Fig. 6.1
Optic disc measurement is essential in glaucoma evaluations. For instance, despite similar cup-to-disc ratio, due to the size, optic disc A may be normal while optic disc B may be pathological (Note: The millimeter scale shown is a representation of the numbers read in the reticule of the slit lamp multiplied the correction factor for the indirect lens used)
Table 6.1
Characteristics of glaucomatous optic neuropathy by appearance
Characteristics of glaucomatous optic neuropathy (ON) | |
---|---|
On appearance | Associated clinical characteristic |
1. Focal ischemic damage | Female predominance |
Vasospastic disorder tendency (migraines) | |
2. Senile sclerotic discs | Older patients |
Systemic vascular abnormalities | |
3. Myopic glaucomatous optic disc | Younger patients |
4. Concentric optic disc | Less prevalent systemic disorders |
Importantly, all comments about magnification using the different types of lenses are based on assumptions that do not completely hold true in clinical use. Likewise, correction factors provided by lens manufacturers are based on assumptions that do not completely apply in clinical practice. Although these numbers are good estimates, they should not be taken as absolutely correct.
Optic Disc Photography
Capturing an image of the optic disc with a fundus camera yields a simple, relatively low-cost [22], and objective record of the appearance. However, interpretation of photographs is subjective, leading to considerable inter- and intraobserver differences in classification of the optic nerve as normal or glaucomatous [18, 22, 23]. Photographs are subject to variations in technique and quality. Traditionally, fundus cameras required dilation, and images were recorded on film. Currently, non-mydriatic and digital fundus cameras are available. A stereoscopic camera produces two slightly dissimilar images by photographing the optic disc from two different angles. When the two photographs are viewed simultaneously through a stereoscopic viewer, the image appears 3-dimensional. Optic disc stereo photography is the most widely [22] used system for objectively documenting structural damage caused by glaucoma. It has also been used as an ophthalmoscopy teaching aid to residents and medical students [19]. Masked subjective, stereo grading by glaucoma specialists provided better discrimination of glaucomatous from non-glaucomatous eyes than other objective imaging [24, 25].
Retinal Nerve Fiber Layer Examination
Retinal ganglion cell axons make up the RNFL and the optic nerve. RNFL loss has been shown to precede glaucomatous visual field loss in patients with elevated intraocular pressure [26]. Therefore, examination of the RNFL may play an important role in the diagnosis and management of glaucoma and should be part of an optic disc examination. In a healthy eye, the RNFL appears slightly opaque with radially oriented striations, which have been likened to “horsehair” [27]. Fibers of RNFL follow an arcuate pattern above and below the macula and are typically brighter along the superior and inferior arcuate bundles than the nasal and temporal bundles [27]. Although defects in the RNFL may be visualized ophthalmoscopically with white light, applying the red-free filter (green-appearing light) may enhance contrast and facilitate their identification. Photography of the RNFL follows the same principle. The use of high contrast film and a red-free filter improves the diagnostic yield.
Retinal Nerve Fiber Layer and Optic Disc Analyzers
Three main techniques are currently used for computerized optic nerve and retinal nerve fiber layer analysis. First, confocal scanning laser ophthalmoscopy (Heidelberg Retina Tomograph (HRT); Heidelberg Engineering, Heidelberg, Germany) generates up to 64 transaxial laser scans through the optic nerve head and peripapillary retina to reconstruct a high-resolution 3-dimensional image [28]. Second, scanning laser polarimetry (GDxVCC; Carl Zeiss Meditec, Dublin, CA, USA) measures peripapillary RNFL thickness by sending a laser beam to the posterior retina and assessing changes in the polarization of the reflected beam [28]. Third, optical coherence tomography (OCT; Carl Zeiss Meditec, Dublin, CA, USA) is an axial cross section of tissues based on the optical backscattering of low-coherence laser light (850 nm) as it passes through layers of different optical density [28]. Recent improvements in OCT technology called Fourier-domain OCT yield up to five times higher resolution with 60 times faster imaging speed than conventional time-domain OCT [29]. A new feature, the Enhanced Depth Imaging OCT, provides visualization of complex structures deep in the optic nerve such as lamina cribrosa, peripapillary choroid, sclera, and the vascular structures [9]. For example, Reis et al. using SD-OCT and photography, managed to measure the minimum rim width of Bruch’s membrane opening and found that it quantifies the neuroretinal rim from a true anatomical outer border [7].
In 2007, the American Academy of Ophthalmology published an Ophthalmic Technology Assessment that evaluated the literature on optic disc and RNFL analyzers. Based on evidence published from 2003 to 2006, the group concluded that these devices provide quantitative information as a complement to optic nerve and RNFL examination as well as other tests for glaucoma. Comparison of the studies was limited by differences in patient populations, definitions of glaucoma, and algorithms for detection. No single device was superior in distinguishing glaucoma patients from controls. Few studies addressed detection of glaucoma progression. All three machines were determined to be useful adjuncts in glaucoma care, with emphasis on familiarization with the device and placing the results in an appropriate perspective within the clinical context [28]. These technologies are constantly being updated, with respect to both hardware and software. It is expected that as digital imaging analyzers continue to improve, they may become the standard of care for optic nerve and RNFL examinations over the next decade.
Evaluating and Monitoring the Optic Disc
Practical application of information about the optic disc requires an accurate, precise, and reproducible way to document and follow its appearance. Characteristics of optic disc appearance from a clinical examination are recorded using drawings and disc photographs. Optic disc and RNFL digital image analyzers create printouts that may be compared over time.
Disc drawing is a quick, inexpensive, and valid method of following the optic nerve in patients with glaucoma. However, intraobserver and interobserver variability may limit the accuracy and precision of this method. Also, drawings of the optic disc based on examination through an undilated pupil often sacrifice detail and may be misleading. Regular drawings of the optic nerve based on findings of a dilated examination are important therefore in following patients by disc drawings. Several features of the optic nerve are systematically examined to formulate a composite picture of the optic nerve: (1) the shape of the outer margin of the optic disc, (2) the width of the neuroretinal rim as well as its color and shape, (3) the depth and dimension of the cup, (4) the peripapillary area, and (5) comparison of the discs between the two eyes. The optic nerve is typically drawn by sketching the cup as an inner circle within a larger circle representing the outer edge of the disc. The margins of both the outer edges of the disc may be difficult to discern, especially in cases with peripapillary atrophy. Also, the width of the neuroretinal rim may be unclear in cases of advanced rim loss and sloping (gradual incline) of the optic disc cup. Sloping may be represented by hash marks perpendicular to the drawn border of the neuroretinal rim or by multiple concentric lines of decreasing circumference. The relatively high magnification afforded by a direct ophthalmoscope may be helpful in identifying details of the optic nerve. Disc blood vessels may be good indicators of the border of the cup because they often travel along the superficial tissue of the optic disc. Therefore, blood vessels change direction at points of significant change in the contour of the optic disc cup. For example, at the juncture of the base of the optic disc cup and the face of its peripheral wall, blood vessels often bend abruptly. In addition, blood vessels bend sharply as they crest the wall of the optic disc cup, which is often a reliable marker of the neuroretinal rim margin. The cup is determined entirely by contour, and not by color. However, the color of the neuroretinal rim may be used to determine important information about the health of the optic nerve. The neuroretinal rim is supplied by a rich network of small blood vessels that give it an orange and/or pink color (Fig. 6.2). Loss of healthy neuroretinal rim, with its orange/pink color, and enlargement of optic disc cup make the optic disc look paler overall. However, an intact but pale neuroretinal rim indicates greater likelihood of non-glaucomatous optic neuropathy [30].
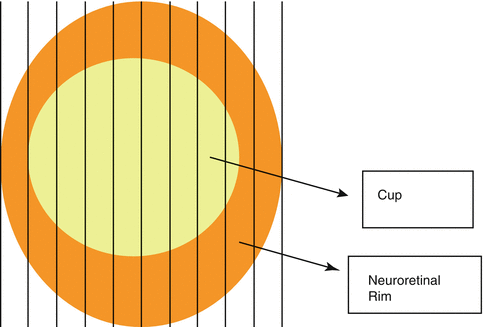
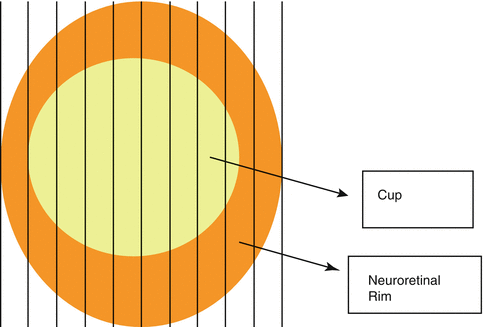
Fig. 6.2
Neuroretinal ring
There are different systems to classify the optic nerve appearance in a standardized manner. Armaly first mentioned the cup-to-disc ratio as a method of optic nerve head classification in 1969 [31]. After recognizing the margin of the disc and cup, the optic nerve is divided into tenths, and the cup is compared to the entire optic disc to obtain the cup-to-disc ratio, vertically and horizontally. Loss of the neuroretinal rim, with a corresponding increase in cupping, results in an increase in the cup-to-disc ratio. Because the optic disc is vertically oval and the optic cup is horizontally oval, the cup-to-disc ratios in normal eyes are larger horizontally than vertically. Less than 10 % of normal eyes have a smaller horizontal cup-to-disc ratio than their vertical cup-to-disc ratio [30, 32]. In early and moderate stages of glaucoma, rim loss typically occurs at the superior and inferior poles of the disc first. Thus, enlargement of the vertical cup-to-disc ratio rapidly overtakes enlargement of the horizontal cup-to-disc ratio [30].
Cup-to-disc ratios greater than 0.6 or cup-to-disc asymmetry greater than 0.2 between fellow eyes is suggestive of glaucoma [33]. However, interpretation of optic disc appearance depends on factors not specified in the cup-to-disc system. Notching of the neuroretinal rim, optic disc size, optic disc pits, intraretinal hemorrhage crossing the rim of the optic disc, rim loss, and localized pallor may all be signs of glaucomatous optic neuropathy. None of these signs are included in the cup-to-disc classification system. For example, two different optic discs may accurately be rated a 0.5 cup-to-disc ratio, though one contains loss of the neuroretinal rim, such as a notch, and one does not (see Fig. 6.3).
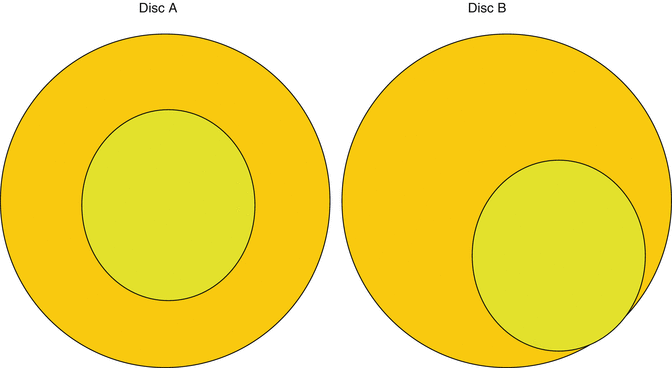
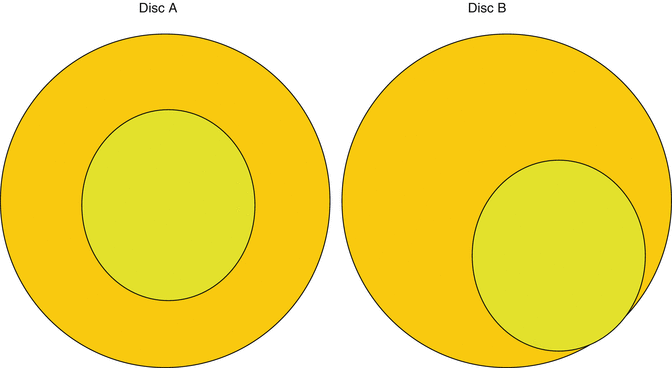
Fig. 6.3
Two optic nerves with cup-to-disc ratio of 0.5 – disc B has a notch with neuroretinal rim loss inferior temporally, while disc A does not
Since the cup-to-disc ratio may not reflect the true rim loss if there is a localized notch, the Disc Damage Likelihood Scale (DDLS) was designed in an attempt to provide a more accurate and reproducible method of defining glaucomatous optic nerve damage [26, 34]. In studies assessing DDLS and cup-to-disc ratio, from masked stereo photography, DDLS had excellent reproducibility and good interobserver reliability. DDLS also had greater inter- and intraobserver reliability than cup-to-disc ratio [35–37]. Briefly, the scale is based on the appearance of the neuroretinal rim of the optic disc, corrected for the disc diameter; gradations in the scale depend on the degree of rim loss rather than enlargement of the optic disc cup. Essentially, the examiner determines the smallest rim-to-disc ratio in any area of the nerve; the extent, if any, of circumference with no rim; and the size of the optic nerve. DDLS, compared to HRT and visual field loss, had a strong correlation which further emphasizes the need for precise neuroretinal rim evaluation [35]. The DDLS has ten stages, ranging from no damage to far-advanced damage.
Determining the DDLS: Step by Step
Step 1. Perform dilation, if necessary. The pupils must be sufficiently enlarged to allow a clear view of the fundus.
Step 2. Obtain a measurement of the vertical size of the patient’s optic nerve via a slit-lamp biomicroscope that indicates the size in millimeters. Multiply this figure by 0.9 for a +60-D lens, by 1 for a +66 D, or by 1.3 for a +90-D lens (Table 6.2) [37].
Table 6.2
Indirect lens correction factors account for the influence of magnification
Indirect lens corrective factors
Lens type
Diopter
Correction factor
Volk
60
0.88
66
1.0
78
1.2
90
1.3
Nikon
60
1.03
90
1.63
Step 3. Examine the optic disc for an area where its outer edge is clearly distinguished from other ocular tissue such as sclera and determine the full circumference of the outer edge.
Step 4. Define the inside edge of the neuroretinal rim (outer edge of the cup). To obtain an estimate of the rim-to-disc ratio, compare the width of the neuroretinal rim with that of the disc diameter on the same axis. Repeat this comparison using several clock positions. If the rim-to-disc ratio is different at various parts of the rim, find the narrowest section of the rim and calculate the rim-to-disc ratio at that point.
Step 5. Draw the shape of the optic disc. When sketching the neuroretinal rim’s inner edge, indicate areas of clear demarcation by drawing a thick line and those of less clear demarcation with thin or hatched lines. Make a note of the course of the blood vessels that help determine the rim’s width and any pertinent features of the disc, such as notches, pallor, and hemorrhage.
Step 6. To determine the DDLS, use your disc drawing, the narrowest rim-to-disc ratio, the disc size, and compare to the DDLS nomogram (Fig. 6.4). If the nerve is smaller or larger than average, adjust the DDLS score appropriately. An easy adjustment method is to stage the nerve as if it were of average size and then increase the stage by one if the nerve is small or decrease the stage by one if it is large.
Fig. 6.4
The DDLS is based on the radial width of the neuroretinal rim measured at its narrowest point. The unit of measurement is the rim-to-disc ratio. The circumferential extent of absent rim (zero rim-to-disc ratio) is measured in degrees (Reprinted from Spaeth [74]. With permission from Bryn Mawr Publishing)
DDLS scores of 1–3 are rarely associated with glaucomatous visual field loss. However, any disc graded stage 5 or higher will almost always be pathological, although it may not be glaucomatous.
For Caucasians without high myopia, mean optic disc area can range from 2.1 mm2 to about 2.8 mm2 in different studies. In healthy subjects, the size range for a normal optic nerve head is between 1.3 and 2.7 mm in diameter, with 1.9 mm considered to be average.
Important Optic Nerve Characteristics
Optic Disc Size
The cup-to-disc ratio does not account for differences in optic disc size. Most studies agree that the average disc diameter is 1.85–1.95 mm (range 0.95–2.9 mm) vertically and 1.7–1.8 mm (range 0.9–2.6 mm) horizontally [8]. There is tremendous variation in disc size within the normal population. On average, Caucasians have relatively small optic discs, with increasing size seen in Mexicans, Asians, and African-Americans [38]. Although the influence of optic disc area on risk of glaucoma is controversial, mathematically, the larger the optic disc area, the larger the neuroretinal rim area, despite a consistent cup-to-disc ratio. Conversely, smaller optic disc area confers smaller neuroretinal rim area despite consistent cup-to-disc ratio. This seems to indicate that vision loss from glaucoma in large optic nerves would require more rim loss than in small optic nerves (Fig. 6.5). Also, large optic nerves have a larger cup volume than small optic nerves despite proportional cup-to-disc ratios. This characteristic draws attention and sometimes predisposes physiologically cupped large optic nerves to an erroneous diagnosis of glaucoma. The converse may also be true. Small optic nerves with proportionately small cups may be underappreciated. Finally, optic disc size is correlated with refractive error. Eyes with greater than 8 D of myopia have increasing optic disc area and those with more than 4 D of hyperopia have decreasing disc area. Between −8 and +4 D of refractive error, optic disc size varies independent of refractive error [39].
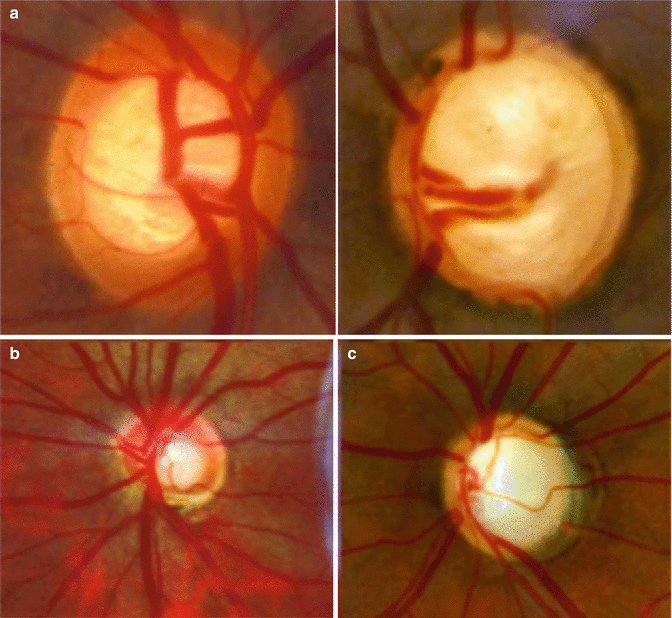
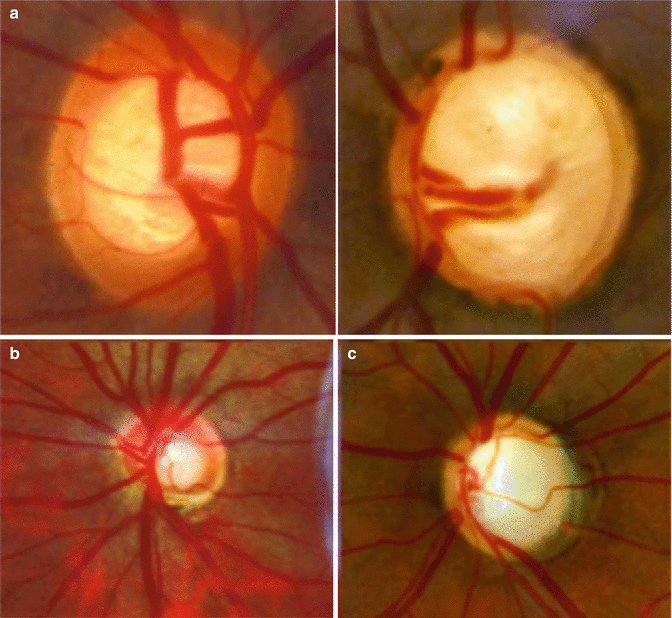
Fig. 6.5
Optic nerves. (a) This illustrates large healthy discs with large cup-to-disc ratios from patients who have been followed for more than 30 years with no disc change or any other sign of glaucoma. (b) Shows a small glaucomatous optic disc without a large cup-to-disc ratio but with an eccentric cup exhibiting an inferior focal notch associated with marked visual field loss. (c) Depicts a large disc with glaucomatous cupping (Reprinted with permission from the author – Zangalli et al. [75]. With permission from Elsevier)
Documenting overall appearance of the optic nerve is of great importance as it may confirm other pathogenesis (Table 6.1) [40–45]. Deokule et al. demonstrated that untreated glaucoma has higher mean IOP and more IOP spikes nocturnally with concentric optic disc enlargement than in patients with a nonconcentric optic disc. Myopic eyes with a tilted appearance and temporal crescent (“β-zone parapapillary atrophy”) challenge the examination [46, 47]. This further instills the necessity of a thorough posterior examination to document progressive tilting of the optic nerve and enlargement of any parapapillary atrophy.
Neuroretinal Rim Size and Shape
The neuroretinal rim width is the distance between the borders of the optic disc delimited by the scleral ring and the inner edge of the rim as it turns posteriorly to create the cup. Neuroretinal rim loss is critically important because it correlates well with loss of visual function [48]. Also, loss of the neuroretinal rim is helpful in separating glaucoma patients from normals as well as ocular hypertensives [48, 49]. The wide range in size of the optic disc cup in normal populations may make cupping less valuable for diagnosis of glaucoma than neuroretinal rim loss [38]. Particularly in patients with focal neuroretinal rim loss, the location of the visual field defect should easily correspond to the location of rim loss. For example, given the arcuate nature of RNFL axon bundles as they stream to the vertical poles of the optic disc, loss of superior and inferior neuroretinal rim will match an inferior or superior arcuate visual field defect, respectively. When focal neuroretinal rim loss adopts a crescent-shaped configuration, it may be termed a notch. Typically, notches are circular or oval in appearance, but with a steeper curvature than the larger internal edge of the neuroretinal rim [1] (Figs. 6.6 and 6.7). Focal damage tends to accompany quicker visual field progression and faster deterioration of the optic nerve [50]. Diffuse appearance of the optic nerve is associated with the slowest rate of change in visual field, and sclerotic optic nerve damage has the slowest rate of change on the optic nerve. Spaeth et al. recommended descriptions of the loss of neuroretinal rim be changed to “narrowing” (narrow, wide, widening) when referring to the plane parallel to the surface of the optic disc (nasal–temporal plane). When referring to the distance between anterior surface of the disc and lamina cribrosa, the depth should be referred to as thin, thinning, thick, or thickening [51].
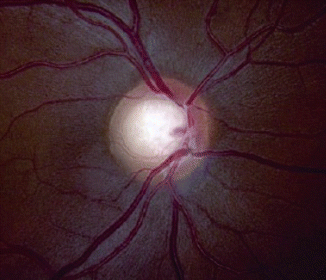
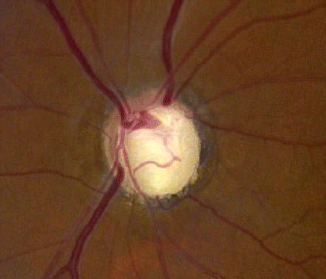
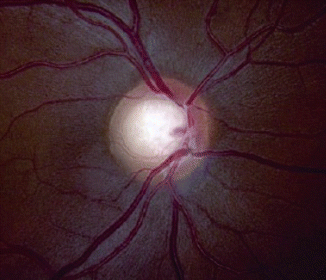
Fig. 6.6
Glaucomatous optic neuropathy with intact but thin neuroretinal rim
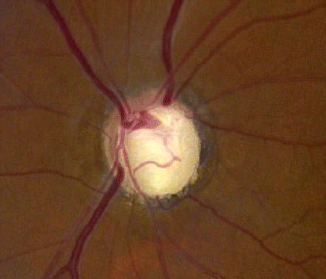
Fig. 6.7
Glaucomatous optic neuropathy with significant neuroretinal rim loss
Neuroretinal Rim Pallor
Pallor of the neuroretinal rim increases the likelihood that a non-glaucomatous optic neuropathy is present, especially when the pallor is more prominent than the cupping [34]. In 1980, Trobe et al. published a study in which three experienced ophthalmologists evaluated stereoscopic optic disc photographs of “unknowns” with glaucomatous and non-glaucomatous optic neuropathy. They found that neuroretinal rim pallor was 94 % specific for non-glaucomatous atrophy, while focal or diffuse obliteration of the neuroretinal rim was 87 % specific for glaucoma. Thinning of the rim was more common in glaucoma than in non-glaucomatous damage, but was only 47 % specific for glaucoma [52]. Therefore, both neuroretinal rim pallor and neuroretinal rim thinning should be placed into perspective with other findings when separating non-glaucomatous from glaucomatous optic neuropathy.
Disc Asymmetry
Asymmetry of optic disc cupping between fellow eyes may be an indicator of unilateral glaucoma, asymmetric but bilateral glaucoma, or non-glaucomatous optic neuropathy.
In general, the cup-to-disc ratio in a normal subject will not differ between fellow eyes by more than 0.2 [53]. However, data regarding disc size, cup-to-disc ratio, and degree of asymmetry is varied among published glaucoma surveys in different populations.
Optic Disc Hemorrhages
Retinal nerve fiber layer hemorrhages at the disc margin have been associated with increased risk of glaucoma progression, especially in average pressure glaucoma [54, 55]. Disc hemorrhages are detected in about 4–7 % of eyes with glaucoma [56, 57]. Disc hemorrhages are more likely to be found in females and older patients [58]. They tend to increase during early to moderately advanced stages of glaucoma and then decrease in frequency toward end-stage glaucoma [59]. Usually, they extend from the disc tissue across the disc margin. They may overlie an adjacent peripapillary zone of choroidal or retinal pigment epithelium atrophy but have at least one end touching the disc margin. Disc hemorrhages may be small and located near blood vessels, making detection difficult. They typically occur in areas of localized RNFL defects and notches of the neuroretinal rim [30]. Reports indicate that disc hemorrhages rarely occur in conditions other than glaucoma, 0.6–1.4 % in the normal population [55, 57]. Therefore, although they are poorly sensitive, disc hemorrhages are highly specific for glaucoma [30, 59]. Generally, hemorrhages are transient and visible only for days to months, but they may recur. Recurrent disc hemorrhages may suggest faster progression in optic disc changes and in defects of the RNFL [57, 60–63]. Concurrent retinal changes may help make non-glaucomatous etiology of disc hemorrhage obvious.
The incidence of optic disc hemorrhages, among patients with bilateral chronic open-angle glaucoma, is not associated with marked differences in the structure of optic nerve head such as size of the optic disc and neuroretinal rim and occurrence of parapapillary atrophy [59]. Diabetic retinopathy, retinal vein occlusion, and papillitis may occur with disc hemorrhage. Acute posterior vitreous detachment may cause disc hemorrhage as well (Fig. 6.8).
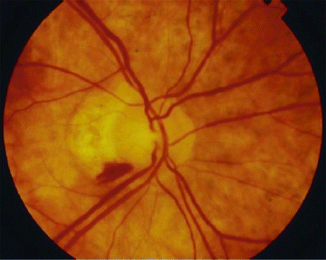
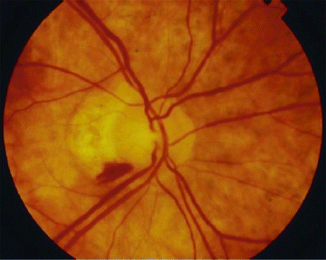
Fig. 6.8
Nerve fiber layer hemorrhage along inferior temporal optic disc margin
Peripapillary Chorioretinal Atrophy
Peripapillary atrophy (PPA) refers to the thinning, misalignment, irregularity, and degeneration of the retinal pigment epithelium, choriocapillaris, choroid, and sclera just outside of the optic disc. PPA has an association with the development and progression of glaucoma [34]. Among ocular hypertensives, studies have shown that 50–75 % of patients who converted to glaucoma also experienced progression of peripapillary atrophy. Among those patients who did not develop glaucoma, 10–14 % experienced progressive PPA [64, 65]. PPA may also be a congenital anomaly in which the neurosensory retina terminates short of the optic disc [1]. Ophthalmoscopically, the regions of peripapillary chorioretinal atrophy may be divided into a peripheral zone (alpha zone) and a central zone (beta zone) [30]. Bordered by retina peripherally and sclera centrally, zone alpha is characterized by irregular hyper- and hypopigmentation indicative of chorioretinal thinning. Choroidal vessels and sclera may be clearly visible in zone alpha. Zone beta is marked by significant atrophy of the retinal pigment epithelium and choriocapillaris, with more clear visibility of the choroidal vessels and sclera than in the alpha zone. If both alpha and beta zones are present, the beta zone is always closer to the optic disc than the alpha zone [4]. Some authors suggest that disc damage is more likely in areas adjacent to PPA. Roberts et al. demonstrated that SD-OCT detected 25 to 30 % thinner peripapillary choroid in glaucoma patients with sclerotic optic disc damage than in patients with focal or diffuse optic disc damage and in healthy controls [66]. However, PPA occurs to a variable degree in normal eyes and may not be directly related to glaucoma [67] (Fig. 6.9, Sidebar 6.1).
