheral flap region with the formation of thin non-branching fibers crossing the side cut. Nerve morphology changes rapidly across the following months reaching the central 3 mm area at 6 month [23]. In spite of this nerve regrowth, the density and the nerve morphology remain altered for years after surgery, representing the basis for some clinical conditions of dryness and recurrent superficial epithelial punctate erosions. Surface excimer laser ablations showed a faster recovery time, with 2 year of mean time-to-restoration against 5 year of the flap-based procedures [24].
Along with the corneal neural impairment, patients show an altered corneal sensitivity that follows a similar pattern by decreasing significantly immediately after, persisting reduced in the first 3 months, and returning to normal values at 6 months after surgery [25]. Previous investigations reported a persistent noncomplete normalization at 1 year and more [26].
The risk of developing “dry eye syndrome” appears to be correlated with the degree of preoperative myopia and the depth of laser ablation/stromal dissection; moreover, in dry eye-affected patients, the risk of myopic regression is increased [27, 28]. The status of the ocular surface and tear film before and after refractive surgery can adversely affect the outcomes in terms of complications, refractive results, optical quality, patient satisfaction, and the severity and duration of dry eye after LASIK [29].
Recently femtosecond laser systems became available for the clinical use in corneal refractive surgery and favored improvements in the reliability of LASIK procedures by increasing flap precision (thickness, shape, diameter), setting up planar configuration of the flap cut geometry, and giving more predictable depth of dissection and reduction of intraoperative complications incidence [30]. Despite these advantages with Fs-laser-created flaps, corneal nerve plexus presents the above-described modification and remains affected by severe damage for a long time. In fact, the flap creation implies the transection of all nerve fibers at the lamellar border. In spite of the better alignment of flap margins achieved with Fs-LASIK, the reinnervation process emerges to be similar to the well-known microkeratome-LASIK procedures. Central nerve fibers density reduction and slow progressive recovery turned out to be comparable in both techniques as long as 3 years after surgery [30]. Similarly, the incidence of dry eye symptoms after surgery is similar in both procedures. Therefore, the planar configuration of thin Fs-LASIK flaps is not associated with faster reinnervation compared with the microkeratome flaps and does not provide clinically significant advantages in terms of induced neurotrophic epitheliopathy. Patients complaining of post-refractive surgery dry eye symptoms are generally treated by frequent instillations of lubricant eye drops and occasionally local steroids and/or topical cyclosporin A for weeks to months after surgery; however, there is no recognized and definite treatment for such condition.
3.3.2 Corneal Nerve Fibers After ReLEx: Anatomical Bases and Scientific Evidence
Femtosecond laser refractive surgery received a notable advancement with the introduction of “all-femto” SMILE technique. The capacity of the femtosecond laser of designing intrastromal dissection planes with three-dimensional cut complex geometry helps the surgeons to remove corneal tissue without a flap by creating a lenticule and a small incision to extract it. The first dissection plane designs the backside face of the lenticule, while the second one creates its anterior face and extends further to form a spaced intrastromal pocket to facilitate the extraction maneuvers (edge of the so-called cap). The complete procedure happens without affecting the superficial tissue, fashioning an intrastromal “disk” that can be easily extracted through the 40–50 arc degrees single superficial incision.
The basis that explains the pattern of nerve fiber resection induced by SMILE (similar to other refractive surgical procedure) relies in the anatomy of the intracorneal distribution of the fibers that give origin to the subbasal nerve plexus and of the topography of emerging fibers that penetrate the Bowman’s layer (BL) running, thereafter, superficially. As explained previously in this chapter, the stromal nerves, coming from the suprachoroidal space through the limbal region, course toward the center in a radial manner and penetrate the Bowman’s layer at different locations, mostly situated in the mid-peripheral zone (outside the central 6 mm), creating, thereafter, the subbasal plexus. A schematic representation illustrating the nerve fiber pathway that runs centripetally is presented in Figs. 3.1 and 3.4a. When a flap-based technique (LASIK, Fs-LASIK, or FLEx) is performed, all nerve fibers that are running across the flap side-cut circumference are resected (the majority of the central and paracentral stromal fibers). The excimer laser ablation on the stromal bed further vaporizes remaining deeper stromal fibers. Only the fibers that run in the region of the flap hinge (few fibers) may course undisturbed within the lifted flap as subbasal fibers (see Fig. 3.4b). This supposed mechanism is in agreement with the described clinical and confocal observations that reported a remarkable reduction of corneal sensitivity and of the central subbasal corneal nerve plexus after LASIK.


Fig. 3.4
(a) Stromal nerve fiber bundles run centripetally and toward the surface perforating the Bowman’s layer (spots indicated by yellow circles). Once the fibers penetrate the BL, the subbasal nerve plexus is originated. Different colors illustrate the subbasal fibers originating from central (red), paracentral (light blue), and peripheral (green) perforating stromal fibers, respectively. (b) In the LASIK procedure, all fibers are cut throughout the extension of the 300–310° degree of arc flap side cut; moreover all deeper fibers are disrupted within the photoablation area. (c) With the SMILE procedure, in the absence of a full flap side cut, peripheral nerve fibers are resected only were the 50° arc of the incision is placed (thick red line). Moreover fibers are resected if rising superficially to perforate the BL within the area of the created and extracted refractive lenticule. The other fibers that had penetrated the BL outside the lenticule area may run undisturbed as subbasal nerve plexus (for simplicity, central subbasal surviving fibers are not depicted in the central zone)
On the other hand, when a SMILE procedure is performed, it is presumed that a different pattern of nerve resection occurs:
1.
A certain amount of fibers running centripetally in the superficial stroma and in the subepithelial zone are resected due to the side cut where the incision is placed.
2.
Other stromal fibers that run toward the surface perforating the Bowman’s zone inside the refractive lenticule and cap area might be interrupted by the lenticule/cap planar dissection itself.
3.
Conversely, fibers that gained the superficial subepithelial location after perforating the BL in areas located outside the lenticule/cap area may run untouched (inside the stromal and epithelial component of the cap) over the refractive zone without interruption with the exception of the mentioned peripheral incision (see Fig. 3.4c).
Some recent investigations have evaluated the corneal innervation and corneal sensitivity after ReLEx surgery. Authors that investigated changes in corneal sensitivity reported it to be significantly better after SMILE than after LASIK in every corneal area. Interestingly, corneal sensitivity values were found to reach preoperative levels as soon as 3 months after SMILE surgery, therefore suggesting that long-lasting changes, typical after LASIK procedures, should not occur after flap-less ReLEx SMILE surgery [31]. Vestergaard et al. evaluated corneal sensitivity and used confocal microscopy to study the subbasal nerve plexus morphology comparing two groups of femtosecond laser refractive surgery treated patients: the SMILE procedure as compared to the FLEx technique (the original approach to all-femto refractive myopic lenticule extraction, based on a complete flap lifting) [32]. The authors observed a better corneal sensitivity and a significantly higher density of central corneal nerve fibers as observed by confocal microscopy in the SMILE group with respect to the FLEx group at 6 months follow-up time. This study highlighted the importance of a flap-less procedure to favor a rapid nerve restoration; however, it does not take into account the early postoperative phase, and the LASIK technique was not investigated. Another investigation used in vivo confocal microscopy to evaluate changes of corneal innervation following SMILE surgery as compared to Fs-LASIK-treated eyes [33]. It reported a decrease in subbasal nerve fiber density less severe after SMILE in comparison to Fs-LASIK in the early postoperative phase (1 week to 3 months), while nerve density reached similar values at 6 months following both procedures. Nerve density values were found to correlate well with corneal aesthesiometry.
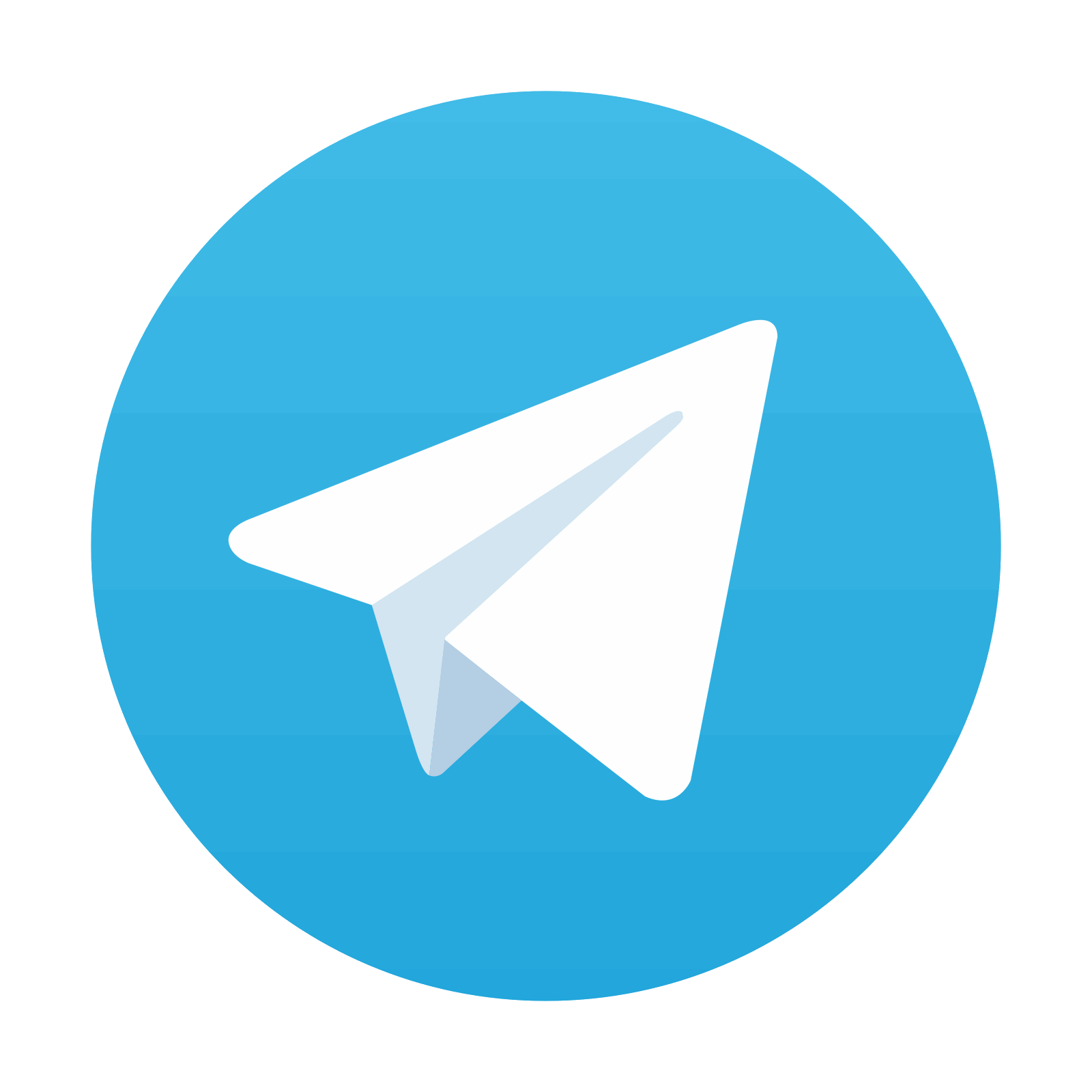
Stay updated, free articles. Join our Telegram channel

Full access? Get Clinical Tree
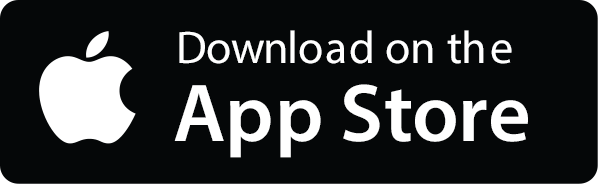
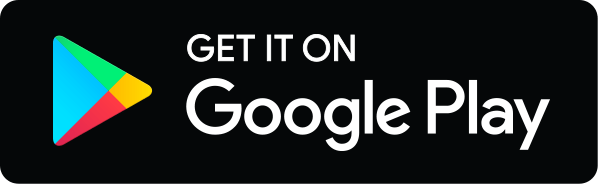