Fig. 6.1
Intraoperative topography after epithelial removal showed an increase in power and diameter of the cone, together with flattening of the surrounding zone

Fig. 6.2
Intraoperative topography after epithelial removal and immediately after CXL showed an initial effect in reducing area and power of the cone
This difference could be caused by a thinner epithelium at the cone apex, where the stroma is steeper. The curvature changes observed are consistent with several histologic studies performed with light, transmission electron, and scanning electron microscopy, which demonstrate an overall thinning of the epithelium across the keratoconic cornea and an apparent variation in epithelial thickness with a thinning in the central region and a thickening toward the inferior keratoconic cornea [19–23]. Anterior segment optical coherence tomography (AS-OCT) and very-high-frequency digital ultrasound arc-scanning technology (described by Reinstein) showed that the epithelium is always thinner at the cone apex [24, 25]. Thus, our intraoperative findings indicate that the epithelium acts as a smoothing agent that reduces corneal power, astigmatism, and irregularity of keratoconic corneas [26–30].
This finding explains why topography obtained 1 month after CXL paradoxically shows an increase in the steepness of the cone. Instead of an effect of the CXL procedure itself, this increase results from the epithelial debridement alone. After reepithelialization, the effect of CXL in flattening and regularizing the keratoconic shape of the cornea is not evident until 6 months after the procedure.
However, the comparison of the intraoperative topography performed immediately after epithelial scraping (i.e., before CXL) with the topography obtained 1 month postoperatively showed that the procedure had a significant effect in flattening and regularizing the corneal curvature. Instantaneous map comparisons of the steepest point of the anterior surface curvature after epithelial abrasion and 1 month postoperatively showed a mean change from 61.05 to 60.54 D; the flattest point of anterior surface curvature changed from 32.77 to 34.12 D [13]. The KC regressed after CXL with flattening at the steepest point measuring an average of 11 μm after the procedure and the flat area surrounding the cone becoming progressively steeper, thus regularizing corneal curvature and reducing superior-inferior asymmetry. Long-term follow-up showed that after initial worsening of all KC indices because of epithelial debridement, the indices improved slowly but continuously for 24 months postoperatively [13]. Planned studies with AS-OCT and very-high-frequency digital ultrasound arc-scanning units may supply more data on the redistribution of the epithelium over the corneal surface after CXL.
Intraoperative Corneal Thickness
It has been demonstrated that in advanced KC (with thin corneas), using a standard isotonic solution with dextran induces a decrease of central corneal thickness (CCT), which can cause endothelial cell damage and deep stromal opacities [31]. To avoid these complications, it is advisable to check intraoperative CCT, and whenever it is under 400 μm, swelling solutions are recommended [32].
Vetter et al. in a recent report did not find a correlation between osmolality and CCT after riboflavin eye drop application but found an inverted correlation between dextran concentration and CCT [33]. The authors explained that hypertonicity and hypotonicity of solutions do not have a significant effect on stromal thickness because only 2–3 % of the corneal stroma volume consists of cells, conversely dextran possesses a high affinity for water because of its abundant hydrophilic hydroxyl groups leading to deswelling beyond the physiological level. For this reason, we suggest to evaluate not only the osmolality of the solution but also the concentration of dextran.
In conclusion, our studies confirmed the literature finding that a preoperative high keratometry is a positive predictive factor [31, 32]. Furthermore, our findings add that keratoconic corneas with very low pachymetry are more likely to improve. For this reason, we suggest to treat advanced KC also and in case of TCT below 400 μm to use swelling solutions.
Corneal Biomechanics: Ocular Response Analyzer Parameters
Preliminary clinical studies have assessed keratoconic corneal biomechanical weakness with the Reichert Ocular Response Analyzer (ORA; Reichert Ophthalmic Instruments, Buffalo, New York), the first simple device able to provide an in vivo dynamic measurement of corneal viscoelastic behavior [33]. Several studies have shown a significant variation of two of the parameters measured by the ORA, corneal hysteresis (CH), and corneal resistance factor (CRF), which are significantly lower in keratoconic eyes compared with normal eyes [34, 35].
CH, the difference between inward applanation amplitude peak 1 and outward applanation amplitude peak 2, is a function of the corneal viscous-damping properties and is likely linked to the stromal collagen nature and state of hydration [36]. CRF, also a viscoelastic parameter, is calculated using a linear combination of peak 1 and peak 2 and more heavily weighted by the underlying corneal elastic properties [37]. Corneal-compensated intraocular pressure (IOP) measurement is strongly correlated to Goldmann tonometry and is also calculated using a specific linear combination of peak 1 and peak 2 [37]. Corneal-compensated IOP should have little correlation with CCT measured by ultrasound pachymetry, and it has been reported to remain fairly constant after refractive surgery [36]. The amplitude of the peaks (peak 1 and peak 2) is a function of how much light hits the infrared detector during each applanation event. If the applanation area is large, the peak amplitude will be large; if it is small, the peak amplitude will be small. Deformed ectatic corneas seem to have an abnormal signal shape, as described by Kérautret et al. [38].
We performed a study with intraoperative ORA measurements on keratoconic eyes undergoing standard CXL [39]. The patient was asked to rise and move to the ORA, OPD-Scan, and Pentacam units where images were taken after the instillation of saline drops. After the examinations, the patient returned to the supine position and was draped anew, the eye rinsed with balanced salt solution (BSS), and a lid speculum inserted. The surgeon then proceeded with CXL.
In this study, CH and CRF showed similar behavior during and after CXL. Corneal deepithelialization did not induce any change in CH and CRF; however, both parameters increased during CXL at the end of the impregnation and irradiation phases. Corneal epithelium did not seem to affect the structural stability of the cornea, which differs from the most anterior part of corneal stroma (100–120 μm), possibly responsible for the stability of corneal shape, as stated by Muller et al. [40].
Corneal deepithelialization influenced the peak amplitudes, which decreased statistically significantly. As Kérautret et al suggested in their study, the peak amplitudes were negatively correlated with KC severity, being an indicator of small applanation area and nonuniform corneal deformation [38]. After the procedure, CH and CRF remained statistically significantly higher than the preoperative values at 1-month follow-up; conversely, at 6 and 12 months postoperatively, no statistically significant changes were noted. Deepithelialization, riboflavin impregnation, and UVA irradiation significantly reduced peak 1 and peak 2 amplitude [39]. Both parameters remained significantly low after contact lens removal. However, the amplitude of peak 1 and peak 2 increased postoperatively significantly to double the preoperative value at 6 and 12 months. Corneal-compensated IOP and Goldmann-correlated IOP did not change significantly during the CXL procedure. This study showed how corneal deepithelialization, riboflavin impregnation, and UVA irradiation increased the steepness and asymmetry of keratoconic eyes with a progressive reduction of corneal thickness as well as of peak 1 and peak 2 during the entire procedure.
As shown by the intraoperative topographic analysis, soon after corneal deepithelialization, apical corneal power increased, the cornea became steeper, the area applanated by the ORA became smaller, and the corneal deformation became less uniform with a reduction of peak amplitude. In addition, after corneal deepithelialization, the surface of the cornea became qualitatively rougher and uneven, which might explain lower signal amplitudes at the ORA examination. To further understand the increase in CH and CRF during the impregnation and irradiation phases of the CXL procedure, it was important to evaluate the corneal thickness change during the procedure itself. As expected, the TCT decreased after deepithelialization. In addition, we observed a continuous reduction of the TCT during the first and second phase of CXL, with a 15 % loss of thickness after the impregnation phase and a 21 % loss of thickness after the UVA irradiation phase [39].
As mentioned above, this thickness reduction could be due to the dehydrating effect of T-dextran, which is part of the riboflavin solution. Despite this thickness reduction, we did not observe any harmful effect on the endothelial cell count, which remained stable over 1 year postoperatively. CH and CRF, which are related to the damping nature of the cornea (e.g., collagen structure, hydration state), significantly increased in these two phases (Fig. 6.3). On the contrary, peak amplitudes did not change statistically significantly compared to the deepithelialized corneal values, probably because corneal surface was qualitatively rougher and uneven, providing lower signal amplitudes at the ORA examination. These results suggest that the dehydrated cornea was more resistant and stiff.


Fig. 6.3
Biomechanical improvement immediately after CXL, with increase in CH and CRF
During the reepithelialization phase, corneal thickness increased significantly due to corneal edema observed at the slit lamp a few days after CXL, when the bandage soft contact lens was removed. CH and CRF decreased but showed no statistically significant difference from the preoperative values, and peak amplitudes remained low. These results suggest that the edematous cornea was less elastic, less resistant, and weak. The fact that no statistically significant difference occurred in CH and CRF after CXL compared with baseline suggests that the change in stiffness may be less than that which can be measured by the sensitivity of the ORA, or it might indicate that CXL changed both elasticity and viscosity in a manner that was not detected by the viscoelastic parameters, CH and CRF [41]. CXL did not induce any effect on IOP, as measured by the ORA. Goldmann-correlated IOP and corneal-compensated IOP did not change significantly over the first postoperative year, except during the first postoperative month, after which the steroid therapy was tapered.
Results of Corneal Collagen Cross-linking in Postrefractive Surgery Ectasia
Introduction
Patients undergoing excimer laser refractive surgery expect high-quality visual results. The rare occurrence of postsurgical ectasia is thus particularly dramatic in this population, due to the inherent progressively decreasing visual acuity, and its potential for severe litigation [42]. The traditional armamentarium of the ophthalmologist for this complication includes rigid gas-permeable (RGP) contact lenses, decrease in intraocular pressure, intrastromal corneal ring (ISCR), and – the most frequent choice in the past – corneal transplantation [43, 44]. We present the results obtained with CXL in ectasia after excimer laser refractive surgery.
Personal Experience
Our personal experience at the Cornea Service of the Ophthalmology Department of Istituto Clinico Humanitas (Rozzano, Milano, Italy) deals with 78 eyes of 54 consecutive patients (36 females, 18 males) in which postexcimer laser refractive surgery ectasia progression was detected. Ectasia progression was confirmed by serial differential corneal topographies and by differential optical pachymetry analysis in all eyes, as previously described [45, 46].
The age of the patients included in the study ranged from 30 to 59 years, with a mean value of 40 years. Of the treated eyes 24 were right and 30 were left eyes. Exclusion criteria included corneal thickness <350 μm at the thinnest point, a history of herpetic keratitis, severe dry eye, concurrent corneal infections, corneal opacities, concomitant autoimmune diseases, and any previous ocular surgery with the exception of excimer laser refractive surgery [14–18]. Also excluded were pregnant or nursing women, patients with poor compliance, and patients wearing RGP lenses for at least 4 weeks before baseline examination.
At baseline and at each of the postoperative follow-up exams (3, 6, and 12 months), we performed on all patients UDVA and CDVA assessment, slit lamp biomicroscopy, basal Schirmer test, Goldmann tonometry, dilated fundus examination, endothelial biomicroscopy (Konan Specular Microscope, Konan Medical Inc, Hyogo, Japan), corneal topography as well as corneal, internal and total aberrometry with the Optical Path Difference Platform (OPD, NIDEK, Gamagori, Japan) and Pentacam HR central pachymetry and optical tomography (Oculus Inc., Lynnwood, WA, USA).
Nidek OPD device was also used to study the 21 Klyce indices provided by the Corneal Navigator Topo-Classifier Map. Pentacam HR provided analysis of the 7 Ambrosio KC indices [47]. Anterior chamber analysis was also performed with the Oculus Pentacam HR, including pupil center pachymetry and TCT [48–52].
Endothelial biomicroscopy was performed with the Konan Noncon Robo (Konan Medical Inc., Nishinomiya, Hyogo, Japan) device manually according to the method described by Prinz et al. [53].
Cross-linking Procedure
The procedure followed the standard Dresden protocol and has been described previously [13, 32, 39, 54, 55].
Before starting UVA irradiation, photosensitizing riboflavin 0.1 % solution (10 mg riboflavin-5-phosphate in 20 % dextran-T-500 10 ml solution: Ricrolin, Sooft, Montegiorgio, AP, Italy) was applied onto the cornea every minute for 30 min to achieve adequate penetration of the solution. This treatment was performed only when corneal thickness was 400 μm or above.
In six eyes, corneal pachymetry ranged between the minimum usually accepted for CXL, 400–350 μm; thus, before irradiation we performed corneal thickness expansion by swelling. Usually, 15 min of riboflavin-5-phosphate solution application was necessary to properly expand corneal thickness. Once 400 μm was reached, the procedure went on to the following step.
The cornea was exposed to a UV source emanating from a solid-state device (UV-X ™ System Peschke Meditrade GmbH, Huenenberg, Switzerland), which emits light at a wavelength of 370 ± 5 nm and an irradiance of 3 mW/cm2 or 5.4 J/cm2. Exposure lasted for 30 min, during which time riboflavin solution was again applied, this time once every 5 min. The cropped light beam has a 7.5 mm diameter. A calibrated UVA meter (LaserMate-Q; Laser 2000, Wessling, Germany) was used before treatment to check the irradiance at a 1.0 cm distance. Patients were examined every day until reepithelialization was completed and then at 1, 3, 6, and 12 months, and yearly thereafter.
Results
We treated 78 eyes. Maximum follow-up period was 5 years and mean follow-up 1.94 years. All the patients previously had excimer laser refractive surgery, either PRK (20 %) or LASIK (80 %), and were referred from other centers. The reasons for biomechanical instability after excimer laser refractive surgery were due to formerly undiagnosed forme fruste keratoconus (FFKC) in 40 % of eyes, and high correction with reduced corneal thickness in 10 % of eyes. The cause could not be identified in the remaining 50 % of eyes. UDVA and CDVA data, expressed in LogMAR and covering the entire follow-up period, are summarized in Table 6.1 and CDVA is presented in Fig. 6.4. UDVA improved significantly at last follow-up examination (2 years). CDVA improvement was statistically significant (P < 0.05) beyond 6 months postoperatively when compared to the preoperative levels.

Table 6.1
UDVA and CDVA in the 13 treated eyes before and after CXL
Pre-CXL | 3 months | 6 months | Final (2 years) | |
---|---|---|---|---|
Eyes | 78 | 78 | 68 | 45 |
UDVA (mean ± SD) | 1.08 ± 0.43 | 1.05 ± 0.45 | 0.93 ± 0.39 | 0.87 ± 0.43 |
CDVA (mean ± SD) | 0.19 ± 0.15 | 0.14 ± 0.12 | 0.09 ± 0.10 | 0.05 ± 0.08 |
SE (mean ± SD) | −4.16 ± 2.90 | −3.05 ± 3.54 | −2.16 ± 2.43 | −2.80 ± 2.42 |
Sphere (mean ± SD) | −2.96 ± 2.63 | −1.96 ± 3.24 | −1.33 ± 2.53 | −1.95 ± 1.61 |
Cylinder (mean ± SD) | −2.40 ± 2.06 | −2.19 ± 2.14 | −1.68 ± 1.74 | −2.33 ± 1.90 |

Fig. 6.4
Post-CXL CDVA over a 3-year period
Refractive results are presented in Table 6.1. The reduction in mean sphere and astigmatism was statistically significant (P < 0.05) at 6 months and beyond.
Topographic astigmatism measured with the Nidek OPD-Scan during follow-up is shown in Table 6.2. Mean baseline flattest meridian keratometry, steepest meridian keratometry, and mean keratometry were 43.29 D, 45.76 D, and 40.63 D, respectively. At 12 months, these readings were 42.44 D, 44.77 D, and 40.42 D, respectively. This difference was not statistically significant.
Table 6.2
Topographic astigmatism measured with the NIDEK OPD Scan
Pre-CXL | Final (2 years) | |
---|---|---|
Eyes | 78 | 45 |
Sim-K_Kf (mean ± S.D.) | 43.29 ± 5.23 | 42.44 ± 3.43 |
Sim-K_Ks (mean ± S.D.) | 45.76 ± 6.25 | 44.77 ± 4.83 |
Sim-K AVG (mean ± S.D.) | 40.63 ± 4.38 | 40.42 ± 3.85 |
At 3 months postoperatively, the Klyce indices CVP, SDP, LogMAR, SRC – obtained with the Nidek OPD platform – showed significant decrease (P < 0.05) [56].
Figures 6.5 and 6.6 show differential map in two cases: preoperatively vs. 6 years after treatment. Central corneal flattening is clearly visible.



Fig. 6.5
Case 12. OD, LASIK for −4.25 –0.75 (145). Differential map between preoperatively (above, right) – CDVA is 0.30 LogMAR with −3.00 −5.50 (70) – and 6 years after CXL (above, left), CDVA is 0.00 LogMAR with −3.00 (70). Note reduction of ectasia on differential map (below)

Fig. 6.6
Case 13. OS, LASIK for −4.00 –0.50 (125). Differential map between preoperatively (above, right) – CDVA is 0.40 LogMAR with 2.75 −6.00 (95) – and 6 years after CXL (above, left), CDVA is 0.00 LogMAR with −0.50 −4.00 (95). Note reduction of ectasia on differential map (below)
The Ambrosio indices obtained with Oculus Pentacam HR were analyzed at baseline and at 3, 6, and 12 months. At 6 months postoperatively, the IVA index of Ambrosio had significantly increased, and the KCI index had significantly decreased (P < 0.05) [47].
Higher-order corneal aberrations, namely, coma, spherical aberration, and high-order astigmatism, were measured preoperatively and at 3, 6, and 12 months, using the Nidek OPD aberrometry program. Mean corneal coma, spherical aberration, and high-order astigmatism had decreased by the 1-year follow-up, but the difference was not statistically significant, when compared with the preoperative data.
Mean Pentacam pupil center pachymetry and total corneal volume at baseline were 436.92 ± 45.28 μm and 57.44 ± 2.58 mm3, respectively. At 12 months, they had decreased to 409.75 ± 13.35 μm – a difference that was statistically significant (P < 0.05) – and 55.30 ± 2.87 mm3, respectively.
TCT decreased from 427.55 ± 47 .33 μm to 396.75 ± 18.57 μm (P < 0.05) at 1 year, anterior chamber volume slightly decreased, from 223.27 ± 38.31 mm3 to 222.00 ± 28.90 mm3 (P < 0.05), anterior chamber depth did not change significantly, and anterior and posterior elevations changed from 5.63 ± 0.65 mm and 7.47 ± 1.00 mm to 7.48 ± 0.93 mm (P < 0.05), and 5.56 ± 0.71 mm, respectively. A statistically significant increase in corneal thickness was found at 0 and 2 mm at the 6-month control, but at the 12-month control they were, respectively, decreased (P < 0.05) and stable.
The difference in endothelial cell count between baseline and 12 months was not statistically significant (P > 0.05), indicating that CXL did not induce endothelial cell damage.
No ocular or systemic adverse events were observed, as well as no epithelial ingrowth or diffuse lamellar keratitis, and no significant intraocular pressure changes were seen.
Discussion
The first case report of CXL treatment in post-LASIK ectasia was published in 2005, by Kohlhaas et al. [57]. A similar case report by Kanellopoulos was published in 2007, with PRK applied after CXL [58]. In the same year, Hafezi et al made a similar report on 10 contact lens-intolerant eyes, with a 1-year follow-up, observing stabilization in 5 eyes and regression of keratectasia in the other 5 eyes [59]. Kymionis et al. reported on a case series of 5 eyes of 5 patients with post-LASIK ectasia. Patients were followed for 1 year with confocal microcopy study after CXL. Corneal alterations, consisting of keratocytes disappearance in anterior and intermediate corneal stroma for the first 3 months after CXL, were similar in both keratoconic and post-LASIK ectatic eyes [60, 61]. It is well known that pregnancy may induce a relapse in the advancement of keratectasia even after CXL [62].
From the psychological point of view, refractive surgery patients with corneal ectasia may be profoundly different from KC patients, being accommodated to emmetropia, complaining earlier of visual disturbances and thus presenting when ectatic changes are not advanced.
Thus, we considered the selection criteria for CXL adopted for keratoconic eyes only partially valid for postrefractive ectasia eyes. In particular, we did not accept minimal corneal thickness of 400 μm as an absolute limit. Since progressing ectasia may lead to penetrating keratoplasty (PKP), we chose to apply corneal expansion procedure with riboflavin-5-phosphate solution application in corneas thinner than 400 μm [63]. Up until now, the three eyes treated by this way did not show any complication and behaved similarly to the other eyes with thicker corneas.
In this study, we observed corneal changes similar but less marked than those observed after CXL on keratoconic eyes [13, 39, 54]. As in KC, the situation remained stable up to 6 months. At the 6-month interval, we noticed improvement in refraction and CDVA. Traditional topographic indices showed that corneal curvature remained remarkably stable at the 1-year interval. Since SimK values do not consider the entire corneal surface, we analyzed the Klyce and Ambrosio indices for KC. These indices did not deteriorate at year 1. We observed corneal curvature stabilization and also a tendency to central flattening, with improvement of the refractive situation. This is in accord with what reported by Hafezi [62]. It is important to remember that post-LASIK ectatic cornea is initially an oblate surface. Thus, even a central small flattening may lead to recentration of the optical zone and to variations in central corneal refractive power.
CXL appeared thus to stabilize these iatrogenic ectatic eyes. Reduction in sphere, increase in CDVA, apparent central corneal flattening, and tendency to decrease in coma at the 6-month interval may indicate a tendency to improvement of the ectatic situation, with possible partial recentration of the optical zone.
Moreover, it is well known that in advanced KC (with thin corneas), use of a standard isotonic solution, which includes dextran, induces a decrease of CCT that can cause endothelial cell damage and deep stromal opacities [31]. To avoid these complications, it is advisable to check intraoperative CCT and in case of thickness reduction to below 400 μm, use of swelling solutions is recommended [7, 32]. In ectatic patients, residual corneal thickness may prohibit use of the standard Epi-Off technique, because of possible endothelial cell damage. A hypotonic solution to induce corneal swelling and intraoperative pachymetry are the key to solve the problem. Controlled intraoperative swelling provides the opportunity for an Epi-Off technique, by eliminating the epithelium, the most important barrier to deep riboflavin penetration. Since the epithelium-on (Epi-On) CXL technique provides only limited penetration of riboflavin, in post-LASIK ectatic corneas, the CXL effect would be limited mostly to the flap. Thus, flap wrinkles could be induced or worsened, without providing a substantial increase in corneal stiffness of the residual stroma.
Another important concept is the limited predictability of CXL. The flattening effect continues in time and cannot be defined with precision. Adding to CXL, a second excimer refractive procedure may reflect the surgeon’s desire to rehabilitate the patient, but it lacks sound refractive logic in improving long-term vision.
Finally, the psychological effect of CXL in post-LASIK ectatic corneas must be considered. A patient who faced refractive surgery to reduce the daily burden of a refractive defect, and after a few years, experiences a high unstable refraction related to ectasia, as well as a new and more dramatic ocular surgery such as PKP and ends up again with a marked, lifestyle-limiting refractive defect. In our experience, patients with ectatic corneas after LASIK who had to undergo PKP often developed a marked resentment towards their surgeon, leading to a medical malpractice case.
In conclusion, CXL appears to improve CDVA in eyes with postrefractive surgery ectasia. No deterioration of the Klyce and Ambrosio indices was observed, as well as no permanent side effects.
Results of Corneal Collagen Cross-linking by Iontophoresis
Basic Principles of Iontophoresis
Removal of corneal epithelium is the mainstay to achieve adequate riboflavin penetration in the corneal stroma in the standard CXL protocol. This causes discomfort, temporary vision reduction, and risk of infection [32, 64, 65]. A CXL procedure warranting epithelial integrity but retaining maximal efficacy has been persistently pursued. Nevertheless, results of Epi-On CXL are controversial, with evidence showing that treated patients continue to progress and others showing good results [66–69].
Corneal epithelium may block UV penetration and riboflavin penetration, and this may be important for understanding the reduced effect of Epi-On CXL [70, 71]. For years, the epithelium has been considered a physiological barrier to UV light [70, 71]. However, as reported by Kolozsvári et al, epithelium and Bowman’s layer mostly absorb UV-B light (up to 300 nm), while UV-A light is not absorbed [72]. Bottós et al explained the reduced efficacy of Epi-On CXL with respect to standard CXL, with the hypothesis that the corneal epithelium reduces riboflavin penetration [73].
A novel approach to enhance riboflavin penetration into the corneal stroma is based on iontophoresis, a noninvasive system aimed to enhance the delivery of charged molecules into tissues, using a small electric current [74]. Iontophoresis enhances drug delivery by two principal mechanisms: electrorepulsion and electroosmosis. Electrorepulsion is the direct effect of the applied electric field on a charged molecule. The electroosmosis is due to the fact that the tissues support a negative charge at physiological pH. Iontophoretic drug delivery is based on the movement of ions under the influence of an applied electric current. A potential difference is established between a positive pole of the circuit (anode) and a negative one (cathode) so that ions move in the direction opposite to their charge [74, 75].
It has been demonstrated that iontophoresis generates a higher molecule concentration compared to agent-free techniques. This approach was used to deliver drugs in various fields of medicine, including dermatology, orthopedics, oncology, and ophthalmology mainly to reach intraocular compartments for the treatment of posterior segment diseases [76, 77]. Recently, the use of iontophoretic systems was proposed for riboflavin intracorneal delivery in CXL [78]. Riboflavin, in the formulation used for iontophoresis, is negatively charged. It has been shown that an iontophoresis imbibition lasting 5 min achieves a sufficient riboflavin concentration in corneal stroma for CXL treatment, with the advantage of shortening the imbibition time while preserving epithelial integrity.
Basic Research Results
Now available are several basic evidences in scientific literature, confirming ex vivo the effectiveness of iontophoresis imbibition applied to CXL.
To assess corneal riboflavin concentration after iontophoresis imbibition into corneal stroma, high performance liquid chromatography (HPLC) was used in rabbit and human models [79, 80].
Testing the entire cornea in rabbit, HPLC showed that iontophoresis allowed riboflavin diffusion with two-fold less riboflavin concentration than conventional application [79].
In human cadaver corneas, HPLC technology was applied to assess differences in riboflavin concentration at different stromal depths after standard, Epi-On, and iontophoresis imbibition [80]. Femtosecond laser was used to divide the entire cornea in 3 stromal lamellae (anterior, intermediate and posterior), and then the amount of riboflavin was tested in each specimen. As observed in rabbit, the overall stromal concentration of riboflavin after Epi-Off imbibition was about twice that observed with iontophoresis; otherwise, compared with the Epi-On technique, a double riboflavin concentration was observed in the entire corneal stroma in iontophoresis. The mean riboflavin content presented an evident reduction with increasing depth in all groups with higher values in the anterior corneal slice, gradually decreasing in the intermediate and in the posterior slices [80].
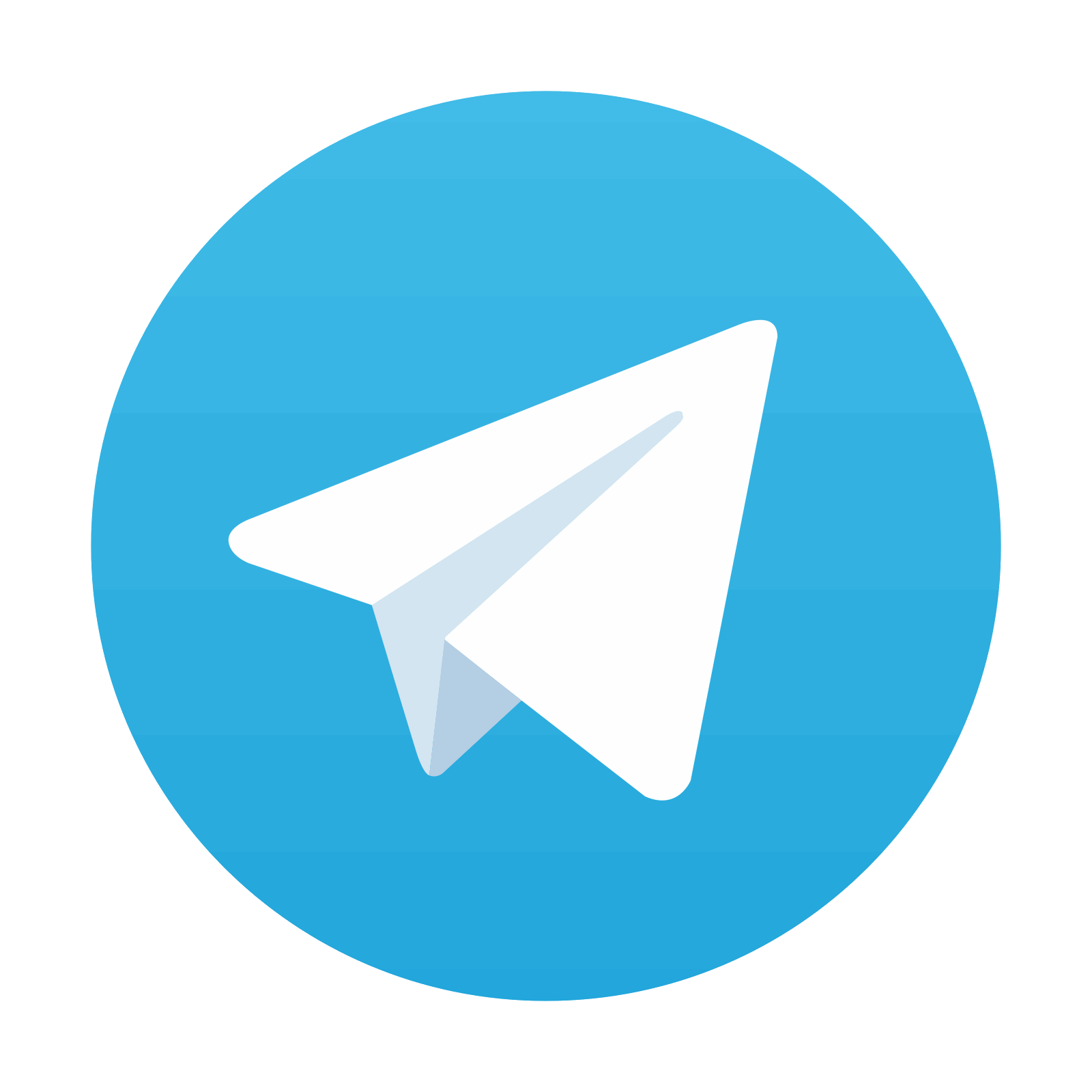
Stay updated, free articles. Join our Telegram channel

Full access? Get Clinical Tree
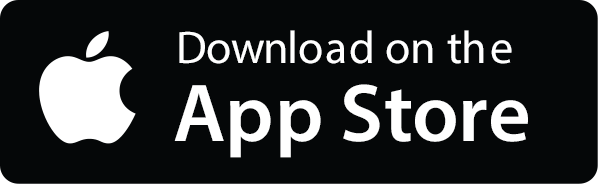
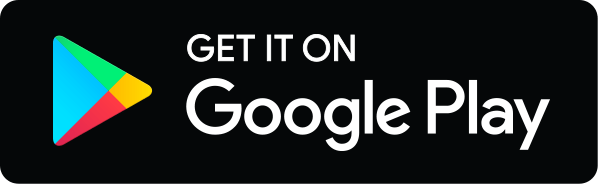