Fig. 7.1
The figure shows PFCL droplets with typical Ω-shaped profile. The superficial tension of the bubble produces an acute angle between the RPE and retina, which differs from a subretinal fluid bubble where the angle between the RPE and retina is obtuse

Fig. 7.2
The figure shows the sticky oil formation as a hyperviscous solution obtained by adding PFCL to heavy silicone oil
Heavy silicone oil (HSO), in the form of fluorosilicone, was also first used in the early 1990s, but it could only be used as a short-term tamponade, not being very useful to treat complex retinal detachments with proliferative vitreoretinopathy (PVR). In the early 2000s, perfluorohexiloctane (F6H8) was also used in some studies, but it was soon abandoned due to numerous complications [6]. Approximately in the mid-2000s, new HSOs, Oxane HD, Densiron 68 and HWS 46-3000, demonstrated less complications and better results and managed to become an excellent tamponade for inferior retinal pathology [6].
Nowadays these tamponades are much safer and have become fundamental tools when operating certain types of retinal pathologies. However, they still have a significant rate of complications. We will review the more frequent complications, the potential benefits and the risks of these tamponades.
7.2 Intraoperative Complications
7.2.1 Subretinal Migration of Perfluorocarbon Liquids
Perfluorocarbon liquids (PFCLs) have an intermediate interfacial tension against water at 50 mN/m and a dynamic viscosity of 5.10 mPas [5]. The high specific gravity, transparency and low viscosity of PFCLs make it a useful surgical tool in displacing subretinal fluid. They stabilize the retina during surgical manoeuvres inside the vitreous cavity. The retina, being hydrophilic, prefers to be in contact with water rather than with PFCLs; therefore, the retina forms a meniscus that is convex upward. Moreover, the PFCL bubble tends to remain rounded because the interfacial energy creates a net attraction inwards. These findings prevent PFCLs from reaching the subretinal space [7]. Among all PFCLs, perfluoro-n-octane (PFO) has the highest vapour pressure, which allows, in an open system, a more complete PFO removal during fluid–air exchange.
Despite accurate removal of PFCLs during fluid–air exchange, small amounts of PFCLs can be inadvertently left in the vitreous cavity, and occasionally it gains access to the subretinal space. Reported incidence of subretinal retention of PFCLs after vitreoretinal surgery ranges from 1 to 11 % [8, 9]. The residual PFCL droplets stimulate a macrophagic foreign body response with phagocytosis of PFCLs by retinal pigment epithelium (RPE) cells. Microscopy of tangential sections through the specimen of proliferative membranes shows that swollen RPE cells containing the endotamponade are able to induce an inflammatory reaction [10, 11]. Moreover, if the subretinal PFCL is located under the macula, it impedes contact between photoreceptors and RPE-inducing mechanical damage and interferes with cell metabolism and electrolytic balance. A subfoveal location causes focal toxicity to outer nuclear layers and RPE, which results in permanent scotoma or even full-thickness macular hole formation with visual loss (Fig. 7.1 ) [12].
7.2.1.1 Risk Factors
(a)
Small-gauge vitrectomy with non-valved trocar cannula
It was recently described that there is a 4.5-fold increased incidence of subretinal PFCL in eyes undergoing retinal detachment repair with small-gauge vitrectomy versus traditional 20-G vitrectomy (10.3 %, 4 out of 176 eyes with 23-G group versus 2.3 %, 6 out of 58 eyes in 20-G group) [13]. This may be due to higher fluid flow through smaller infusion lines. In order to optimize the cutting and the speed of the vitrectomy through small-gauge system, we need to use a higher vacuum set (450–650 mmHg) and higher bottle infusion (25–35 mmHg). Such settings generate high turbulence inside the PFCL if the level of PFCL fluid is close to the fluid infusion line. The low viscosity of PFCL, together with the high current of fluid, produces disrupted PFCL surface tension, which results in the formation of small bubbles of PFCL that can easily reach the subretinal space. This phenomenon is less frequent with valved trocar cannula because the trocar flow rate is slower compared to the rapid free flow of fluid that occurs through an open non-valved small-gauge cannula [7].
(b)
Incomplete release of retinal traction
In the presence of a retinal break (RB) associated with retinal traction, the specific gravity of PFCL overcomes the superficial tension of the PFCL bubble at the edge of the RB. This creates separation of the bubble that passes through the RB and reaches the subretinal space. The surgeon needs to release the traction on the edge of the RB before raising the level of PFCL above the posterior margin of the RB.
(c)
Rapid injection and aspiration of PFCL
The injection and aspiration of PFCL needs to be performed slowly in order to avoid any disturbance of the superficial tension which will result in the formation of “fish eggs” of PFCL. The tip of the cannula should be placed inside the PFCL bubble. During the fluid–air exchange, it is recommended to keep one of the trocars opened (if the trocar is valved to keep open with venting) in order to vaporize the PFCL.
(d)
Indentation manoeuvres
During the surgery, the surgeon frequently uses scleral indentation for trimming of the vitreous base or for endolaser treatment of the peripheral retina. The indentation can induce contact between the infusion line and the PFCL; moreover, rapid release of the indentation can generate high suction forces that may draw small PFCL bubbles into the subretinal space. In the presence of PFCL at the edge of RB, the scleral indentation needs to be performed gently with lower and more controlled infusion pressure.
(e)
Entrapment in residual vitreous gel
In case of incomplete trimming of the vitreous base or incomplete posterior vitreous detachment, the PFCL can remain entrapped in the vitreous gel and, subsequently, be released during fluid–air exchange or in the early postoperative period. PFCL retained pre-retinally in the vitreous cavity can migrate into the subretinal space during early changes in the patient’s posture, and a complete retina–RPE adhesion can develop [14]. The behaviour of such entrapped bubbles is unpredictable.
(f)
Extensive retinotomy and direct PFCL–SO exchange
Extensive retinotomy of 120° or larger is highly correlated with subretinal PFCL. During manipulations at the edge of the retinotomy with the flute needle, one can accidently flush PFCL remnants throughout the flute needle [9]. Moreover, in direct PFCL–SO exchange, the peripheral meniscus between the PFCL and SO may come in contact with residual retinal traction, which induces separation of the PFCL bubble that then gains access to the subretinal space.
7.2.1.2 Management
The main objective in managing subretinal PFCL is to minimize retinal damage during extraction. This involves the use of self-sealing small retinotomies performed with small-gauge cannulas. The 50-gauge or 41-gauge cannula is the smallest, but it could take longer to aspirate PFCLs even with high suction because of high resistance to flow [15]. It has been proven that longer aspiration times increase the chance of retinotomy enlargement or retinal or choroidal damage due to involuntary surgeon or patient movement. The choice of surgical approaches mainly depends on the location and size of the bubble [16].
Other technique is to induce a larger retinotomy (with endolaser or endodiathermy) when the bubble is extrafoveal. In case the location of the bubble is subfoveal, an option is to induce retinal detachment localized at the posterior pole with a 41-gauge cannula injecting balanced salt solution under the retina. Then we inject PFCL in the preretinal space allowing the bubble to move away from the fovea, and the surgeon removes the bubble through a safer retinotomy. In case the PFCL bubble cannot be removed, the patient should be placed on the nasal or temporal side in the presence of light tamponade (air, gas or SO) and then into a face-down position in order to push the subretinal PFCL bubble out the nasal periphery [17].
7.2.2 Sticky Oil Formation
Sticky oil has been described as SO-like material that remains glued to the retinal surface during SO removal [18]. Veckeneer et al. reported sticky oil formation in 28 patients in which 1,000 cSt SO and PFCL was used in the previous surgery. The authors, who used gas chromatography mass spectroscopy analysis, found that there was a significant presence of PFCL in samples of sticky silicone oil, and in all cases, the authors proceeded with direct exchange between PFCL and SO 1,000 cSt [18]. Friberg et al. using electron impact ionization mass spectrometry also reported an interaction between SO and PFCL that dissolves small amounts into solution over time [19]. Sticky oil formation has been described in eyes previously treated with PFCL and light SO and more frequently with PFCL and HSO.
HSO is a mixed compound obtained by adding a semifluorinate (alkane or ether) to SO. The saturation point of HSO is the maximum concentration of semifluorinate that dissolves in SO. HSO is a partially saturated, fluorinated compound and, therefore, more unstable than SO. The presence of PFCL induces changes in saturation points, which alters both the opacity and viscosity of the oil [20]. Solubility equilibrium is also temperature dependent. Changes in temperature change the saturation of HSO by PFCL. Temperature also affects the PFCL–HSO interaction. A decrease in temperature increases opalescence and viscosity of the solution [20].
We demonstrated that the interactions between PFCL and HSO generate a hyperviscous solution with increased shear viscosity and characteristics of that of “sticky oil”. In the absence of PFCL, hyperviscous solutions were not formed (Fig. 7.2) [20].
In surgical practice, the direct exchange of PFCL with HSO is not recommended during retinal surgery because of the direct contact between the two compounds at the oil interface.
In a recent paper, Romano et al. showed sticky oil formation in three cases in which direct PFCL–HSO was performed [21]. We believe that the defined sticky oil is a hyperviscous compound produced by interaction with PFCL. At the same time, stickiness of the compound seems to be related to a reduction in the surface tension of the surrounding aqueous material contaminated with PFCL [20]. We demonstrate in vitro that by increasing the temperature, the shear viscosity significantly decreases, with the resolution of the sticky oil [20].
7.3 Postoperative Complications
7.3.1 Emulsion and Inflammation
An emulsion is a mixture of two or more immiscible liquids. There is still some confusion regarding the differentiation between emulsification and opacification. Factors contributing to opacification include water content of SO as well as solvents that dissolve spontaneously in SO and induce phase separation. Real emulsification represents a breakdown of the originally inert bubble of SO into smaller bubbles.
Two liquids can form different types of emulsions. Regarding the compounds currently used in the human eye (oil and water), there can be (a) water-in-oil emulsion, when the water is the dispersed phase and oil is the external phase (eye filled with SO tamponade), or (b) oil-in-water emulsion, when the oil is the dispersed phase and water is the dispersion medium (eye filled with water after SO removal).
7.3.1.1 Detection
Emulsions contain both a dispersed and a continuous phase with the boundary between the phases called the “interface”. Micro- and nano-emulsions are scattered and, therefore, visible only if the droplet sizes exceed one-quarter of the wavelength of the incident light (390 and 750 nm). Thus, in the presence of droplets below 100 nm, the emulsion is not visible (no Tyndall effect), and light can penetrate through the emulsion without being scattered. The size of an emulsified SO drop removed from a patient’s anterior chamber is around 0.038 mm [22].
7.3.1.2 Formation
Silicone oil stability refers to the ability of SO to resist change in its properties over time.
In the presence of movement (saccadic eye movements), fluids develop at interfaces, and high shear stress is able to overcome the interfacial tension, which induces the formation of droplets.
The stability of a compound and the formation of microemulsion mainly depend on changes in superficial tension (ST) induced by heat, changes in pH level or changes in the viscosity.
7.3.1.3 Surface Tension
Surface tension (ST) is a contractive force in the surface of a liquid that tends to resist external force. This property is caused by cohesion of molecules at the surface that are pulled inward. Minimizations in the sphere shape the necessary “wall tensions”, which create forces at the surface to contact the minimized area. The ST is, thus, responsible for the shape and stability of liquid droplets assuming that the SOs used are purified without any irregularity in the manufacturing process.
The surfactants are the main compounds able to lower the ST.
The surfactants are mainly organic, amphiphilic compounds that contain both hydrophobic and hydrophilic groups. Therefore, it contains both an oil-soluble part and a water-soluble part.
At the interfaces, the surfactants will extend in both oil and water with two different tails. The position of surfactants at the interface alters the forces at the surface and prepares the compound for the emulsion. Human eyes with SO tamponade in the presence of two different types of surfactants, biosurfactants and detergents, have been previously described. Biosurfactants are surface-active substances produced by cells. After retinal detachment surgery, biosurfactants such as HDL apolipoproteins, red blood cell membranes and plasma lipoproteins are present at the oil–water interface. The concentration of this substance in the vitreous cavity plays a key role in the formation of emulsification. Overall, HDLs are smaller than LDLs, and when the blood–retinal barrier is broken after surgery, HDLs can reach the vitreous cavity to significant levels [22]. Moreover, RPE cells and fibroblasts also produce biosurfactants such as growth factors and cytokines [22].
The detergents are compounds that are able to solubilize sensible material by dissociating aggregates and unfolding proteins. The detergents are also routinely used to clean and sterilize reusable surgical equipment. Even small quantities of detergents left behind can modify the ST of SO resulting in reduced stability and increased emulsification [23]. Remnants of SO or cleaning substances from the sterilization processes of reusable equipment can increase the risk of emulsification in SO-filled eyes. The amount of surface-active substances necessary to modify the ST changes according to the patient’s eye and also depends on the damage of the blood–retina barrier. The surgical team should be informed of any variations in the cleaning and sterilization process of reusable instruments [23].
7.3.1.4 Viscosity
Viscosity is the measure of the resistance to the deformation induced by shear stress. In a non-Newtonian fluid, such as SO, flow properties are dependent on the relationship between the shear rate (forces applied) and the shear rate (deformation obtained). We can differentiate shear viscosity from extensional viscosity:
(a)
The shear or dynamic viscosity is measured as the resistance to flow. The shear stress is the friction induced by a coplanar stress between parcels of the fluid that are moving at different speeds. We currently use kinematic viscosity that is the ratio of the dynamic viscosity to the density of the compound measured in centistokes (cSt).
(b)
Extensional viscosity is the measurement of the resistance from the extension of the fibre and breakup of the droplet that is also defined as emulsion. In our surgical practice, the shear viscosity is described as the easiness to inject and remove SO from the vitreous cavity, whereas the extensional viscosity describes the resistance to emulsification.
The easiest way to decrease emulsification, even if it is not the most effective method, is to increase extensional viscosity. Crisp et al. demonstrated that the propensity of SO to emulsify increases according to the proportion of low-molecular-weight components and with decreasing the length of the molecular chain of the compound [24]. Silicone oil 1,000 cSt has a low molecular weight of 37 kDa, whereas SO 5,000 cSt has a higher molecular weight of 65 kDa and, thus, a higher extensional viscosity with less tendency to emulsify. Williams et al. reported a new method to increase the extensional viscosity of SO to keep a low shear viscosity. The authors suggest adding 5–10 % high-molecular-weight (HMW) SO (423 kDa) to 1,000 cSt SO. The resulting SO has the same chemical properties of those used in clinical practices but is more resistant to emulsification and is relatively easy to inject and remove [25].
Caramoy et al. found that the best proportion to attain optimal fluid elasticity is adding 10 % HMW [26]. We believe that such effort can be useful to minimize one risk factor, but emulsification is a more complex phenomenon that cannot be controlled just by viscosity, which is seen by the emulsification still present in our clinical practice (Fig. 7.3).


Fig. 7.3
Emulsification in aphakic eye 3 months after 25-gauge pars plana vitrectomy, endolaser and 2,000 silicone oil injection for rhegmatogenous retinal detachment
7.3.2 Migration of Silicone Oil
Silicone oil has a relatively low surface tension than water which is around 40 mn/m. The SO bubble is not able to resist to external forces higher than the ST. In such cases, a small bubble of SO can pinch off from the main bubble and migrate away from the vitreous cavity.
The migration of SO is responsible for decreased tamponade efficiency on the retina and for side effects related to the contact between SO and anterior segment structures.
7.3.2.1 Subconjunctival Migration
Subconjunctival SO migration occurs more often in patients treated with sutureless 23-gauge vitrectomy. The risk factors are related to inadequate oblique wound construction and scleral rigidity. It is critical to obtain the correct wound with a tunnel long enough to create an internal lip that presses against an external lip. Such construction is able to create self-sealing sclerotomies and also avoids leakage [27]. Scleral rigidity in older patients allows a more effective apposition of the lips when the trocar is removed by the end of the surgery. In younger patients, a more flexible sclera is a risk factor for incomplete closure of sclerotomies. It is important to suture sclerotomies, even 23–25G, if any doubt of leaking is present. A generous final wash of the subconjunctival space with balanced solution to clear every droplet of remaining SO is also recommended.
7.3.2.2 Anterior Chamber Migration
The risk factors for anterior chamber (AC) migration are mainly related to overfilling of the vitreous cavity or to zonular defects in phakic and pseudophakic eyes.
In aphakic eye, sometimes the iridectomy is not enough to avoid AC migration. SO dislocation can be avoided by scleral-fixated artificial iris–intraocular lens [28–30] or by artificial iris diaphragm.
SO displacement in the AC is associated with corneal endothelial damage, secondary glaucoma, cataract progression and adhesion of SO to IOL. The prompt removal of SO is required in such cases. Sometimes it is also necessary to use F4H5 as a silicone solvent to resolve the adhesion between SO and IOL or trabecular meshwork.
7.3.2.3 Subretinal Migration
SO migration into the subretinal space from the vitreous cavity may happen in the presence of strong traction that has not completely resolved before the SO injection. The subretinal localization of SO may result in photoreceptor toxicity with bad visual prognosis. The SO removal from the subretinal space can be achieved by internal or external drainage. Internal drainage needs a large relaxing retinotomy, whereas the transscleral approach seems to be relatively safe and effective [31].
7.3.2.4 Suprachoroidal Silicone Oil
Suprachoroidal SO displacement is due to the iatrogenic location of SO infusion cannula in suprachoroidal space. It is rare to have a separation between the choroid and the sclera induced by anterior proliferation, which allows egress of SO into the suprachoroidal space [32].
The oil can be surgically removed using an internal incision or a transscleral external aspiration. Perfluorocarbon liquid is injected in the vitreous cavity to help the suprachoroidal displacement of residual SO through the existing sclerotomy [33].
7.3.2.5 Intracranial Silicone Oil
Although several reports show the retrolaminar migration of SO microbubbles with subsequent severe optic neuropathy, the risk of extraocular SO penetration remains uncommon and anecdotal. The mechanism seems to be correlated to elevated intraocular pressure (IOP) with macrophages transporting SO through the optic nerve head, which is anatomically predisposed as an optic pit. Subarachnoid or intraventricular migration of SO at MRI has also been described. There is no consensus regarding SO removal from the intracranial space, and the cases reported are always asymptomatic [34–36].
7.3.3 Secondary Glaucoma
High IOP is a common complication after vitreoretinal surgery. The use of SO is related to glaucomatous damage of the optic nerve due to diverse mechanisms, such as mechanical obstruction of the trabecular meshwork or by inducing changes in the draining capacity of the trabecular meshwork.
Pathophysiology | |
---|---|
Secondary angle closure | Overfill with pupillary block |
Anterior migration of SO with mechanical obstruction of the trabecular meshwork | |
Rubeosis iridis | |
Synechiae with angle closure | |
Secondary open angle | Emulsification |
Inflammatory response of the trabecular meshwork | |
Trigger of an underlying primary open-angle glaucoma | |
Ghost cell glaucoma | |
Steroid responder glaucoma |
Increase of IOP can appear as an early or delayed complication after surgery.
Early postoperative pressure rise is frequently related to SO overfilling, migration into the AC, to surgically related inflammation or the exacerbation of a pre-existing glaucoma.
Possible mechanisms for late-onset glaucoma include emulsified SO that infiltrates the trabecular meshwork, chronic inflammatory changes, synechia formation with angle closure, rubeosis iridis or idiopathic open-angle glaucoma.
7.3.3.1 Incidence
The true incidence is difficult to establish and has been reported between 2.2 and 56 % [37–43]. With recent developments in SO and changing concepts, the rates have diminished when compared to information published in the 1970–1980s, but it is still difficult to provide a more precise incidence rate as most of the retrospective studies have different criteria regarding glaucoma timing and definition. A silicone study report observed a prevalence of long-term elevated IOP at 8 % [40]. The presence of some risk factors may help us to closely follow and give early treatment to those patients if needed.
7.3.3.2 Risk Factors
Studies have identified a variety of risk factors, although the evidence is still unclear and some characteristics have contradictory reports. The main risk factors are:
(a)
Get Clinical Tree app for offline access
Silicone oil in the anterior chamber:
(i)
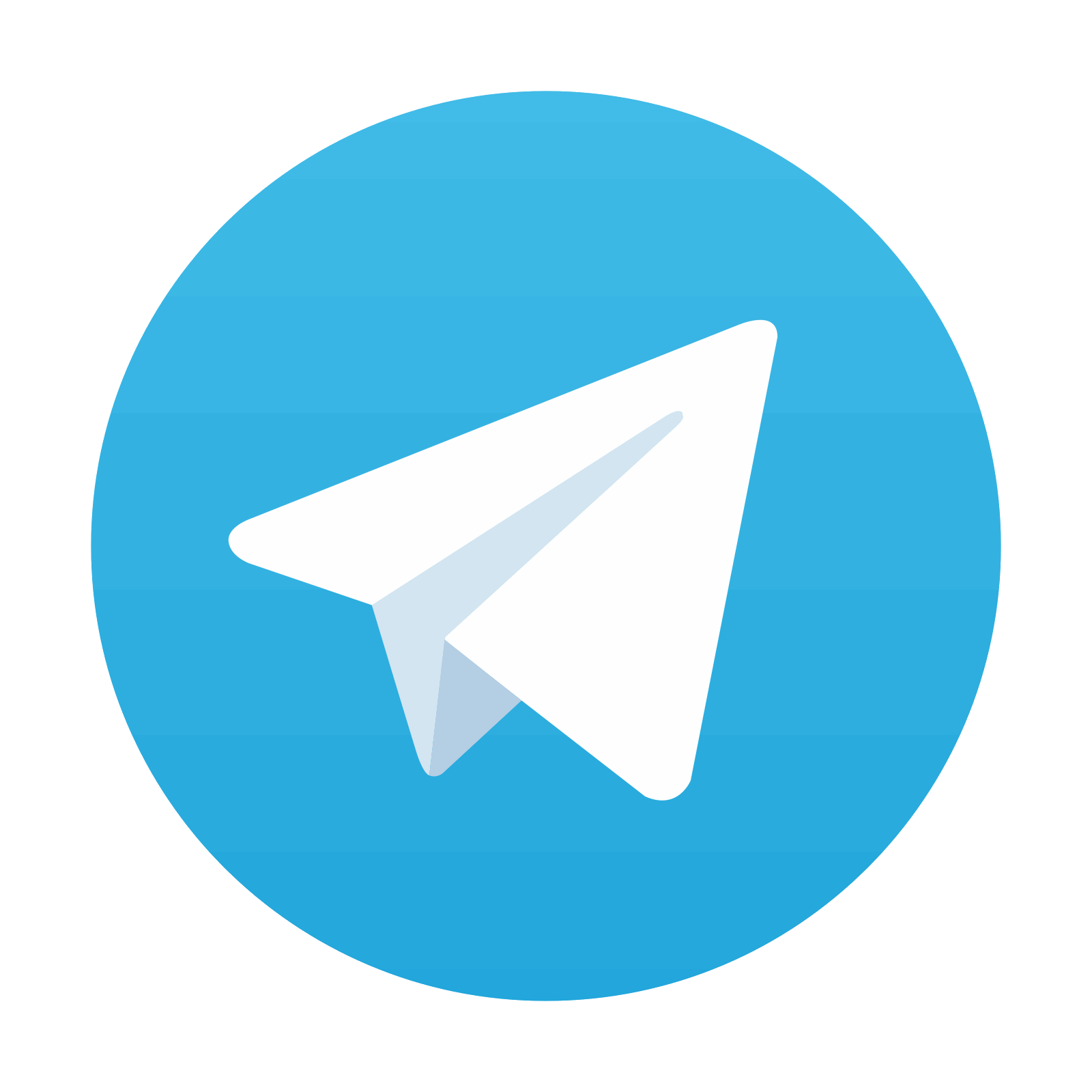
The presence of a bubble of SO in the AC is responsible for the obstruction of the trabecular meshwork [37, 38, 40, 41].
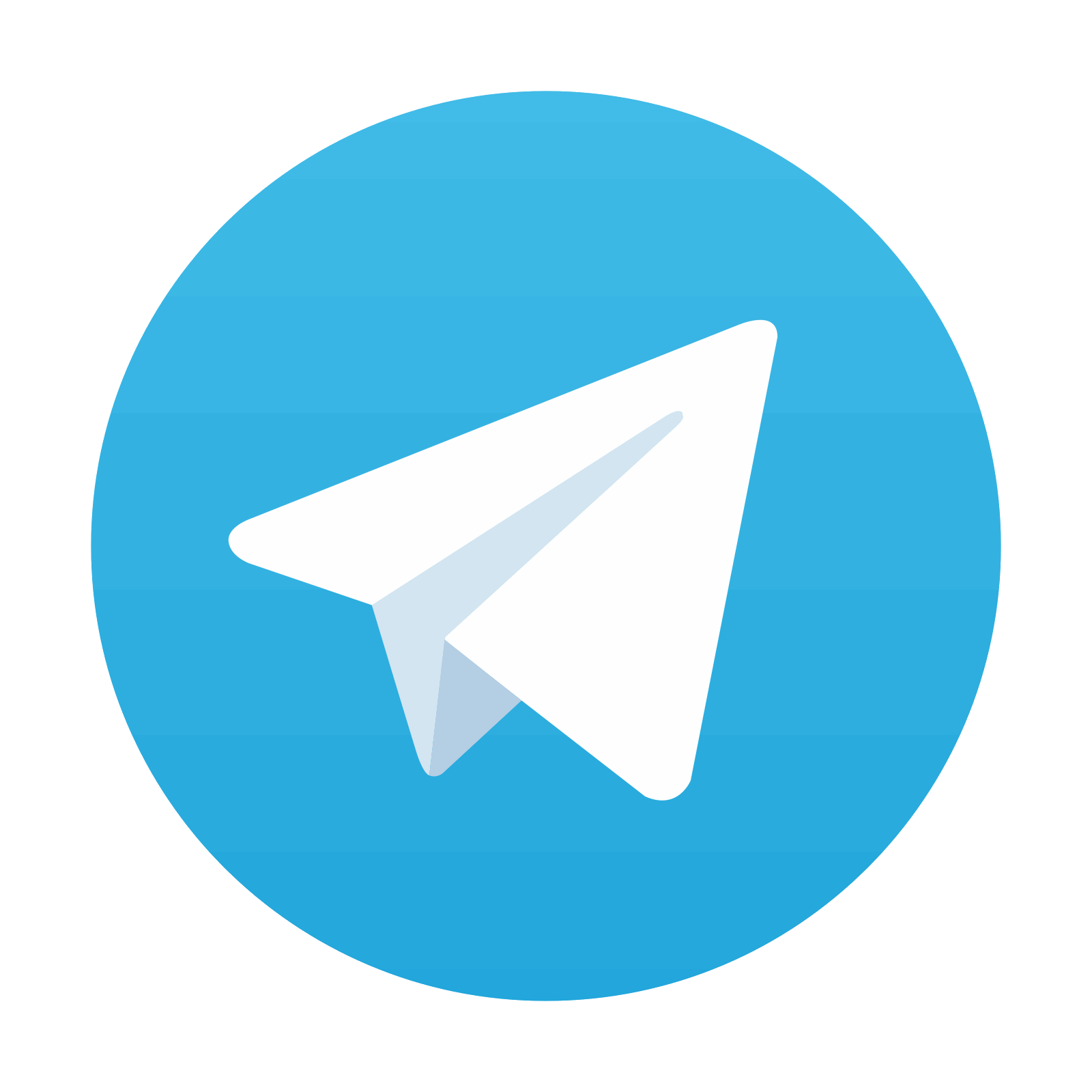
Stay updated, free articles. Join our Telegram channel

Full access? Get Clinical Tree
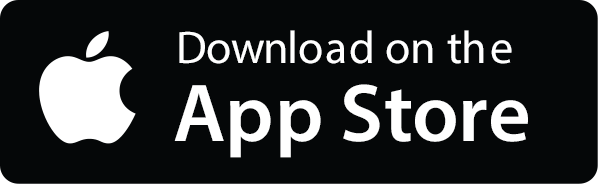
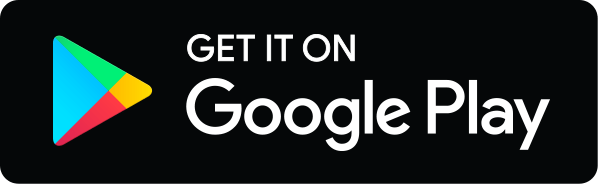
