Fig. 8.1
Flowchart to explain the changes of the cornea with age
It has already been demonstrated that the corneal stiffness increases by a factor of 2 between the ages 20–100 [16], and the stress and strain relationship changes result in a determination of elasticity defined as Young’s modulus. KC may influence the development of natural stiffness within the cornea allowing the change in elasticity to assume a behavior similar to that of plastic, whereby the original shape is not regained following external forces or even forces which are usually resisted by a “normal” cornea. Of course, it would be unreasonable to regard corneal biomechanics so simply, but this would allow us to understand why diabetics are less likely to develop severe KC [19, 20].
It is of course important to mention that there are children who may be wrongly diagnosed with KC based on clinical findings. Without the availability of ultra-modern diagnostic techniques in all units, and with the issue of patient compliance with many of these tests, it is important to remember the differential diagnoses when suspecting KC [21]. There have been cases of forceps obstetric deliveries causing corneal changes mistaken for KC, as the break in Descemet’s membrane (DM) can cause acute hydrops, which can occur in KC. Forceps corneal injuries have been documented as far back as 1895 [22]. Similarly, a clear history should be elicited and any history or suggestion of trauma should be considered as a potential cause of DM breaks. Conditions such as keratoglobus (KG) and congenital glaucoma could also potentially present with similar findings, and so a full ocular and systemic examination is necessary, not forgetting to document corneal measurements, axial lengths, intraocular pressure (IOP), and fundal findings including optic nerve appearances.
Presentation Age of Keratoconus
The age range of KC is typically adolescence through to early adulthood, as this may represent a period when the cornea is most biomechanically susceptible. There are situations when the condition presents unusually early or late, and in these cases there may be excessive external factors or preexisting abnormalities in the corneal biomechanics. The youngest case of KC in the literature was a 4-year-old girl with Down syndrome, who developed KC and underwent CXL [23]. This patient was reported to be stable with regard to her KC until the report was published when she was 7 years old. Similar case reports of young children with KC have been published thought to be due to persistent eye rubbing [24, 25]. The authors have treated progressive KC in 4- and 6-year-old siblings. Some corneas may be more at risk than others of developing KC at an earlier age if triggered by environmental factors, which may be related to inflammation. The improved detection techniques such as epithelial analysis may allow for treatment of subclinical KC in children at a very young age, preventing any manifestation of the disease.
What Triggers Keratoconus in Childhood?
A currently unanswered question would be whether KC presents in childhood because it is an aggressive form, or whether the child’s corneas allow for KC to present earlier and maybe more rapidly? It is important to distinguish how and why KC presents earlier in some patients during childhood, and whether this is a variant or simply an earlier presentation [26]. It is all too easy to assume that if CXL is effective in increasing the rigidity of the cornea to reduce or halt the progression of KC, the same would apply to a child’s cornea. However, is this based on anecdotal evidence or can we confirm that a child’s cornea would respond in the same way as an adult? We must bear in mind that treatment for KC prior to the advent of CXL would have been supportive with as much visual rehabilitation as possible until a corneal graft would be necessary. Should we therefore embrace CXL as a requirement when possible to prevent any progression whatsoever?
If a child presents with rapidly progressing KC, this may indicate that there has been a failure, or lack of, formation of natural cross-links between the collagen fibers within the cornea via processes such as transglutaminase and lysyl oxidase enzyme pathways [27]. Therefore, the cornea allows for KC to develop due to genetic make-up/missing vital components to maintain CH and resistance. This may be associated with an underlying systemic or ocular condition leading to the development of early KC. It would therefore be important, especially in a child, to consider systemic associations such as those highlighted in Table 8.1, which is adapted from Rabinowitz et al. [2]. It is important to remember that associations such as Tourette’s syndrome relate to the behavioral aspects related to the condition rather than the condition directly causing KC, such as recurrent eye rubbing or trauma [28].
Table 8.1
Diseases associated with KC
Systemic associations | Ocular associations |
---|---|
Alagille’s syndrome | Aniridia |
Albers-Schonberg syndrome | Anetoderma and bilateral subcapsular cataracts |
Angelman syndrome | Ankyloblepharon |
Apert’s syndrome | Bilateral macular coloboma |
Autographism | Blue sclerae |
Anetoderma | Congenital cataracts |
Bardet-Biedl syndrome | Ectodermal and mesodermal anomalies |
Crouzon syndrome | Floppy eyelid syndrome |
Down syndrome | Gyrate atrophy |
Ehlers-Danlos syndrome | Iridoschisis |
Goltz-Gorlin syndrome | Leber congenital amaurosis |
Hyperornithemia | Persistent pupillary membrane |
Ichthyosis | Posterior lenticonus |
Kurz syndrome | Retinitis pigmentosa |
Laurence-Moon-Bardet-Biedl syndrome | Retinal disinsertion syndrome |
Marfan’s syndrome | Retrolental fibroplasia |
Mulvihill-Smith syndrome | Vernal keratoconjunctivitis |
Nail patella syndrome | Atopic keratoconjunctivitis |
Neurocutaneous angiomatosis | Axenfeld’s anomaly |
Neurofibromatosis | Avellino’s dystrophy |
Noonan’s syndrome | Chandler’s syndrome |
Osteogenesis imperfecta | Corneal amyloidosis |
Oculodentodigital syndrome | Deep filiform corneal dystrophy |
Pseudoxanthoma elasticum | Essential iris dystrophy |
Rieger’s syndrome | Fleck corneal dystrophy |
Rothmund’s syndrome | Fuch’s corneal dystrophy |
Tourette’s disease | Iridocorneal dysgenesis |
Turner’s syndrome | Lattice dystrophy |
Xeroderma pigmentosum | Microcornea |
Congenital hip dysplasia | Pellucid marginal degeneration |
False chordae tendineae left ventricle | Posterior polymorphous dystrophy |
Joint hypermobility | Terrien’s marginal degeneration |
Mitral valve prolapse | |
Measles retinopathy | |
Ocular hypertension | |
Thalesselis syndrome |
The other factor to consider would be environmental. This may include where the child was brought up (hot vs. warm climate), ethnic background, presence of ocular inflammation (VKC/ atopy), or chronic eye rubbing. Eye rubbing is an interesting association, as several hypotheses have been posed as to why and whether a direct association exists [24, 28, 29]. The youngest reported case of KC was found in a 4-year-old girl known to rub her eyes habitually [23].
There may also simply be a more aggressive form of KC presenting earlier in life, and this may explain the earlier and more advanced stages at presentation. The Collaborative Longitudinal Evaluation of Keratoconus (CLEK) study lasted 8 years and was a multicenter natural history study of 1209 patients with a minimum age of 12 [26]. The study established that age is a major factor in severity-related outcomes of KC; the earlier the presentation of clinical symptoms/signs, the more severe the KC will become. This association would definitely warrant further studies to confirm, even though it has been supported by many case reports and observational studies [5, 27, 30–37]. Similarly, the CLEK study found that half of the patients reported rubbing one or more eyes vigorously [26]. As it has been established that eye rubbing/atopic eye disease is associated with KC progression, this is one modifiable factor that can be controlled. While not specifically looking at children, the study did mention younger age as a risk factor for corneal scarring.
Diagnostic Techniques in Childhood Keratoconus
Corneal Tomography vs. Clinical Evaluation
As mentioned in Chaps. 1 and 2, the keystone in diagnosis of KC is corneal tomography. However, doing corneal tomography to a child is challenging, and sometimes it can be difficult, unreliable, or even impossible. Therefore, clinical examination with the use of retinoscopic patterns, changing refraction, and corneal signs remain the mainstay diagnostic methods in young and/or noncompliant children.
Anterior Segment Optical Coherence Tomography (AS-OCT) and Epithelial Imaging
Using Fourier-domain AS-OCT, epithelial maps can provide subtle differences within and between eyes. The use of epithelial thickness in KC has been studied, and the markers of KC may be reflected in epithelial thickness patterns. An increased thickness variability of the epithelial layer is found in KC with a thin layer over the cone. In contrast to normal corneas, the KC corneas have thicker epithelium superiorly compared to inferiorly [4]. Concerns include the influence of VKC and eye rubbing on the epithelium, as the epithelial layer does fluctuate with severity of disease. The idea of epithelial thickness variability suggests that the epithelium may be adapting to the irregularities developing early on the keratoconic stroma. Topographers may miss early KC due to the lack of epithelial analysis. As a result, by marking out these patterns through epithelial layer analysis, the variability and early warning markers may make earlier diagnosis of KC possible. This would be an ideal method of detection in children, but there may be a couple of drawbacks. The most obvious would be the influence of eye rubbing and VKC disease on measurements. The next concern would be whether everybody with epithelial variability and thickness changes have early KC? There may be many who have very mild, previously undetected signs of KC who never progressed, including those labeled as “forme fruste” that may never require an intervention. Of course, detection would be useful in screening for refractive laser surgery, but would subjecting these groups to either frequent screening or early CXL be warranted? How could we be sure all these detected eyes would progress into KC if left alone?
Corneal Cross-linking: Why Children?
Before the seminal paper by Spoerl and Seiler [20, 39, 40] suggesting that ectatic disease such as KC may benefit from CXL, CXL was developed for lens material following phacoemulsification [41] and epikeratoplasty (a historical operation used to flatten the corneal surface) [42]. Currently riboflavin was found to be a suitable photosensitizer as it was nontoxic, water-soluble and penetrated the corneal stroma easily without epithelium on the cornea [39, 43].
CXL stiffens the cornea by 328 % [20], increasing Young’s modulus by 4.5, and so increasing rigidity preventing development of an increasingly misshapen cornea. In a cornea where the elasticity is not supportive enough to return it to a normal shape in the presence of pressure, CXL would allow rigidity to prevent the stretching of tissue.
Figure 8.2 demonstrates the stress–strain relationship. In this figure, the different colored lines represent more elastic and more brittle materials. The cornea may behave similar to the black line, but of course corneal biomechanics is a far more complex entity with the cornea having viscoelastic properties.


Fig. 8.2
Stress–strain relationships of different corneas. The black line represents a normal cornea
Current Evidence for Corneal Cross-linking in Children
With no adequate prospective randomized control trials (RCT) in adult CXL, there is even less evidence for CXL in children. A recent Cochrane review [14] looked at three RCTs, analyzing 219 eyes. Due to different performance and outcome measures, it was not possible to pool the data that only included adults, and even to look at the 12-month outcome data there was insufficient evidence and performance, detection, and attrition bias. It is, however, generally regarded that CXL does halt the progression of KC despite the lack of RCT evidence. Four-hundred and sixty-two papers were reviewed for the article, and the vast majority supported the use of CXL, in particular Epi-off, and even though this evidence would not be the strongest, the overwhelming positive reports cannot be ignored.
While there have been case reports and studies with a limited follow-up time showing success, the actual evidence base for CXL in children is lacking. Initially, CXL was advised to be limited to those aged 18 or over, but it is clear that the ectatic process begins earlier than this age [2], and as a result, Sorters et al. suggested the use of CXL in children who showed progression [44]. Arora et al. [34] established by their review of 15 patients that CXL was safe and had good visual and topographic outcomes at 12 months. Similarly Chatzis et al. [32], with a longer follow-up time and increased patient number (average 3 years and 59 eyes, respectively), were more conclusively able to ascertain that while CXL was effective in halting progression, this effect was lost in 55 % patients by 36 months (increase in Kmax by > 1 D). More importantly, during the study period, Chatzis was able to confirm that 88 % of patients progressed, suggesting that with such a high progression rate in these patients who have early KC, treatment should not be withheld until progression has been documented. Kankariya et al. [30] also specifically reviewed pediatric KC management, and this review has suggested that the current evidence would only advise what has already been the protocol for adult patients. This would include the use of 400-μm depth as a minimum for isotonic riboflavin. Interestingly no treatments have been as effective as the Dresden protocol of Epi-off technique.
Summary of Current Evidence Available for CXL in Children
Table 8.2 is a summary of the available studies looking at CXL in children and their findings. The youngest child treated with CXL in the reviewed literature was 8 years old [46]. However, there have been case reports of younger patients having CXL [23, 24].
Table 8.2
A summary of all the papers available on CXL in children at time of publication
Study | Subjects (eyes) | Age range | Design | Evidenced progression | CXL method | Outcomes | Duration F/U | Complications |
---|---|---|---|---|---|---|---|---|
Soeters et al. 2011 [45] | 4 (5) | 10–16 | Case series | Yes | Dresden | 4 stabilized, 1 PKP | 1 year | Nil |
Arora et al. 2012 [34] | 15 (15) | 10–15 | Prospective | No | Dresden | Stabilized | 1 year | Nil |
Bakshi et al. 2012 [35] | 9 (9) | 11–17 | Retrospective | Yes | Dresden | Stabilized | 2 years | Nil |
Vinciguerra et al. 2012 [33] | 40 (40) | 9–18 | Prospective | Yes | Dresden | Stabilized | 2 years | Nil |
Caporossi et al. 2012 [36] | 77 (152) | 10–18 | Prospective | Yes | Dresden | Stabilized | 3 years | Nil |
Chatzis et al. 2012 [32] | 52 (59) | 9–19 | Retrospective | Yes | Dresden | Stabilized, but progressed later | 3 years | Nil, 88 % progression |
Maghli et al. 2013 [46] | 29 (37) | 12–18 | Retrospective | Yes | Epi-on and Epi-off | Stabilized | 1 years | Prolonged postop edema in Epi-Off group |
Buzzonetti et al. 2012 [44] | 13 (13) | 8–18 | Prospective | No | Epi-on CXL | Progression | 18 months | Nil, demarcation 105 μm |
Zotta et al. 2012 [31] | 4 (8) | 11–16 | Case series | Yes | Dresden | Stabilized | 3 years | Nil |
Shetty et al. 2014 [47] | 18 (30) | 11–14 | Prospective | Yes | Accelerated CXL | 3 VKC eyes progressed (17 %), rest stabilized | 2 years | 17 eyes (10 patients) had VKC |
Salman et al. 2013 [48] | 22 (22) | 13–18 | Prospective | No | Epi-On CXL | Stabilized | 1 year | Nil |
Ozgurhan et al. 2014 [49] | 38 (44) | 9–18 | Retrospective | Yes | Accelerated CXL | Stabilized | 2 years | Nil |
Buzzonetti et al. 2015 [50] | 14 (14) | 10–18 | Case series | No | Iontophoresis Epi-On CXL | Stabilized | 15 months | Nil, good tolerance to treatment |
Bakshi et al. 2014 [103] | 21 (31) | 11–17 | Retrospective | Yes | Dresden | Stabilized | 3–48 months (80 % 1 year) | Nil |
Barbara et al. 2012 [27] | 20 (29) | 12–18 | Retrospective | Yes | Epi-off and Disruption | Stabilized | 6–48 months (mean 25) | 1 postop keratitis – resolved |
Summary | 376 (508) | 8–18 | 6 prospective 9 retrospective | 8 Dresden (2 with accelerated CXL) | Majority stabilized | 1–4 years |
Methods of CXL Treatment and the Corresponding Evidence
Epithelium-Off Technique
The original Dresden protocol stated the importance of removing the epithelium to allow riboflavin to penetrate the cornea. This technique remains the “gold standard” for CXL currently [5, 40]. Eight out of 13 papers investigating CXL in children adopted the Dresden protocol with a further two studies using accelerated CXL with Epi-Off. Therefore, the overwhelming majority of evidence is based on Epi-Off CXL. Table 8.3 summarizes the steps of the original Dresden protocol.
Table 8.3
The original Dresden protocol, still in use by many units today
Dresden protocol |
---|
Topical anesthetic (tetracaine); can also apply pilocarpine 2 % |
Remove central 7–9 mm epithelium (with hockey stick/spatula) |
0.1 % riboflavin 5-phosphate with 20 % dextran every 5 min for 30 min |
UVA light (370 nm, 3 mW/cm2) 30 min while continuing to apply riboflavin every 5 min |
Topical antibiotics, bandage contact lens |
Epithelium-On Technique
It would be ideal, particularly in children, to avoid deepithelialization for CXL. There are obvious reasons for this such as less pain, more tolerance to the procedure itself, and less risk of infection and possible inflammation. The molecular weight of riboflavin prevents effective absorption on its own through healthy epithelium [10, 11]. Pinelli et al. have created protocols for Epi-On treatment, via pretreatment with substances to increase permeability [47]. These include EDTA, gentamicin, tetracaine, and oxybuprocaine. However, porcine corneal studies by O’Brart et al. have highlighted the importance of complete basal epithelial cell removal for even and maximal riboflavin absorption [10]. Currently available Epi-On solutions combine components which increase the permeability of riboflavin, including Ricrolin TE (0.1 % riboflavin-5-phosphate, 15 % dextran, sodium edetate, trometamol, and NaCl), Medio-Cross TE (0.25 % riboflavin-5-phosphate hydroxypropyl methylcellulose, benzalkonium chloride and NaCl), ParaCel (0.25 % riboflavin-5-phosphate, hydroxypropyl methylcellulose, sodium edetate, trometamol, benzalkonium chloride, and NaCl), and Ricrolin plus (0.1 % riboflavin-5-phosphate, sodium edetate, trometamol, sodium dihydrogen phosphate dehydrate, and sodium phosphate dibasic dehydrate) [48].
Buzzonetti et al. reviewed children who had Epi-On CXL with 18-month follow-up, and while it was safe, the demarcation line of treatment was 105 μm with less efficacy at halting progression of KC [46]. Several further studies looking at Epi-On CXL in children showed contrasting conclusions; Magli et al. revealed a similar efficacy between Epi-Off and Epi-On CXL, but with only 12-month follow-up [49]. Caporossi et al. looked at patients 26 years or younger having Epi-On CXL, but clearly stated that those aged 18 or younger showed instability, despite many showing initial stability at 12 months [51]. This study will be presented in detail later in this chapter. Salman published the results of a case control series in children having Epi-On CXL, but again despite showing positive results only had 12 months of follow-up [47].
Currently there is little evidence to support the routine use of Epi-On CXL in children.
Iontophoresis
More recently, there has been interest in iontophoresis-assisted riboflavin delivery into the corneal stoma [46]. As riboflavin 0.1 % is hydrophilic, it is unable to cross the barrier created by healthy epithelium. Iontophoresis is a noninvasive riboflavin delivery system allowing penetration of the epithelial barrier via a small electric current administered. An annular suction ring is used to place the device holding the riboflavin solution (dextran-free), and a 5-min application of electric current via an electrode on the corneal surface and on the forehead allows penetration of the riboflavin into the stroma. Current evidence suggests that demarcation line reaches 180 μm which is still below the desired depth of infiltration [4, 10, 11, 52, 53]. There is only limited evidence of the use of iontophoretic-based CXL in pediatric cases, with a recent case series by Buzzonetti showing a demarcation line of 180 μm and apparently effective halting of progression in the 15 months of follow-up after the procedure. The initial results are promising, with no side effects documented and good tolerance, but the question remains whether the depth is sufficient to halt progressive KC.
Epithelial Disruption
The idea of making regular breaks within the epithelium to allow absorption of riboflavin yet not fully debriding the epithelium has been adopted by many in CXL. O’Brart et al. established through porcine studies that there was some transmission of riboflavin through partially debrided/disrupted epithelium, but the levels of absorption were definitely not as pronounced as with Epi-Off [10, 45]. The advent of the Daya epithelial disrupting device allowed for small full thickness breaks in the epithelium over a grid-like area over the cornea, and when combined with Epi-On riboflavin solution was found safe and effective for stabilization over the cornea over a 12-month period along with less patient discomfort [50]. There is currently no clear evidence on the effect of epithelial disruption in children. Queen Victoria Hospital in East Grinstead has its own results for CXL in children. The results so far for epithelial disruption in 25 eyes in children aged between 13 and 18 years at 12-month follow-up are promising with only two patients requiring repeat CXL for progression, 57 % gaining two lines CDVA and 7.14 % losing two or more lines CDVA. In 23.53 % of patients, Kmax increases by ≥ 1 diopter (D). There were no instances of microbial or sterile keratitis.
Barbara et al. looked at the role of the epithelium in CXL with their review of the literature in 2012 [54] and mentioned other ways of avoiding epithelial removal, including a femtosecond pocket [55]. The general consensus is that there is no CXL treatment as effective as when removing the epithelium.
Safety Concerns of Corneal Cross-linking
It is known that UVA is cytotoxic, and the eye can become damaged if UVA levels reach a certain threshold affecting the endothelium, and with higher exposure times the lens and retina can be affected too. Corneal thickness still remains a crucial marker for safety of CXL. It should be noted that riboflavin combined with adequate corneal thickness provides protection from cytotoxicity of the endothelium in CXL. However, it would be advisable to always check the corneal thickness prior to application of UVA, even in safe preoperative corneal thickness measurements. Riboflavin 0.1 % with dextran 20 % can potentially thin the cornea (75–87 μm has been documented) [11, 43, 56], to the point where endothelial damage may be a possibility. This thinning may be from evaporation from the stromal surface or colloid osmotic effect of dextran pulling water out of the stromal tissues. Similarly, when using hypotonic riboflavin in corneas thinner than 400 μm, the corneal thickness should always be measured prior to UVA application, as its effect on corneal swelling may only last 10–30 min.
Confocal microscopy has identified edema, superficial nerve loss, rarefaction of keratocytes in the anterior and mid-stroma, and isolated endothelial damage in the immediate postoperative period after CXL. Can these lead to long-term damage in the pediatric eye especially if repeated procedures are required in the future? Vinciguerra at al [33] showed in their study of 40 eyes of children with KC that endothelial cell density did not change in two years following Epi-Off CXL. Generally, in the majority of studies looking at CXL in children, there are very few safety concerns and very few documented complications post-CXL. In adult studies, there have been reports of complications such as corneal haze, sterile infiltrates, and infectious keratitis [57–60]. Corneal haze is thought to occur at the demarcation line (usually 300–350 μm) and has been reported to be persistent at 12 months in 8.6 % [61]. The specific figure in children specifically is unknown, but is thought to occur in cases with more advanced stages of KC (higher apex power, Km and thinner pachymetry). Many children who undergo CXL may however fall into this category if they have aggressive progressing KC. Similarly, with sterile infiltrates, 7.6 % of cases have been reported to have some form, but a more serious early postoperative course may occur, leading to delayed visual recovery associated with atopic disease. This must be borne in mind with children requiring CXL, as many will have atopic disease/VKC.
CXL may also reactivate Herpes Simplex Virus (HSV) in the cornea, and any signs of HSV scarring should be investigated before subjecting a child to CXL as reactivation of HSV could lead to corneal melting. HSV affecting the eye can be devastating, and even mild keratitis/keratouveitis can leave corneal scarring when recovered. Rana et al. [62] reported two cases of severe microbial keratitis resulting in corneal melt after CXL, and similarly in a review by Shetty et al. [63], despite the extremely low incidence (0.0017 %) of microbial keratitis, staphylococcus aureus is usually the culprit. Other causes in the literature include pseudomonas [64], polymicrobial [65], staphylococcus epidermidis [59], and Escherichia coli [60]. For these reasons, the recommendation would be for dual antibiotic cover such as a fluoroquinolone (ofloxacin 0.3 %) and chloramphenicol both four times a day for a week after CXL.
The effects of CXL on stem cells may also be of concern, and care should be taken when treating patients with known stem cell deficiency. Irradiation of tissues with UVA light may prevent an already compromised cornea repopulating its tissues adequately, as genes promoting apoptosis may be expressed. With a lack of in vivo studies looking at effect of UVA and riboflavin on limbal stem cells, it would be advisable to use a protecting shield around the limbus for children known to have limbal cell deficiency or when pellucid marginal degeneration (PMD) is being treated. Richoz et al. [66] investigated the effect of CXL on the limbal area on rabbit eyes and concluded that the use of CXL on sectorial areas of limbus is safe, and this was supported by a similar study by Wollensak et al. [67]. Ex vivo studies on human cadaveric eyes however have shown changes in keeping with possible oxidative/pro-apoptotic changes but no direct evidence of damage [4, 10, 68]. Animal studies are promising for safety of CXL on limbal areas, further supported by the lack of any reports of limbal cell deficiency or mutagenesis following CXL. However, there is no definite confirmation that limbal cells are protected in children who have CXL, and so in patients with preexisting limbal cell deficiency or risk of limbal cell deficiency, it would still be advisable to minimize the effects of UVA on the limbal area [69].
Is an increased corneal rigidity from CXL problem-free? The long-term results of CXL are still unknown, and there has been some concern about the ability of the cornea to absorb topical medications due to reduced penetrance through a stiffer cornea [5, 11, 70]. A change in corneal biomechanics may also change IOP measurements, with an overestimation with increased rigidity [11]. The accuracy of readings in high pressures is unknown. In adults, visual fields and accurate disk assessments with full preoperative measurements would always be ideal, but would not be possible in all children undergoing the procedure.
Intraoperative AS-OCT
AS-OCT can be used during the CXL process to assess the depth of riboflavin penetration before and after irradiation with UVA. This has been demonstrated by several studies, but recently the comparison of Epi-On and epithelial-off accelerated CXL demonstrated only a roughly 40 μm difference in depth of penetration between the two [70]. The overall depth was surprisingly lower than previously seen with standard CXL, and this is supported with other studies [71]. This technique may be beneficial for checking level of penetration prior to UVA in thin corneas and may help alleviate some safety concerns of CXL thin corneas.
Other Methods of Corneal Cross-linking
The Cretan protocol has utilized the method of combining PTK (photo-therapeutic keratectomy) with CXL [72, 73]. The technique of photorefractive surgery and CXL has been demonstrated successfully by several groups as a method of stabilizing KC and improving visual acuity [72–75]. The theory behind this method is to improve corneal irregularities which are found in KC, allow epithelial removal, and cross-link the corneal stroma. This combination would allow visual improvement with stabilization of the cornea. While the results from Kymionis [75] look promising, the method has been used for adults and may not be appropriate for children. The reasons include the removal of corneal tissue during a period of continuing visual maturation and the suggestion that the effects of CXL may not last in all children who undergo CXL.
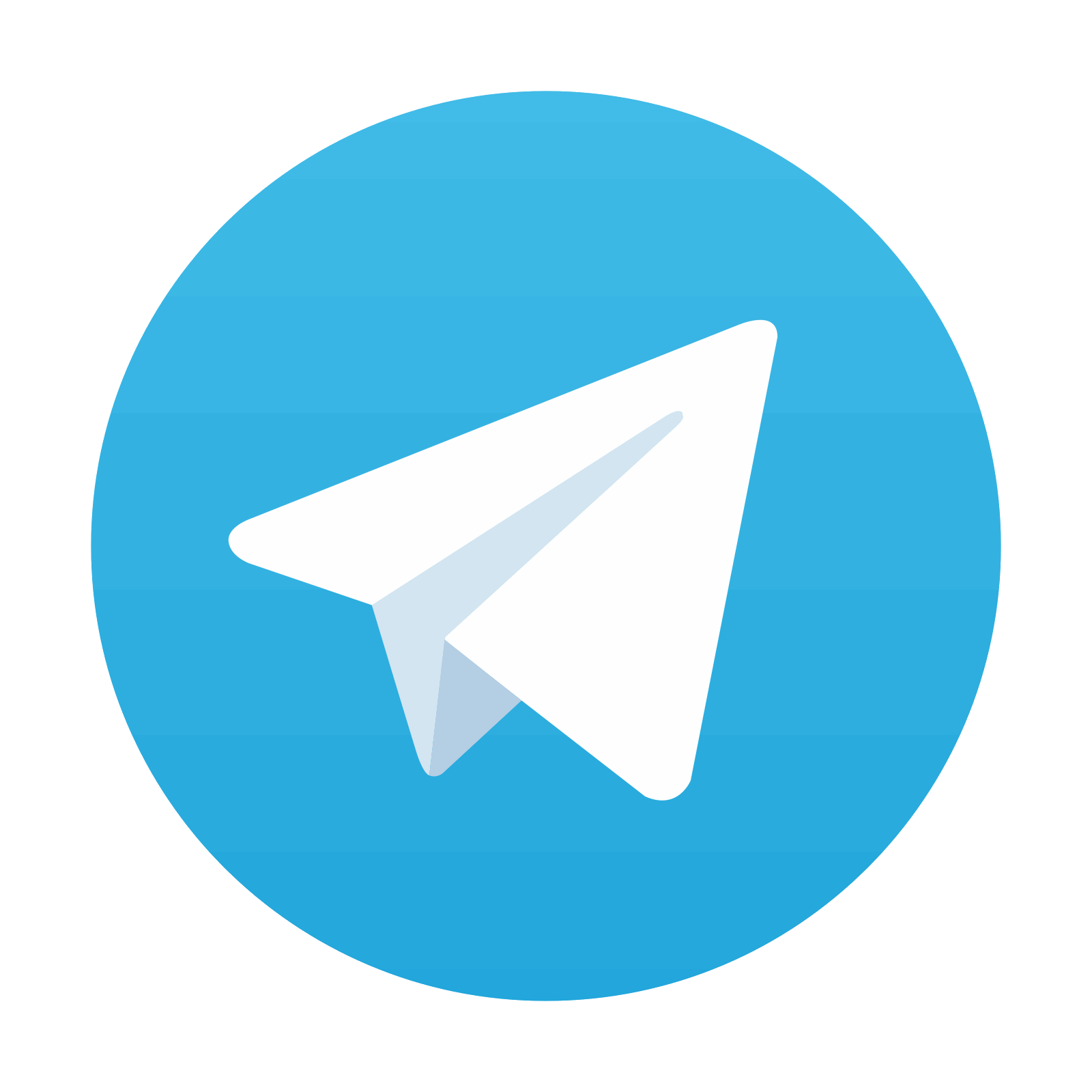
Stay updated, free articles. Join our Telegram channel

Full access? Get Clinical Tree
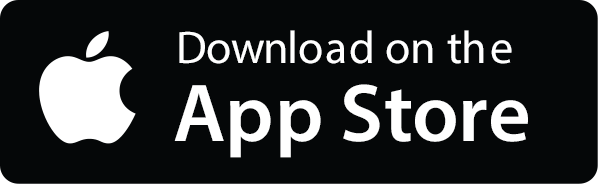
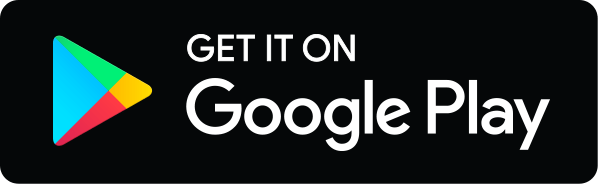