Corneal Angiogenesis
Joseph J. K. Ma
Anthony P. Adamis
Angiogenesis is defined as the formation of new vessels from vascular endothelial cells derived from existing blood vessels. Aside from female reproductive cycles and wound healing, angiogenesis seldom occurs in the normal adult; only approximately 0.01% of vascular endothelial cells divide at any given time in the adult (1,2). Although much of the prior work in angiogenesis has focused on the role and interaction of vascular endothelium in angiogenesis, recent evidence has highlighted the importance of pericytes in competent vessel formation, the presence of bone marrow-derived endothelial precursor cells, and a clarification of the roles of the cytokines involved.
Corneal neovascularization is always pathologic, and represents an important cause of visual morbidity (3). It occurs in a significant proportion of corneal transplants (41%), and is an important risk factor for corneal transplant rejection and failure (4,5). There is animal model evidence that the presence of patent corneal vessels impairs transdifferentiation (6), the process by which proliferating and migrating epithelial limbal stem cells differentiate to assume a transparent corneal epithelium phenotype (7). These new, immature vessels are friable, have increased permeability, lack structural integrity, and can result in lipid deposition and corneal opacities. It is estimated that approximately 4.14% of patients in the United States may have some degree of corneal neovascularization (8). Of these patients, it is estimated that approximately 12% may have an associated decrease in visual acuity. Both diagnostically and therapeutically, it is often useful to distinguish between neovascularization that is located deep within the stroma of the cornea from that which is superficial or associated with a superficial pannus of fibrous tissue.
ETIOLOGY
The growth of new vessels in the cornea typically results from pathologic etiologies that cause acute or chronic inflammation, stem cell deficiency, or hypoxia. In the United States, herpes simplex and bacterial keratitis are the most significant causes of neovascularization from inflammation resulting from infection. Infectious etiologies such as trachoma and onchocerciasis, however, are internationally important. Trachoma is estimated to affect 400 million people and to blind approximately 6 million people worldwide (9). Onchocerciasis affects 50 million and is estimated to blind 1 million people (10).
Stem cell deficiency leading to neovascularization of the cornea can result from trauma, alkali burns, or inflammation from diseases such Stevens-Johnson syndrome and cicatricial pemphigoid, or in association with congenital anomalies such as aniridia. Although contact lens wear is probably the most frequent cause of neovascularization from hypoxic injury to the cornea, the incidence of visual impairment from this is low. Contact lens-induced neovascularization depends on the oxygen permeability (Dk) of the contact lens material as well as duration of wear, and it often regresses with discontinuation of contact lens use (11, 12, 13). Recent advances with high-Dk lenses and daily-wear lenses are expected to decrease the incidence of corneal neovascularization secondary to contact lens wear.
THE “ANGIOGENIC SWITCH” AND STEPS INVOLVED IN ANGIOGENESIS
Angiogenesis is thought to be initiated by a disequilibrium in the balance between angiogenic and antiangiogenic factors. Collectively termed the angiogenic switch by Hanahan and Folkman (14), this theory arose from a study of tumor models and observations that certain tissues with constitutive expression of proangiogenic molecules [e.g., vascular endothelial growth factor (VEGF)] can remain without new blood vessel growth, whereas other tissues and assay systems could undergo steps in angiogenesis simply with the addition of these same molecules. In this latter group, however, angiogenesis could be blocked with increasing amounts of angiogenesis inhibitors (e.g., thrombospondin-1) (15,16). It is thought that this critical balance, which may be tissue specific (i.e., high inhibitory levels in some, low
levels of both in others), may explain why different levels of angiogenic and antiangiogenic molecules are necessary to initiate the process in any given tissue (14). The metalloproteinases (MMPs), tissue-inhibitors of metalloproteinases (TIMPs), angiostatin, endostatin, prolactin, thrombospondin-1, arresten, tumstatin, canstatin, and pigment epithelium-derived factor are all thought to be involved, either in whole or in part, with the “angiogenic switch.”
levels of both in others), may explain why different levels of angiogenic and antiangiogenic molecules are necessary to initiate the process in any given tissue (14). The metalloproteinases (MMPs), tissue-inhibitors of metalloproteinases (TIMPs), angiostatin, endostatin, prolactin, thrombospondin-1, arresten, tumstatin, canstatin, and pigment epithelium-derived factor are all thought to be involved, either in whole or in part, with the “angiogenic switch.”
The paradigm of cryptic inhibitors of angiogenesis emerged with the discovery that certain ubiquitous extracellular matrix proteins (e.g., fibronectin, plasminogen, collagen) could be degraded by proteolytic enzymes (including MMPs) into fragments with potent antiangiogenic properties (e.g., angiostatin and endostatin-like molecules) (14). Coupled with the presence of angiogenic factors [e.g., basic fibroblast growth factor (bFGF), VEGF] nested within the extracellular matrix, a paracrine balance of factors in the angiogenic switch mechanism is also thought to play an important role in homeostasis and normal physiologic regulation (14). Matrilysin (MMP-7) may play such a role in the cornea, allowing for what some have termed the angiogenic “privilege” of the cornea (17,18).
When a disequilibrium in the angiogenic switch favors angiogenesis, the cascade of steps that follows includes (a) the disruption of the basement membrane, (b) endothelial cell migration, (c) endothelial cell proliferation, (d) endothelial cell differentiation and tube formation (19), (e) vascular pruning by leukocytes (20), and (f) maturation of the blood vessels with the recruitment of pericytes (21,22) (Fig. 5-1). There is evidence that circulating bone marrow-derived endothelial precursor cells are also involved and incorporated into new vessels (22,23) and that placental growth factor (PlGF) may play a role in recruiting them (24). VEGF-A plays a vital role in the switch mechanism and steps (a) through (e). The disruption of the basement membrane is titrated by a balance between urokinase-type plasminogen activator, MMPs, cysteine proteinase systems and plasminogen activator inhibitors, TIMPs, and cystatins (25). Endothelial cell migration is facilitated by the expression of integrins (α5β3, α5β5). The maturation of the blood vessels by pericytes/smooth muscle cells appears to be mediated by angiopoietins, platelet-derived growth factor (PDGF),
and transforming growth factor (TGF)-β, with PDGF playing a critical role in pericyte recruitment. Both in vitro and in vivo assays for angiogenesis have been developed to observe the effects of various factors on each of these crucial steps, and are briefly elaborated in the following section.
and transforming growth factor (TGF)-β, with PDGF playing a critical role in pericyte recruitment. Both in vitro and in vivo assays for angiogenesis have been developed to observe the effects of various factors on each of these crucial steps, and are briefly elaborated in the following section.
ASSAYS USED IN ANGIOGENESIS RESEARCH
In Vitro Methodologies
In vitro methods allow for the rapid screening and quantification of compounds purported to be involved in angiogenesis. However, because the endothelial cells used in these assays are usually either derived from bovine aorta or human umbilical vein, their results must be tempered by the knowledge that these cell lines may not necessarily represent those present in the in vivo situation. Assays for endothelial DNA synthesis (thymidine incorporation assays), endothelial cell proliferation, migration (modified Boyden chamber assays), and tube formation in extracellular matrices such as Matrigel (a product of Engelbreth-Holm-Swarm tumor cells consisting of extracellular matrix components) are available and have been useful in differentiating the specific effects of angiogenic factors on each of these critical steps in angiogenesis. Organ culture assays, which usually involve the placement of sections of rat aorta or chick aortic arch on an extracellular matrix such as Matrigel, allow for the influence of surrounding nonendothelial cells to be factored into assay responses (26).
In Vivo Methodologies
The avascular and easily accessible cornea is one of the most commonly studied in vivo models of angiogenesis. The corneal micropocket assay is the most widely used of these, and involves the stimulation of angiogenesis by the implantation of an angiogenic substance such as endotoxin, bFGF, or VEGF (13,27,28) in sustained-release polymers or sponge material. Originally described in rabbits (29), it has been adapted to rats and mice, allowing, in the latter, study in genetically altered models. Corneal injury models of neovascularization, involving mechanical scrape, cautery, xenograft transplants, alkali injury with NaOH, suture placement, and infection, have also been studied (30,31). Although much of the fundamentals of what we know about ocular angiogenesis has been garnered from cancer research, the classic proof of principle that tumor growth and metastasis require angiogenesis was first demonstrated in tumor cells placed on a rabbit cornea assay (32).
The earliest in vivo assays for angiogenesis consisted of diffusion chambers composed of Millipore filters designed to monitor neovascularization in implanted tumors (26). Chick chorioallantoic membrane (CAM) assays, which involve the study of a fertilized chick embryo that can be transferred and incubated in a culture dish (33), have become a useful tool for studying angiogenesis. Substances incorporated on membranes, gels, or coverslips are placed on the CAM, and their effect on neovascularization can be analyzed by quantifying either surface area or bifurcation points (34,35). Their results, however, can be confounded by the vessel development associated with normal embryologic development. Matrigel plug assays (36) involve the subcutaneous injection of Matrigel impregnated with test cells. A plug is formed and then harvested for the histologic analysis and quantification of neovascularization. The effect of endogenous substances administered by peritoneal pumps or similar devices can then be studied. A number of retinal and choroidal models of neovascularization have also been developed and are useful in the study of specific disease entities (37).
IMPORTANT MOLECULAR FACTORS IN ANGIOGENESIS
The Vascular Endothelial Growth Factors
The VEGFs appear to be key regulators in angiogenesis and lymphangiogenesis. VEGF, also known as VEGF-A, was first described as a vascular permeability factor isolated from tumor-induced ascitic fluid with a vessel permeability potency 50,000 times that of histamine (38,39). Since then, five related genes have been identified: PlGF, VEGF-B, VEGF-C, VEGF-D, and VEGF-E. In humans, VEGF-A has at least 3 isoforms (121, 165, and 189 amino acids in length) that result from differential splicing of a single gene.
VEGF-A is produced by a variety of cells, including macrophages, retinal pigment epithelial cells, Müller cells, astrocytes, smooth muscle cells, and T cells, and is the most extensively studied of these molecules. It is required for vascular development during embryogenesis and can stimulate endothelial cells to undergo crucial steps in angiogenesis, including basement membrane destruction, the release of MMPs and plasminogen activators, the expression of integrins, and the formation of tubes, as demonstrated by in vitro techniques (25,40, 41, 42). VEGF-A is temporally and spatially correlated with corneal neovascularization associated with inflammation in animal models and is increased in hypoxia (31,43, 44, 45). It is thought that the latter is mediated by hypoxia-inducible transcription factors (46,47). VEGF-A upregulation has also been demonstrated in the human cornea in inflammatory disease associated with neovascularization (48,49).
The functions of the related VEGFs are currently being characterized. VEGF-B is thought to have a significant role in nonangiogenic tumor progression. VEGF-C and VEGF-D are being investigated for their role in angiogenesis and lymphangiogenesis associated with cancer, and PlGF, for its
role in vascular permeability, proliferation, chemotaxis, and angiogenesis (24,50,51).
role in vascular permeability, proliferation, chemotaxis, and angiogenesis (24,50,51).
The VEGFs interact with three receptor tyrosine kinases (RTKs) identified to date: Flt-1/VEGFR-1, Flk-1/KDR/VEGFR-2, and Flt-4/VEGFR-3. A soluble form of VEGFR-1 also exists in vivo. VEGF-A is thought to mediate its action on vascular endothelial cells primarily through VEGFR-2 and its effect on pericytes and smooth muscles cells through VEGFR-1. Neuropilin-1, which acts as a mediator of axonal repulsion in the nervous system, is a coreceptor for VEGF-A (165 isoform only), binds to VEGFR-2, and appears to play an important role in vascular development (52). VEGFR-1 is also expressed on goblet cells, and the inhibition of VEGF-A activity in an animal model of limbal stem cell deficiency suppressed VEGFR-1 in a pattern that was temporally and spatially related to the degree of corneal neovascularization. Thus, the suppression of neovascularization with VEGF-A may also inhibit the process of conjunctivalization of the cornea (53).
VEGF-C and VEGF-D can bind to VEGFR-2 and VEGFR-3, but with a higher affinity to the latter. VEGF-B and PlGF bind to VEGFR-1 and VEGF-E binds to VEGFR-2 (54, 55, 56, 57). VEGF-C and VEGFR-3 are thought to be important in the process of lymphangiogenesis as well as angiogenesis (58, 59, 60). The receptors for these related compounds and their potential roles in angiogenesis are being investigated and characterized.
Angiopoietins
Angiopoietins are glycoproteins that appear to be crucial for vessel stabilization and remodeling by acting in concert with VEGF in angiogenesis and lymphangiogenesis (47,61,62). There are two known angiopoietins (Ang1 and Ang2). Angiopoietins mediate their actions through the endothelial cell-specific Tie 2 receptor through signaling that affects the regulation of PDGF-BB (63) and its effect on pericytes and smooth muscle cells.
In blood vessels, Ang1-Tie 2 signaling recruits pericytes and smooth muscle cells to stabilize vessels, whereas Ang2 inhibits this interaction. These interactions may allow for angiogenesis in the presence of VEGF and apoptosis in its absence (64,65). Ang2 appears to be important for the recruitment of smooth muscle cells and the maturation and proper functioning of lymphatic vessels (62).
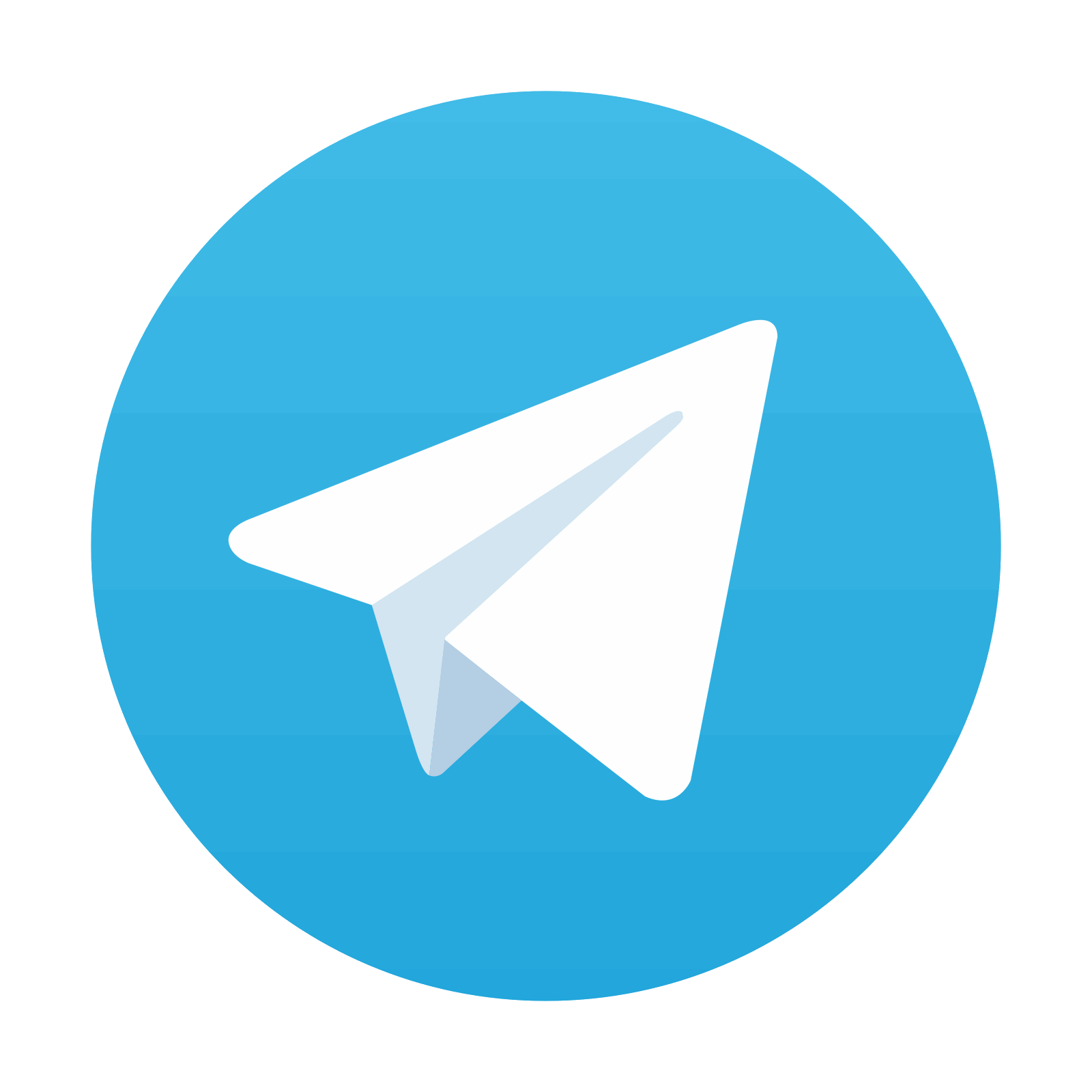
Stay updated, free articles. Join our Telegram channel

Full access? Get Clinical Tree
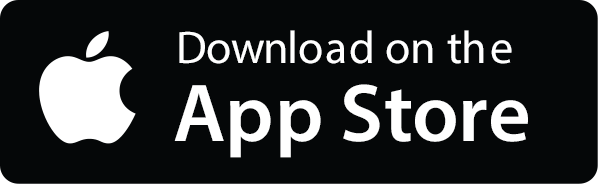
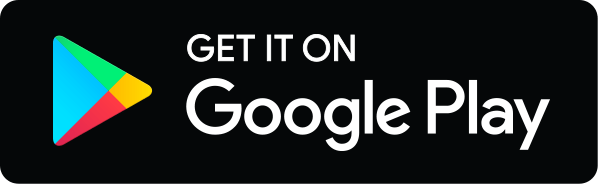