Figure 1–1. A. Cartilages of the larynx. B. Schematic representation of position changes of laryngeal cartilages illustrating the most extreme positions achieved by each. (Reproduced with permission from Pernkopf.1)
The perichondrium of the thyroid cartilage is thinner internally than externally. Externally, in the mid-line, there is a tiny landmark that can be helpful in identifying the position of the anterior commissure.6 This small, diamond-shaped surface depression is associated with a slightly lighter color compared to the adjacent thyroid cartilage. It is found in the anterior midline, approximately halfway between the thyroid notch and the inferior border of the thyroid cartilage, and a small, unnamed artery travels through this tiny depression, as described by Adams et al.6 The landmark is referred to sometimes as Montgomery’s aperture. At a corresponding location on the inner surface, there is a small protrusion that is devoid of perichondrium. This is the point of attachment of Broyles ligament and the anterior commissure tendon. The rest of the thyroid cartilage is covered with fairly thick perichondrium, and the smooth, concave inner surface is covered by a mucosal membrane. Broyles ligament is formed by the vocal ligament (which is also the upper border of the conus elasticus), the internal perichondrium of the thyroid cartilage, and the thyroepiglottic ligament.
The oblique line is another important external landmark of the thyroid cartilage. It runs anteroinferiorly from a superior thyroid tubercle located just inferior to the superior cornu, and it extends to the inferior thyroid tubercle located at the lower border of the thyroid lamina. The oblique line is actually a ridge to which the thyrohyoid, sternothyroid, and inferior pharyngeal constrictor muscles attach. Fibers from the palatopharyngeus and stylopharyngeus muscles attach to the posterior border of the thyroid cartilage.
The signet ring–shaped cricoid cartilage is the only circumferential cartilaginous structure in the airway. Its posterior lamina may rise to a height of approximately 30 millimeters (mm), and its anterior arch may be only a few millimeters in height. Not only is the anterior aspect of the arch thin, but it also ossifies later than the posterior aspect of the cricoid cartilage, which begins to ossify in the early to mid-20s. Because the anterior portion of the arch is both thin and tends to ossify later, it is particularly prone to fracture during surgical manipulation. This should be remembered in procedures such as cricothyroid approximation; traction should always be centered laterally on the cricoid arch, rather than near the midline. The cricoid and thyroid cartilages are joined through the cricothyroid joints. These synovial joints vary among individuals. They have been divided into 3 groups.7 In group 1, the rotation axis of the cricothyroid joint is located in the lower third of the joint (13 of 24 specimens studied); in group 2, it is located in the middle third of the joint (5/23); and in group 3 the effective axis of rotation is located in the lower third of the cricoid cartilage. Elongations of the vocal fold were 12% in group 1, 8% in group 2, and 3% in group 3. These differences may be important for patients undergoing cricothyroid approximation surgery, but more research is needed to confirm these findings and investigate their clinical implications. The cricoid is connected to the thyroid cartilage not only through the cricothyroid joints, but also through the cricothyroid membrane and its midline thickening known as the cricothyroid ligament.
Internal dimensions of the cricoid cartilage and trachea vary substantially. Such information is important with regard to tracheal intubation, dilatation, stenting, endoscopy, anastomosis, and transplantation. The luminal cross sections vary between and among men and women. The smallest dimension occurs in the frontal plane.8 In women, this measures approximately 11.6 mm, with a range of 8.9 to 17 mm. In men, it is about 15 mm, with a range of 11 to 21.5 mm. The distance between the cricoarytenoid joint facets varies from person to person, as well, as does the angle between longitudinal axes of the cricoarytenoid joint facets (42°–74° in women, 37°–75° in men).8 Morphometric characteristics of the larynx have also been studied by Jotz et al.9 They examined larynges of 50 male and 50 female fresh cadavers of humans older than 40 years. All laryngeal measurements were greater in men than in women except for the thyroid angle that was greater in women. There was no significant difference in morphological comparison between men and women among various age groups.
The cross section of the trachea is also highly variable, with a frontal diameter reported as narrow as 9.9 mm in women and 12 mm in men.8 The marked variation in size and shape highlights the difficulty in creating a standardized rigid stent. It should also be noted that the diameter of the cricoid ring in some women is too narrow to permit the atraumatic passage of an endotracheal tube with a 7-mm internal diameter. Anatomic variation also must be taken into consideration during laryngotracheal replacement or transplantation.
In addition to the cricoid, thyroid, and paired arytenoid cartilages, there are numerous other components of the laryngeal skeleton and the related structures. The superior aspect of the laryngeal skeleton is the hyoid bone, which is usually ossified by age 2. The hyoid bone attaches to the mylohyoid, geniohyoid, and hyoglossus muscles superiorly and inferiorly connects to the thyroid cartilage via the thyrohyoid membrane. This U-shaped bone has an inferiorly located lesser cornu and a superiorly located greater cornu on each side.
The epiglottis is a fibroelastic cartilage that is shaped like a leaf and narrows inferiorly where it becomes the petiole. The petiole attaches to the inner surface of the thyroid cartilage immediately below the thyroid notch by the thyroepiglottic ligament. The superior aspect of the epiglottis faces the base of the tongue anteriorly and the laryngeal inlet posteriorly. The hyoepiglottic ligament connects the posterior surface of the hyoid bone to the lingual surface of the epiglottis. On its laryngeal surface, the epiglottis contains a protuberance that sometimes obscures view of the anterior commissure. This is the epiglottic tubercle. Perichondrium is less densely adherent to the epiglottic cartilage on the lingual surface than on the laryngeal surface, explaining why epiglottic edema tends to be more prominent in the vallecula than in the laryngeal inlet. However, edema on the lingual surface can push the epiglottis posteriorly, resulting in airway obstruction. The preepiglottic space is formed by the mucosa of the vallecula superiorly, the thyroid cartilage and thyrohyoid membrane anteriorly, and the epiglottis posteriorly and inferiorly. Blood vessels and lymphatic channels course through this space.
There are several cartilages of less functional importance located above the thyroid cartilage. The cartilages of Santorini, or corniculate cartilages, are fibroelastic and are found above the arytenoid cartilages. They help improve the rigidity of the aryepiglottic folds. Like the epiglottis and many other elastic cartilages, they do not ossify. The cuneiform cartilages (cartilages of Wrisberg) also do not ossify, even though they consist of hyaline cartilage. They are located in the aryepiglottic folds and also improve rigidity, helping to direct swallowing toward the piriform sinuses. The triticeal cartilages are located laterally within the thyrohyoid ligaments. These structures are hyaline cartilages and often do ossify (as may the lateral thyrohyoid ligaments themselves). They may easily be mistaken on x-rays for foreign bodies. The lateral thyrohyoid ligaments are actually thickenings of the thyrohyoid membrane. There is also more central thickening called the medial thyrohyoid ligament. The laryngeal vessels and the internal branches of the superior laryngeal nerves enter the thyrohyoid membrane posterior to the lateral thyrohyoid ligaments. The thyrohyoid ligaments and membranes are among the structures that suspend the larynx directly or indirectly from the skull base. The other structures that do so include the stylohyoid ligaments, the thyrohyoid ligaments and membrane, the thyroepiglottic ligaments, the cricothyroid ligaments and membrane, the cricoarytenoid ligaments, and the cricotracheal ligament and membrane.
The arytenoid cartilages are capable of complex motion. Previously, it was believed that the arytenoids rock, glide, and rotate. More accurately, the cartilages are brought together in the midline and revolve over the cricoid. It appears as if individuals use different strategies for approximating the arytenoids, and these strategies may influence a person’s susceptibility to laryngeal trauma that can cause vocal process ulcers and laryngeal granulomas.
The larynx contains 2 important, large, paired “membranes,” the triangular membranes and the quadrangular membranes (Figure 1–2). The paired triangular membranes form the conus elasticus. Each triangular membrane is attached to the cricoid and thyroid cartilages anteriorly (the base of the triangular membrane), to the cricoid cartilage inferiorly, and to the vocal process of the arytenoid cartilage posteriorly (the apex of the triangular membrane). The superior edge of each fibroelastic triangular membrane is the vocal ligament, forming the intermediate and deep layers of lamina propria of the vocal folds, as discussed below. These structures extend anteriorly to form a portion of Broyles ligament. More anteriorly, a portion of the conus elasticus constitutes the cricothyroid ligament.
Like the upper border of the triangular membrane, the upper and lower borders of the quadrangular membrane are free edges. The upper border of each quadrangular membrane is the aryepiglottic fold, bilaterally. The lower border extends from the inferior aspect of the epiglottis to the vocal process of the arytenoid cartilages and forms part of the vestibular (or ventricular) fold, or false vocal fold. Superior and inferior thickenings in the quadrangular membrane form the aryepiglottic ligament and the vestibular ligament, respectively. The quadrangular membrane is shorter in vertical height posteriorly than anteriorly. Lateral to these structures is a region called the paraglottic space. It is bounded laterally by the thyroid lamina and medially by the supraglottic mucosa covering the vestibular fold from the ventricle to the aryepiglottic fold. There is a thin, elastic membrane that is contiguous above with the quadrangular membrane and below with the conus elasticus, forming an intermediate segment of elastic tissue that encloses the laryngeal ventricle. The paraglottic space is contiguous with the space between the cricoid and thyroid cartilages. The laryngeal inlet lies between the aryepiglottic folds.
Figure 1–2. Internal view of the larynx illustrating the position of the quadrangular and triangular membranes. (Reproduced with permission from Pernkopf.1)
The region formed by the paired ventricular and aryepiglottic folds is designated as “supraglottis.” A pouch of mucosa between the under surface of the false vocal folds and the upper surface of the true vocal folds is called the ventricle of Morgagni, or the laryngeal ventricle. The superior aspect of the laryngeal ventricle is known as the saccule of Hilton. The supraglottis extends from the tip of the epiglottis to the junction between the floor and lateral wall of the laryngeal ventricle. Hence, most clinicians define the floor of the ventricle as part of the glottic larynx. A detailed understanding of the supraglottic larynx is becoming more important clinically because of advances in transoral robotic surgery (TORS), as illustrated by Goyal et al who described anatomic variations in the superior laryngeal neurovascular bundle and differences in anatomic perspective using a robot.10 The glottic larynx also includes the true vocal folds, anterior commissure, and interarytenoid region at the level of the vocal folds posteriorly (commonly, and incorrectly, referred to as the posterior commissure). The subglottis begins at the junction of squamous and respiratory epithelium under the vibratory margin of the vocal folds, about 5 mm below the beginning of the vibratory margin. The subglottis ends at the inferior border of the cricoid cartilage.
Larynx: Mucosa
With the exception of the vocal folds, the epithelial lining of most of the vocal tract is pseudostratified, ciliated columnar epithelium, typical respiratory epithelium involved in handling mucous secretions. The vibratory margin of the vocal fold is covered with nonkeratinizing, stratified squamous epithelium, better suited than respiratory epithelium to withstand the trauma of vocal fold contact. Vocal fold lubrication is created by cells in several areas. The saccule, the posterior surface of the epiglottis, and the aryepiglottic folds contain seromucinous, tubuloalveolar glands that secrete serous and/or mucinous lubricant. There are also goblet cells within the respiratory epithelium that secrete mucus. These are especially common in the area of the false vocal folds. The goblet cells and glands also secrete glycoproteins, lysozymes, and other materials essential to healthy vocal fold function. The laryngeal mucosa also contains immunologically active Langerhans cells.11 In most people, secretory glands are not located near the vibratory margin.
The vibratory margin of the vocal folds is much more complicated than simply mucosa overlying muscle. It consists of 5 layers (Figure 1–3).15 The thin, lubricated epithelium covering the vocal folds forms the area of contact between the vibrating vocal folds and acts somewhat like a capsule, helping to maintain vocal fold shape. The superficial layer of the lamina propria, also known as Reinke space, is composed of loose fibrous components and matrix and lies immediately below the epithelial layer. It contains very few fibroblasts and consists of a network of mucopolysaccharides, hyaluronic acid, and decorin that provide for the flexibility required of the vocal fold cover layer.16–19 In normal vocal folds the superficial lamina propria also contains fibronectin. Fibronectin is also thought to be deposited as a response to tissue injury.20 The superficial lamina propria also contains elastin precursors (elaunin and oxytalin), but relatively few mature elastin or collagen fibers. Ordinarily, it has few or no lymphatics, secretory glands, or capillaries. Myofibroblasts and macrophages were found in the superficial lamina propria in about one-third of laryngeal specimens studied by Catten et al19 and were more common in women than in men. The third layer of the vocal folds is the intermediate layer of the lamina propria. Mature elastin fibers make up most of the intermediate layer. They are arranged longitudinally. This layer also contains large quantities of hyaluronic acid, a relatively inflexible space filler that is hydrophilic and is believed to act as a shock absorber.17 Fibromodulin also is present in the intermediate layer.18 The deep layer of the lamina propria, the fourth layer, is composed primarily of longitudinally arranged collagenous fibers and is rich in fibroblasts. De Melo et al analyzed the distribution of collagen in the lamina propria.21 They described a layer of thick collagen type 1 immediately below the epithelium, and a second, more dense layer superficial to the thyroarytenoid muscle and penetrating between muscle fibers. A layer of collagen type 3 was located between the 2 collagen type 1 layers. The collagen fibers formed an intertwined “wicker basket” network within the lamina propria which helps explain how the vocal fold is able to stretch, despite containing nonstretchable fibers. Interestingly, the authors also noted segmental areas of disarray in the intertwining collagen layers in older patients, suggesting that the variation in histoarchitecture might be related to voice changes common among elderly patients. Prades et al reported similar findings and noted that the mean thickness of the superficial layer of lamina propria is about 13% of the total lamina propria.22 The intermediate layer constitutes about 51%, and the deep layer makes up about 36% of the lamina propria. The TA or vocalis muscle constitutes the body of the vocal fold and is the fifth layer, as well as one of the intrinsic laryngeal muscles. The region that consists of the intermediate and deep layers of the lamina propria is called the vocal ligament and lies immediately below Reinke space. It is important to note that this layered structure of the vocal folds is not present at birth, but rather begins developing at around the age of 7 or 8 years and is not completed until the end of adolescence.23,24 There are other differences between the pediatric and adult larynx that are not reviewed in this chapter, but many are discussed elsewhere in this book in connection with relevant clinical entities. A great deal more information is available about the ultrastructure of human vocal folds and may be found in other literature.25
Figure 1–3. A. An overview of the larynx and vocal tract, showing the vocal folds, and the region from which the vocal fold was sampled to obtain the cross section showing the layered structure. (Reprinted with permission from Sataloff.12) B. The structure of the vocal fold. (Reprinted with permission from Hirano.13(p5)) C. Schematic representation of a horizontal section of the vocal fold. TC, thyroid cartilage; ACT, anterior commissure tendon; AMF, anterior macula flava; PMF, posterior macula flava; AC, arytenoid cartilage; E, epithelium; LP, lamina propria; s, superficial layer; i, intermediate layer; d, deep layer; VOC, vocalis muscle. (Reproduced with permission from Gray et al.14)
Although variations along the length of the membranous vocal fold are important in only a few situations, the surgeon, in particular, should be aware that they exist. The mucosa (epithelium and lamina propria, together) of the normal adult male has been described as being approximately 1.1-mm thick. The superficial layer is about 0.3 mm, the vocal ligament is about 0.8 mm,26 and the epithelium is about 5–25 cells (about 50 microns) in thickness.27 However, particularly interesting research by Friedrich et al shows additional interesting complexity and variation in the anatomy of the vocal fold.28 Friedrich et al divided the vocal fold into 5 histological and functional portions. Three of the portions included the musculomembranous vocal fold, and the other 2 regions were divisions of the posterior glottic region and included the region of the vocal process of the arytenoids, which constitutes the cartilaginous portion of the vocal folds and the lateral wall of the posterior glottis. They also found significant differences between men and women in terms of not only absolute measurements, but also relative dimensions of various portions of the vocal fold. In particular, the middle portion of the musculomembranous vocal fold was twice as long in men (8.5 mm) as in women (4.6 mm), accounting for 37% of total glottic length in men and only 29% in women. The authors speculate that this difference in the length of the vibrating portions of the vocal folds may explain why the fundamental frequency ratio between men and women is approximately 1:2, while the overall laryngeal dimensions are only 1:1.5. In addition to variations in length, Friedrich et al also found that the thickness of the lamina propria varied depending on location along the length of the vocal fold and on gender (Figure 1–4). Friedrich’s elegant research should be interpreted in the historical context of earlier observations, some of which appear to provide data that are contradictory to his. Actually, the methods of observation and intent of the studies differed; most of the findings in the various studies are reconcilable.
The vocal folds join anteriorly. The area of union is known as the anterior commissure. A “commissure” is a point of coming together. Hence, the term posterior commissure has been discouraged, because the vocal folds do not come together in posterior larynx. However, this terminology has been challenged by Tucker and Tucker,29 who argue that histologic data showing the posterior union the cricoid cartilage justify the use of the term posterior commissure. Their view has not been accepted widely, and most laryngologists still agree with Hirano et al30 and refer to “the posterior glottis” or “the posterior larynx,” rather than the posterior commissure. In 1986, Hirano et al30 studied the posterior glottis in 20 specimens obtained from autopsy, photographing the larynges from above and below in neutral, adducted, and abducted conditions. They also studied the histology of the posterior glottis in the same 3 conditions. Hirano et al defined the posterior glottis as consisting of 3 portions: the posterior wall of the glottis, the lateral wall of the posterior glottis, and the cartilaginous portion of the vocal fold. They noted that the posterior larynx closes at the supraglottis during vocal fold adduction, rather than at the glottis, thus producing a conical space that can be seen only from below. Using this methodology, they calculated that the posterior glottis accounted for 35%–45% of the entire glottic length, and 50%–65% of the entire glottic area. The mucosa covering the posterior glottis consisted of ciliated epithelium, and the lamina propria consisted of only 2 layers. The superficial layer was looser in structure, and the deep layer was composed of dense elastic and collagenous fibers with numerous mucosecretory glands. Many of the fibers in the deep layer ran vertically in the region of the posterior wall of the glottis, but the fibers on the lateral wall were found to run obliquely. This study provided useful, interesting anatomic information. However, in interpreting its physiological significance, it should be remembered that abduction and adduction were accomplished by threads attached to the muscular processes of the arytenoid cartilages; the results may or may not be identical to the complex motions that occur during phonation in a living human.30
Figure 1–4. A. Vocal fold divided into zones. B. Proportions of the vocal fold constituted by each zone in men. C. Thickness of the lamina propria in millimeters in women. Note that the European numbering system is used in which 0.45 mm is written as 0,45 mm. (Courtesy of Gerhardt Friederich, MD.)
It has long been recognized that particularly striking variations occur at the anterior and posterior portions of the musculomembranous vocal fold, the region between the vocal process and attachment of the vocal fold to the thyroid cartilage.31 Anteriorly, the intermediate layer of the lamina propria becomes thick, forming an oval mass called the anterior macula flava. This structure is composed of stroma, fibroblasts, and elastic fibers. Anteriorly, the anterior macula flava inserts into the anterior commissure tendon (Broyles ligament). The anterior commissure tendon is a mass of collagenous fibers, which is connected to the midpoint of the thyroid cartilage anteriorly, the anterior macula flava posteriorly, and the deep layer of the lamina propria laterally. As Hirano has pointed out, this arrangement allows the stiffness to change gradually from the pliable musculomembranous vocal fold to the stiffer thyroid cartilage.31 Sato et al have described stellate cells in the macula flava that are related to fibroblasts, but which appear to constantly synthesize extracellular matrices that are required for normal human vocal fold mucosal function.32–34 Changes in extracellular matrices alter vocal fold function, particularly viscoelasticity, and these changes are associated with some aspects of vocal aging. Aging changes in the vocal fold stellate cells in the macula flava may be responsible for some of the age-related extracellular matrices alterations.35–38 Sato also demonstrated that stellate cells of the macula flava were more sensitive to radiation-induced change than many of the other components of vocal folds.39 These findings suggest that radiation may damage the ability of stellate cells to generate precursors of collagenous and elastic fibers, indicating that radiation causes changes not only in fiberblasts and other components of the lamina propria, but also in the ability of the stellate cells in the macula flava to maintain normal vocal fold homeostasis. Fuja et al cultured vocal fold stellate cells and demonstrated induced deactivation.40 Their data suggest that the stellate cells are a potential target for research on the physical elastic properties of vocal fold mucosa during normal phonation, aging, scar, fibrosis, and other conditions.
A similar gradual change in stiffness occurs posteriorly where the intermediate layer of the lamina propria also thickens to form the posterior macula flava, another oval mass that is structurally similar to the anterior macula flava. The posterior macula flava attaches to the vocal process of the arytenoid cartilage through a transitional structure that consists of chondrocytes, fibroblasts, and intermediate cells.41 Thus, the stiffness progresses from the flexible musculomembranous vocal fold to the slightly stiffer macula flava, to the stiffer transitional structure, to the elastic cartilage of the vocal process, and to the hyalin cartilage of the arytenoid body. It is believed that this gradual change in stiffness serves as a cushion that may protect the vocal folds from mechanical damage caused by contact or vibrations.41 It may also act as a controlled damper that smooths mechanical changes during vocal fold movements. This arrangement seems particularly well suited to vibration, as are other aspects of the vocal fold architecture. For example, blood vessels in the vocal folds begin posteriorly and anteriorly and run parallel to the vibratory margin, with very few vessels entering the mucosa perpendicular to the free edge of the vibratory margin or from the underlying muscle. Even the elastic and collagenous fibers of the lamina propria run approximately parallel to the vibratory margin, allowing them to compress against each other or pull apart from each other flexibly and parallel with the forces of the mucosal wave. The more one studies the vocal fold, the more one appreciates the beauty of its engineering.
Functionally, the 5 layers have different mechanical properties and are analogous to ball bearings of different sizes that allow the smooth shearing action necessary for proper vocal fold vibration. The posterior two-fifths (approximately) of the vocal folds are cartilaginous, and the anterior three-fifths are musculomembranous (from the vocal process forward) in adults. Under normal circumstances, most of the vibratory function critical to voice quality occurs in the musculomembranous portion.
Mechanically, the vocal fold structures act more like 3 layers consisting of the cover (epithelium and superficial layer of the lamina propria), transition (intermediate and deep layers of the lamina propria), and the body (the vocalis muscles). Understanding this anatomy is important because different pathologic entities occur in different layers and require different approaches to treatment. For example, fibroblasts are responsible for scar formation. Therefore, lesions that occur superficially in the vocal folds (eg, nodules, cysts, and most polyps) should permit treatment without disturbance of the intermediate and deep layers, where fibroblast proliferation, or scar formation, occurs.
In addition to the 5 layers of the vocal fold, there is a complex basement membrane connecting the epithelium to the superficial layer of the lamina propria.42 The basement membrane is a multilayered, chemically complex structure. It gives rise to type VII collagen loops that encircle type III collagen fibers in the superficial layer of the lamina propria (Figure 1–5). Current research has changed substantially our understanding of vocal fold composition and function. For example, in his description of the basement membrane zone, Gray described a chain link–fence arrangement of anchoring fibers, which he believes permits tissue compression and bending.16 The density of the anchoring fibers is greatest in the area of greatest vibration and shearing stresses, in the middle of the musculomembranous portion of the vocal folds. Knowledge of the basement membrane has already been important in changing surgical techniques, as discussed later in this book. It also appears important in other matters, such as the ability to heal following trauma, the development of certain kinds of vocal fold pathology, and in histopathologic differential diagnosis.
Figure 1–5. A. Structure of the vocal fold (not drawn to scale). The basement membrane lies between the epithelium and the superficial layer of the lamina propria. (Reproduced with permission from Gray,42) B. Basement membrane zone. Basal cells are connected to the lamina densa by attachment plax (AP) in the plasma membrane of the epidermis. Anchoring filaments (AFL) extend from the attachment plax through the subbasal densa plate (DP) and attach to the lamina densa (dark single-layer, electron-dense band just beneath the basal cell layer.) The subbasement membrane zone consists of anchoring fibers (AFs) that attach to the lamina densa and extend into the superficial layer of the lamina propria. Type VII collagen fibers attach to the network of the lamina propria by looping around type III collagen fibers. (Reproduced with permission from Gray.42) C. Type VII collagen anchoring fibers pass from the basement membrane, reinserting into it. Through the anchoring fiber loops pass type III collagen fibers of the superficial layer of lamina propria. (Courtesy of Steven Gray, MD.)
The vocal folds may be thought of as the oscillators of the vocal mechanism.43 Above the true vocal folds are tissues known as the “false vocal folds.” Unlike the true vocal folds, they do not make contact during normal speaking or singing. However, they may produce voice during certain abnormal circumstances. This phenomenon is called dysphonia plica ventricularis. Until recently, the importance of the false vocal folds during normal phonation was not appreciated. In general, they are considered to be used primarily for forceful laryngeal closure; they come into play during pathological conditions. However, contrary to popular practice, surgeons should recognize that they cannot be removed without affecting phonation. The physics of airflow through the larynx is very complex, involving vortex formation and sophisticated turbulence patterns that are essential to normal phonation. The false vocal folds provide a downstream resistance, which is important in this process, and they probably play a role in vocal tract resonance, as well.
Laryngeal: Blood Supply and Lymphatic Drainage
The larynx receives its blood supply primarily from the inferior and superior laryngeal arteries and the cricothyroid artery. The superior laryngeal arteries arise from the superior thyroid arteries, which are branches of the external carotid arteries. The superior laryngeal arteries course with the superior laryngeal nerves, piercing the thyrohyoid membrane with the internal branch of the superior laryngeal nerve. The superior laryngeal arteries supply the structures related to the quadrangular membranes and piriform sinuses, primarily above the cricoid cartilage. These include the epiglottis, aryepiglottic fold, thyroarytenoid (TA) muscle, lateral cricoarytenoid (LCA) muscle, interarytenoid (IA) muscle, and vocal fold mucosa. There are anastomoses with the superior laryngeal artery on the contralateral side and with the inferior laryngeal artery. The cricothyroid (CT) artery also arises from the superior thyroid artery and supplies the CT muscle and membrane, some of the extrinsic laryngeal musculature, and portions of the subglottic pharynx (after penetrating the cricothyroid membrane). The inferior laryngeal arteries arise from the inferior thyroid arteries, branches of the thyrocervical trunk. On the left, the inferior thyroid artery may arise directly from the subclavian artery. The inferior laryngeal arteries travel with the recurrent laryngeal nerves to supply the posterior cricoarytenoid (PCA) muscle, and probably portions of the true vocal fold ventricles and false vocal folds, in some cases. The superior laryngeal veins join the internal jugular veins, and the inferior laryngeal veins empty into the thyrocervical trunks and from there communicate with the subclavian venous system.
The blood supply to the vocal fold mucosa deserves special attention. It is unusual in several ways. The vocal folds contain only small vessels including arterioles, venules, and capillaries. They run parallel to the vibratory margin of the vocal fold, entering from anterior or posterior, and they have frequent arteriovenous anastomoses.44 This arrangement appears to optimize vessel patency and blood flow even in the presence of the substantial shearing forces encountered during high-pressure phonation. Although Franz and Aharinejad have suggested that there are venous connections between the mucosa and muscle,44 other authors disagree.45,46 There appear to be no direct communications between the microvasculature of the superficial lamina propria and the medial belly of the thyroarytenoid (vocalis) muscle. However, Franz and Aharinejad appear to have been correct in suggesting that the serpentine course of subepithelial vessels is engineered to accommodate safely the extreme changes in length and tension that may occur during phonation.45 The appearance of blood vessels on the superior surfaces of vocal folds may vary in the presence of laryngeal pathology. For example, De Biase and Pontes47 reviewed 280 videolaryngoscopic images divided into groups of 70 patients each with vocal nodules (VNs), polyps, minimal structural alterations (MSAs) or no abnormalities (control group). Visible superior surface vessels were found in 91.4% of the MSA group, 77.1% of patients with polyps, 44.7% of VN subjects, and 31.4% of controls. Longitudinal and transverse vessels were present in 74.3% and 37.1% (respectively) of the MSA group, 65.7% and 22.9% of subjects with polyps, 34.3% and 12.9% of VN patients, and only 25.7% and 5.7% of controls. Tangled vessels were present in only the MSA subjects (8.6%). Abrupt changes in vessel caliber and sinuous vessels were found in the subjects with polyps (21.4% and 5.7%, respectively) and MSA subjects (61.4% and 27.1%).
The vessels along the vibratory margin have a different structure than those on the superior surface of the vocal fold or in the TA muscle.24 For example, capillaries along the vibratory margin are lined by endothelial cells and encircled by pericytes with tight intercellular junctions. The endothelial cells have intermediate-thickness filaments near the cell nucleus and bundles of thick filaments adjacent to the luminal cell membrane.46 These filaments, together with the lamellate structure of the basement membrane and its interspersed myocytes and pericytes, form a lattice that stabilizes the structure of the microvessels, helping them to tolerate the high shearing forces that can be generated during phonation.46 This structure enhances mechanical support and helps explain the relative infrequency with which vocal fold hemorrhage occurs, even during forceful phonation. The other vessels of the vocal fold and laryngeal muscles are composed mainly of simple, endothelial-lined capillaries of the continuous (nonfenestrated) variety (the most common type of capillary).48
Lymphatic drainage from the larynx occurs through superficial and deep systems, although the deep system is most important. The superficial lymphatic system communicates bilaterally and provides only intramucosal drainage. Each deep system is submucosal and drains its lateral structures. Lymphatic drainage from the larynx courses superiorly and inferiorly. Supraglottic lymphatic vessels travel with the superior laryngeal and superior thyroid vessels to deep cervical lymph nodes (levels II and III) associated with the internal jugular veins. This drainage may be bilateral. There are also lymphatic vessels from the laryngeal ventricles that course through the cricothyroid membrane and thyroid gland en route to the prelaryngeal, prethyroid, supraclavicular, pretracheal, and paratracheal lymph nodes. Inferiorly, lymphatic vessels from the glottic and subglottic larynx form 2 posterolateral pedicles and a middle pedicle. The posterolateral pedicles travel unilaterally with the inferior thyroid artery to the deep lateral cervical (levels III and IV), subclavian, paratracheal, and tracheoesophageal lymph nodes. The middle pedicle courses through the cricothyroid membrane, communicating with pretracheal and delphian nodes, which drain into the deep cervical lymph nodes. There is scant lymphatic drainage from the true vocal folds.
Larynx: Intrinsic Muscles
The intrinsic muscles are responsible for abduction, adduction, and tension of the vocal folds (Figures 1–6 and 1–7). All but one of the muscles on each side of the larynx are innervated by the 2 recurrent (or inferior) laryngeal nerves, which are discussed in detail below. Because these nerves usually run long courses from the neck down into the chest and back up to the larynx (hence, the name “recurrent”), they are easily injured by trauma, neck surgery, and thoracic surgery. The left recurrent laryngeal nerve is more susceptible to injury during chest surgery because of its course around the aortic arch. The right recurrent laryngeal nerve, however, is more likely to have an oblique course laterally in the neck and thus may be at greater risk for injury in neck surgery. Such injuries may result in abductor and adductor paralysis of the vocal fold. The remaining muscle, the CT muscle, is innervated by the superior laryngeal nerve on each side, which is especially susceptible to viral and traumatic injury.
For some purposes, including electromyography and surgery, it is important to understand the function of individual laryngeal muscles in detail. The muscles of primary functional importance are those innervated by the recurrent laryngeal nerves including the thyroarytenoid (TA), posterior cricoarytenoid (PCA), lateral cricoarytenoid (LCA), and interarytenoid or arytenoideus (IA), and the superior laryngeal nerves including the cricothyroid (CT) (Figures 1–6, 1–7, and 1–8).
The TA muscle adducts, lowers, shortens, and thickens the vocal folds, thus rounding the vocal fold’s edge. Thus, the cover (epithelium and superficial layer of lamina propria) and transition (intermediate and deep layers of lamina propria) are effectively made slacker, while the body is stiffened.
Figure 1–6. The intrinsic muscles of the larynx.
Figure 1–7. A. Action of the intrinsic muscles. In the bottom 4 figures, the directional arrows suggest muscle actions but may give a misleading impression of arytenoid motion. These drawings should not be misinterpreted as indicating that the arytenoid cartilage rotates around a vertical axis. The angle of the long axis of the cricoid facets does not permit some of the motion implied in this figure. However, the drawing still provides a useful conceptualization of the effect of individual intrinsic muscles, so long as the limitations are recognized. B. The shapes of the glottis as seen on mirror examination and on anatomic preparations during rest (a), inspiration (b), phonation (c), whispering (d), and falsetto singing (e). (Reproduced with permission from Pernkopf.1)
Figure 1–8. A. Schematic presentation of the function of the laryngeal muscles. The left column shows the location of the cartilages and the edge of the vocal folds when the laryngeal muscles are activated individually. The arrows indicate the direction of the force exerted. 1, thyroid cartilage; 2, cricoid cartilage; 3, arytenoid cartilage; 4, vocal ligament; 5, posterior cricoarytenoid ligament. The middle column shows the views from above. The right column illustrates contours of frontal sections at the middle of the musculomembranous portion of the vocal fold. The dotted line illustrates the vocal fold position when no muscle is activated. CT, cricothyroid; VOC, vocalis; LCA, lateral cricoarytenoid; IA, interarytenoid; PCA, posterior cricoarytenoid. (Reproduced with permission from Hirano M.13(p8)) B. The superior and recurrent laryngeal nerve branch from the vagus nerve and enter the larynx.
Adduction from vocalis muscle (the medial belly of the TA) contraction is active, particularly in the musculomembranous segment of the vocal folds. Contraction of the vocalis muscle tends to lower vocal pitch. The thyroarytenoid originates anteriorly from the posterior (interior) surface of the thyroid cartilage and inserts into the lateral base of the arytenoid cartilage, from the vocal process to the muscular process. More specifically, the superior bundles of the muscle insert into the lateral and inferior aspects of the vocal process and run primarily in a horizontal direction. The anteroinferior bundles insert into the anterolateral aspect of the arytenoid cartilage from its tip to an area lateral to the vocal process. (These fibers are associated primarily with the lateral belly.) The most medial fibers run parallel to the vocal ligament and insert onto the medial aspect of the vocal process. There are also cranial fibers that extend into the aryepiglottic fold. Anteriorly, the vertical organization of the muscle results in a twisted configuration of muscle fibers when the vocal fold is adducted. The neuromuscular organization of the medial belly of the TA muscle is more complex than previously believed. Research by Sanders helped clarify these complexities. He has shown, for example, that muscle groups along the superior and inferior margin of the medial (contact) surface of the vocal folds function differently from one another.49 The thyroarytenoid is the third largest intrinsic muscle of the larynx. The TA muscle is divided into 2 compartments. The medial compartment is also known as the vocalis muscle. It contains a high percentage of slow-twitch muscle fibers. The lateral compartment has predominantly fast-twitch muscle fibers. One may suspect that the medial compartment (vocalis) is specialized for phonation, whereas the lateral compartment (muscularis) is specialized for vocal fold adduction, but these suppositions are unproven. De Campos et al studied TA muscle in comparison with tongue, because the tongue muscle is considered the most complex structure in the body with regard to movements and muscle fiber orientation.50 The authors speculated that TA complexities might be correlated with similar muscle structure. They found that both tongue and TA muscle showed the same percentage of transverse (about 72%), undefined (about 15%), and longitudinal (about 10%) fibers. In contrast to these similarities, they found no morphometric correlation comparing the structures of the recurrent laryngeal nerve and the hypoglossal nerve. Studies of TA muscle using myosin antibodies have shown that TA muscle contains unique extrafusal fibers containing tonic myosin, but no muscle spindles.51 These findings suggest similarities between thyroarytenoid muscle function and extraocular muscles, both of which perform unloaded (non-weight-bearing) contractions without afferent information from native muscle spindles.
The LCA muscle is a small muscle that adducts, lowers, elongates, and thins the vocal fold. All layers are stiffened, and the vocal fold edge takes on a more angular or sharp contour in response to LCA muscle contraction. It originates on the superior lateral border of the cricoid cartilage and inserts into the anterior lateral surface of the muscular process of the arytenoid. It is an extremely important adductor and is especially important in the initial movement from abduction to adduction. The bilaterally innervated IA muscle (arytenoideus, or interarytenoid muscle, a medium-sized intrinsic muscle) primarily adducts the cartilaginous portion of the vocal folds. It is particularly important in providing medial compression to close the posterior glottis. It has relatively little effect on the stiffness of the musculomembranous portion. The interarytenoid muscle is the only unpaired laryngeal muscle. It is innervated by the recurrent laryngeal nerve and receives fibers from the internal branch of the superior laryngeal nerve (although there is no evidence that the internal branch of the superior laryngeal nerve provides motor innervation to this muscle. The IA muscle consists of transverse and oblique fibers. The transverse fibers originate from the lateral margin of one arytenoid and insert into the lateral margin of the opposite arytenoid. The oblique fibers originate posteriorly and inferiorly from the base of the cricoid cartilage, but they extend around the apex of the arytenoid cartilage to continue as the aryepiglottic musculature.
The PCA muscle abducts, elevates, elongates, and thins the vocal fold by rocking the arytenoid cartilage posterolaterally. All layers are stiffened, and the edge of the vocal fold is rounded during PCA muscle contraction. It is the second largest intrinsic muscle. It originates over a broad area of the posterolateral portion of the cricoid lamina and inserts on the posterior surface of the muscular process of the arytenoid cartilage, forming a short tendon that covers the cranial aspect of the muscular process. Asanau et al studied the PCA.52 They found 2 separate muscle bellies in all cases. They contained 1, 1-11A, and 11A fibers, but comparing the vertical and horizontal bellies of the PCA revealed differences in the fiber-type composition. They also noted regional differences in both bellies of the PCA. They suggested that the PCA should be considered as a combination of 2 functional subunits differing significantly in muscle fiber-type composition, rather than as a single muscle.
When the superior laryngeal nerves are stimulated, the CT muscles move the vocal folds into the paramedian position. They also lower, stretch, elongate, and thin the vocal folds, stiffening all layers and sharpening the vocal folds’ contours. It is the largest intrinsic laryngeal muscle and is largely responsible for longitudinal tension, a very important factor in control of pitch. Contraction of the CT muscles tends to increase vocal pitch through their lengthening effect on the vocal folds. The CT muscle originates from the anterior and lateral portions of the arch of the cricoid cartilage and has 2 bellies. The oblique belly inserts into the posterior half of the thyroid lamina and the anterior portion of the inferior cornu of the thyroid cartilage. The vertical (erect) belly inserts into the inferior border of the anterior aspect of the thyroid lamina.
Intrinsic laryngeal muscles are skeletal muscles. Skeletal muscles are composed primarily of 3 types of fibers. Type I fibers are highly resistant to fatigue, contract slowly, and utilize aerobic (oxidative) metabolism. They have low glycogen levels, high levels of oxidative enzymes, and are relatively smaller in diameter. Fast-contracting type IIB fibers are subdivided into types IIA, IIB, and IIX. The myosin heavy-chain isoform in each fiber type is primarily responsible for determining contraction speed. Only type I, IIA, and IIX fibers are present in humans. Type IIA fibers use principally oxidative metabolism but contain both a high level of oxidative enzymes and glycogen. They contract rapidly but are also fatigue resistant. Type IIB fibers are found in small mammals and are the most fatigable but fastest-contracting fiber types. They also are the largest in diameter. They utilize anaerobic glycolysis primarily and contain a large amount of glycogen but relatively few oxidative enzymes. Human laryngeal muscles generally contain a mixture of type I, IIA, and IIX fibers, with the proportion of each varying from muscle to muscle. The fiber composition of laryngeal muscles differs from that of most larger skeletal muscles. Elsewhere, muscle fiber diameters are fairly constant, ranging between 60 and 80 microns. In laryngeal muscles, there is considerably more variability,53,54 and fiber diameters vary between 10 and 100 microns, with an average diameter of 40–50 microns. The TA and lateral CT muscles are designed for rapid contraction. Laryngeal muscles have a higher proportion of type IIA fibers than most other muscles, which makes them particularly well suited to rapid contraction with fatigue resistance.55 In addition, many laryngeal motor units have multiple neural innervations. There appear to be approximately 20–30 muscle fibers per motor unit in a human CT muscle,56 suggesting that the motor unit size of this laryngeal muscle is similar to that of extraocular and facial muscles.57 In the human TA muscle, 70%–80% of muscle fibers have 2 or more nerve endplates and some fibers have as many as 5 nerve endplates.58 Only 50% of CT and LCA muscle fibers have multiple endplates, and multiple endplates are even less common in the PCA muscle (5%). It is still not known whether one muscle fiber can be part of more than one motor unit (receive endplates from different motor neurons), or whether all of the endplates on each muscle are associated with the same motor neuron.55
Recent research has provided additional interesting observations in laryngeal muscles. Wu et al found that human PCA and TA muscles express 3 types of myosin heavy-chain (MyHC) isoforms, including slow type I, fast type 2A, and fast type 2X.59 Single-fiber analysis has demonstrated regional differences, and the common occurrence of hybrid fibers.59 Recent research by Malmgren and coworkers has also demonstrated that laryngeal muscles remain capable of spontaneous regeneration over a lifetime and that the proportion of regenerating fibers (identified immunohistochemically by the presence of the developmental myosin isoforms) increases as the TA muscle ages.60 Malmgren et al also speculate that the increase in regenerating fibers may be a compensatory response to an age-related increase in muscle fiber injury or death. These new findings are consistent with clinical observations that many “aging changes” of the voice can be reversed through voice therapy.61
Larynx: Innervation
The recurrent and superior laryngeal nerves are branches of the 10th cranial nerves, or the vagus nerves, which are the longest of the cranial nerves. The vagus nerves originate from 8 to 10 rootlets in a groove in the brainstem between the olive and inferior cerebellar peduncles, in close association with the origins of the 9th and 11th cranial nerves. The rootlets attach to the medulla oblongata in the brainstem, and course below the cerebellar flocculus, where they unite to form the 10th cranial nerve, which exits the cranium through the jugular foramen with the spinal accessory nerve, the glossopharyngeal nerve, and the jugular vein. The hypoglossal nerve exits the skull through the hypoglossal canal, which is adjacent to the jugular foramen and separated from it by a septum. The first ganglion of the vagus nerve is the jugular ganglion, which is located in the jugular foramen, and is known also as the superior ganglion of the vagus nerve. The nodose ganglion (inferior ganglion) is the second ganglion of the vagus nerve and is located in the neck, slightly inferior to the jugular foramen. The vagus nerve contains branchial motor fibers, somatic sensory fibers (from the posterior external auditory canal, from skin adjacent to the ear in some people, and from the tympanic membrane and pharynx), special sensory fibers (taste), visceral motor fibers, and visceral afferent fibers (from the upper respiratory tract, esophagus, stomach, pancreas, abdominal viscera, aortic bodies, aortic arch, and heart). Cells for the visceral, special sensory afferent, and somatic sensory afferent fibers originate in the jugular ganglion and the nodose ganglion. Visceral afferent fibers have cell bodies in the inferior vagal ganglion. Their axons ascend to the medulla oblongata, then descend in the tractus solitarius from which they enter the caudal aspect of the nucleus of the tractus solitarius, also called the nucleus solitarius. Sensory afferent fibers from the larynx and pharynx travel with the visceral afferent fibers via the recurrent and superior laryngeal nerves to their cell bodies in the inferior ganglion.
The nucleus ambiguus is the branchial motor nucleus for the external branch of the superior laryngeal nerve, the recurrent laryngeal nerve, and the pharyngeal nerve and its plexus. Bilateral corticobulbar fibers from the motor cortexes descend through the internal capsule to synapse with the nucleus ambiguus. This bilateral innervation is important in maintaining coordinated, voluntary control of the laryngeal muscles. The motor neurons of the CT muscle are situated rostrally in the nucleus ambiguus, just caudal to the nucleus of the facial nerve. More rostrally, the motor neurons of the TA, LCA, and PCA muscles are located in overlapping pools.62,63 The LCA, TA, and IA motor neurons are located in close association to each other (partially overlapping) and are separate from the CT motor neurons, which are more rostrally co-located with pharyngeal and palatal motor neurons.64 Davis and Nail62 have shown that there are larger motor neurons associated with the TA and LCA muscles (fast-acting muscles used to constrict and protect the airway) than with the PCA and CT muscle (slower-acting muscles that dilate the airway for respiration). Many other neuromotor regions of importance to phonation are beyond the scope of this chapter (mouth, tongue, palate, pharynx, chest, abdomen) but should be considered part of the anatomy of the voice.
Premotor neurons, or interneurons, control motor neurons; motor neurons with multiple functions may be controlled by more than 1 premotor neuron. For example, for phonation, the premotor neuron controlling the TA muscle is associated with the nucleus retroambiguus, located caudally in the medulla. However, for swallowing functions, the TA muscle can be driven by premotor neurons elsewhere in the medulla and pons.65
The periaqueductal gray (PAG) matter is a central area of particular interest to voice specialists and has been studied elegantly by several investigators, especially Pamela Davis, PhD, in Sydney, Australia. The PAG is a region of gray matter, composed of neuronal cell bodies, surrounding the cerebral aqueduct. It is contiguous with gray matter surrounding the third ventricle in the hypothalamus and thalamus rostrally and with gray matter surrounding the pontine portion of the fourth ventricle caudally. Stimulation of the PAG, or of other regions that stimulate the PAG (such as the hypothalamus),66 produces vocalization; destruction of the PAG produces mutism.67–70 The neurons in the PAG form longitudinal columns and are essential to emotional expression.71 The lateral column of neurons in the PAG is responsible for vocalization, increases in blood pressure and heart rate associated with the fight-or-flight response, alterations in bodily blood flow, and nonopioid analgesia.72–75 PAG neurons control vocalization by anastomosing with cells in the nucleus retroambiguus, the origin of premotor neurons discussed above. In addition to stimulating motor neurons in the nucleus ambiguus associated with the larynx, the nucleus retroambiguus stimulates motor neurons associated with chewing; movements of the tongue, palate, face, and pharynx; and motor activity in the chest and abdomen.62 The PAG region receives numerous connections from the cortex,76 and it may be involved in emotional expression associated with the voice in speech and song. It clearly plays an important role in spontaneous emotional voicing in animals, newborns, and adult humans.77 The PAG matter is also involved in muscle control during respiration,72,78,79 a function linked closely with phonation. Interestingly, Davis and coworkers have shown that vocalization activated by the PAG is also associated with laryngeal and vagal afferent input, suggesting that the duration of vocalization is controlled, not just by stimulation of vocalization (including emotional content), but also by the amount of air available in the lungs.80 Ambalavanar demonstrated that afferents from the internal branch of the superior laryngeal nerve travel to the portions of the PAG involved in vocalization, which may be the pathway associated with coordinating such reflex control.81 Interestingly, the Lombard effect, which is so important to speakers and singers (the tendency to speak more loudly in the presence of background noise), is also represented in the PAG.82
Bandler et al have suggested that the PAG may not activate and deactivate laryngeal, respiratory, and orofacial motoneurons directly, but rather may establish “emotional and vocal readiness,” with activation actually being dependent on higher brain structures.75 They suggest that this may explain why damage to the PAG and/or the anterior cingulate cortex produces mutism, even when other components of the voluntary vocal motor system are intact, and why motor cortex injury does not cause mutism when the lateral PAG remains intact to produce emotional vocalization.
Broca area and the sensory-motor regions of the lateral cerebral cortex are involved in control for vocalization. In 1959, Penfield and Roberts published classic descriptions of the function of the primary motor cortex based on cortical stimulation performed in patients with epilepsy.83 Vocalization was localized in the lowest portion of the cortical strip. Stimulation of this region produced activity not only in laryngeal muscles, but also in those of the jaw, lips, and tongue. The premotor cortex (Brodmann area 6) is located close to the primary voice motor cortex, as is the supplementary motor region, which is involved in initiation of speech. Adjacent subcortical areas are also important for speech, but their functions are not understood completely. Most axons in the cerebral cortex cross the midline. So, functions such as motor control from the left hemisphere may affect primarily the right side of the body. However, some functions, including those controlled by cranial nerves (ie, facial and laryngeal muscle function), may be ipsilateral and/or contralateral.
Studies utilizing dynamic imaging techniques such as positron emission tomography (PET) have begun to improve our understanding of function in these areas of the brain. Perry et al used PET to study singing.84 They found hyperperfusion during singing in various areas associated with the motor cortex, including the anterior cingulate cortex, precentral gyri, anterior insula, supplementary motor area, and the cerebellum. The central nervous system specializations that enable processing of acoustically complex voice signals are not understood fully, although it is likely that there are “voice cells” in the brain. Using functional magnetic resonance imaging (fMRI), in 2011 Perrodin et al identified “voice cells” analogous to “face cells” in the brains of monkeys.85 Unlike face cells, they found that voice clusters contain moderate proportions of voice cells that exhibit high stimulus selectivity. Looking at a broader prospective of the brain’s management of speech production, Simonyan and Horwitz published a review of laryngeal motor cortical control during speech and other laryngeal activities.86 They suggested that the location of the laryngeal motor cortex is in the primary motor cortex and its direct connections with the brainstem laryngeal motor neurons in humans, as opposed to its location in the premotor cortex with only indirect connections to the laryngeal motor neurons in nonhuman primates. They suggested that this major evolutionary development in humans is associated with the human ability to speak and vocalize voluntarily. In addition to dynamic imaging (cerebral blood flow) fMRI studies, a variety of other methods can be used to study neurophysiology of brain function, including neuropsychological testing, dichotic listening, dichaptic touching, split-field tachistoscopic viewing, electroencephalography with evoked potential testing, and other techniques. Some of these approaches have been used to study cerebral dominance and its relationship to musical faculty. It is popularly believed that music and art are associated with the type of thinking performed primarily in the right brain of a right-handed individual, while language is represented primarily by processes in the left brain. At present, it is unclear whether these traditional models are valid; it seems probable that complex interactions involving numerous cortical and subcortical areas of both sides of the brain are involved in complicated activities such as emotional expression through speech or song. In a particularly interesting study, Kleber et al examined the brains of expert opera singers, conservatory-level voice students, and laymen during singing of an Italian aria, using fMRI.87 The researchers were interested in evaluating effects of motor-skill training. Their experiments revealed that voice training is associated with increased functional activation bilaterally of the primary somatosensory cortex representing articulators in the larynx. There was additional activation in the right primary sensory motor cortex, the inferior parietal lobe, and the dorsolateral prefrontal cortex bilaterally. Expert singers also showed subcortical changes including increased activation in the basal ganglia, thalamus, and cerebellum. Increased vocal training also correlated with increased activity of a cortical network for enhanced kinesthetic motor control and sensory motor guidance, as well as increased involvement of motor memory areas at the cerebellar and subcortical levels. Further research into these observations should provide interesting information that might be applied to voice training, diagnosis, and rehabilitation. Although other areas of the brain are beyond the scope of this chapter, it should be noted that there are many brain activities of interest and importance to voice patients and clinicians. For example, hearing is essential to optimal voice and speech production, so understanding auditory brain function also is important for voice care, as is understanding areas involved in emotion and many other functions.
The superior laryngeal nerve branches off the vagus nerve high in the neck at the inferior end of the nodose ganglion. It travels between the internal and external carotid arteries, dividing into an internal and external branch near the posterior aspect of the hyoid bone. The external branch courses inferiorly with the superior thyroid artery and vein over the constrictor muscle and through the posterior cricothyroid membrane into the larynx (see Figure 8–8B). Chuang et al studied the anatomic positions of the external branch of the superior laryngeal nerve in Chinese adults. They noted that the inferior corner of the thyroid cartilage was a reliable landmark for identifying the external branch but that there were variations in the position of the nerve.88 Using the Cernea classification,89 after investigating 86 nerves in 43 cadavers they identified Cernea type 1 position in 16.2% (nerve crossing the superior thyroid vessels more than 1 cm above the horizontal plane passing through the upper border of the superior pole of the thyroid gland), type 2a anatomy in 39.5% (nerve crossing the vessels less than 1 cm above the horizontal plane), and type 2b in 38.3% (nerve crossing the superior thyroid vessels below the horizontal plane). They concluded that 77.8% of the superior laryngeal nerve external branches studied were in positions of high risk for injury during thyroid surgery. These findings are similar to those of other authors.90,91 The authors recommended identifying the nerve inferiorly at the inferior cornu of the thyroid cartilage and reported a nerve identification rate significantly better than that reported by authors who identify the nerve distally at the inferior constrictor-cricothyroid junction92 but different from those of Cernea89 and others.93–95 While it is possible that there may be racial differences (the Chuang study was performed off Chinese adults), Furlan studied the question and found no statistically significant different between ethnic groups, but they reported variations in the external branches superior laryngeal nerve related to individual stature and thyroid volumes.94 The external branch supplies the CT muscle. An extension of the external branch may also supply motor and sensory innervation to the vocal folds. Wu et al have identified this extension of the external branch of the superior laryngeal nerve as the human communicating nerve.96 This neural connection was found in 12 (44%) of 27 specimens. When present, it exited the medial surface of the cricothyroid muscle and entered the lateral surface of the thyroarytenoid muscle. The communicating nerve was composed of an intramuscular branch, which combined with the recurrent laryngeal nerve or terminated within the thyroarytenoid muscle directly, and an extramuscular branch that passed through the thyroarytenoid muscle and terminated in the subglottic mucosa in the region of the cricoarytenoid joint. The communicating nerves contain an average of 2510 myelinated axons, of which 31% were motor neurons. Wu et al believe that when the communicating nerve is present, it supplies a second source of motor innervation to the thyroarytenoid muscle and extensive sensory innervation to the subglottic area and cricoarytenoid joint.
The internal branch of the superior laryngeal nerve is responsible primarily for sensation in the mucosa at and above the level of the vocal fold, but it may be responsible for some motor innervations of laryngeal muscles, as well. The internal branch of the superior laryngeal nerves is divided into 3 divisions.97 The superior division supplies the mucosa on the laryngeal surface of the epiglottis. The middle division supplies portions of the true vocal folds, false vocal folds, and the aryepiglottic folds. The inferior division supplies the mucosa of the arytenoids, the portion of the subglottis not supplied by the recurrent laryngeal nerve, the upper esophageal sphincter, and the anterior wall of the hypopharynx. The internal branch of the superior laryngeal nerves also supplies the thyroepiglottic and cricoarytenoid joints.
Terminal sensory nuclei also are involved in reflex pathways associated with the reticular formation. The distributions of the sensory nerves within the larynx in humans remain somewhat speculative, being inferred primarily from research performed in cats.98 Yoshida and coworkers found that the internal branch at the superior laryngeal nerve supplies the ipsilateral side of the epiglottis, aryepiglottic fold, arytenoid eminence, rostral aspect of the vocal fold, vestibule, and mucosa overlying the PCA muscle. The posterior branch of the recurrent laryngeal nerve divides into two branches, one of which goes to Galen anastomosis. The other sensory branch provides bilateral supply with ipsilateral dominance to the caudal aspect of the vocal fold and the subglottic region. Some fibers from the internal branch of the superior laryngeal nerve join with fibers from the posterior branch of the recurrent laryngeal nerve to share innervation of the posterior wall of the glottis and the medial aspect of the arytenoids bilaterally with ipsilateral predominance.99 The cell bodies of the sensory fibers arise primarily in the nodose ganglion and project to the ipsilateral nucleus solitarius. Special sensory fibers for taste from the epiglottis and the larynx course with the vagus nerve to the tractus solitarius and its nucleus.
The recurrent laryngeal nerves branch off the vagus in the chest. On the left, the nerve usually loops around the aortic arch from anterior to posterior and passes lateral to the ligamentum arteriosum behind the arch to enter the tracheoesophageal groove. Occasionally, the nerve is not “recurrent” and does not loop around the aortic arch. Instead it branches directly off the vagus nerve in the neck and courses directly to the larynx. On the right, it usually loops around the brachiocephalic or subclavian artery. This anatomic relationship is usually, but not always, present; and nonrecurrent recurrent nerves occur, probably in less than 1% of people. Nonrecurrent right “recurrent” laryngeal nerves are seen most commonly when the right subclavian artery arises from the descending aorta. In such cases, the “recurrent” nerve arises in the neck and travels directly to the larynx. The recurrent nerves travel superiorly in the tracheoesophageal grooves, entering the larynx between the esophagus and tracheopharyngeus muscle. As they course toward the larynx, the recurrent nerves give off branches to the heart, esophagus, trachea, pharynx, and larynx. The recurrent nerves run perpendicularly between the first 2 branches of the inferior thyroid artery and are attached closely to the posterior, medial aspect of the thyroid lobe. They enter the larynx coursing just below or under the inferior constrictor muscle and communicate with the ansa Galeni, a connection between the posterior branch of the recurrent laryngeal nerve and the internal branch of the superior laryngeal nerve, which is described in more detail in the next paragraph below. Interestingly, it has been found that the myelinated fibers in the left recurrent laryngeal nerve are larger in diameter than those on the right.100 This led Malmgren and Gacek to speculate that differences in fiber size may allow the simultaneous activation of laryngeal muscles via faster transmission rates on the left, despite the fact that the right recurrent laryngeal nerve is shorter than the left101 and thus should otherwise transmit faster causing signal activation sooner. More recently, Jotz et al reexamined the question of histological asymmetry of the RLN.102 They found that the intraperineural area and perimeter of fibers of the right RLN were statistically larger than those of the left RLN. They speculated that the morphological differences were related to the different time of arrival of the stimulus to the laryngeal muscles.
Within the larynx, the recurrent laryngeal nerve crosses from posterior to anterior usually at a level slightly below the cricoarytenoid joint. Usually, the recurrent nerve passes approximately 4 to 5 mm posterior to the cricothyroid joint, but in up to about 15% of adults, the nerve may split around the joint, or it may pass anterior to it. These landmarks may be particularly important during surgical procedures in which identification of the cricothyroid joint is necessary. As the recurrent nerve enters the region of the cricothyroid joint, it divides into branches to each of the intrinsic muscles to which it provides motor innervation. It appears that the first branch usually goes to the PCA muscle. This posterior branch also innervates the IA muscles. An anterior branch courses toward the LCA muscle and supplies the TA muscle, as well. Detailed studies of the courses and variations on the terminal branches of the recurrent laryngeal nerves have not yet been published. Research is currently underway to address this deficiency in knowledge, which has become relevant clinically because of surgical procedures such as thyroarytenoid neurectomy. Consequently, the thyroarytenoid branch was studied first.103 In this study, we determined that the median distance from the inferior tubercle of the thyroid cartilage to the thyroarytenoid branch of the recurrent laryngeal nerve was 3.75 mm. Fifty-four percent of the nerves traveled in a horizontal direction within the larynx, but vertical and oblique orientations were observed. The thyroarytenoid division of the recurrent laryngeal nerve branched in approximately 20% of specimens. From this study, we concluded that surgeons performing thyroarytenoid neurectomy can identify the likely position of the thyroarytenoid nerve by measuring approximately 4 mm from the inferior tubercle along a perpendicular line. In most specimens, the nerve was encountered within 1 to 4 mm from the inferior tubercle. In 2007, the author (RTS) and colleagues performed similar studies on the innervation of the posterior cricoarytenoid muscle, in an effort to explore the practicality of PCA nerve section for abductor spasmodic dysphonia.104 Microscopic dissection allowed the identification and measurement of the branches from the recurrent laryngeal nerves (RLNs) to the PCA in the 43 human cadaver larynges. The cricothyroid (CT) joint was the primary landmark for measurement. All of the PCA muscles received innervation from the anterior division of the RLN. The number of direct branches from the RLN ranged from 1 to 5 (average 2.3). More than 70% of PCA muscles also received 1–3 branches off of the branch to the interarytenoid (IA) muscle. Less than half of PCA muscles received any kind of nerve branches from the posterior division of the RLN. Branches to the PCA most commonly departed the main RLN in its vertical segment, and all entered the muscle from its deep surface. Branches departed the RLN within an average of 9.5 mm from the CT joint; the branch to the IA occurred distal to this point. The innervation to the PCA is complex and redundant, and the segment of the RLN supplying those branches is difficult to expose safely. For these reasons, selective denervation or reinnervation procedures limited to the PCA nerve branches may be difficult technically. When needing only to denervate the PCA, this can be accomplished by removing a portion of the PCA and the underlying nerve supply, maximizing efforts to avoid injury to the branches to the IA muscle. In addition to motor innervation, the recurrent laryngeal nerves are also responsible for sensory innervation primarily below the level of the true vocal folds, and of the spindles of the intrinsic muscles,105 although they may supply portions of the vocal folds as noted above. There are interconnections between the superior and recurrent laryngeal nerves, particularly in the region of the IA muscles. The IA muscles are also the only laryngeal muscles that receive bilateral innervation (both recurrent laryngeal nerves).
Sympathetic innervation of the larynx is from the superior cervical ganglion. Parasympathetic innervation from the dorsal motor nucleus travels to the supraglottic larynx with the internal branch of the superior laryngeal nerves and to the subglottic larynx with the recurrent laryngeal nerves. The larynx also contains other important structures not discussed in detail in this chapter, including chemoreceptors, taste buds, and various mechanoreceptors, Meissner corpuscles, free nerve endings, and Merkel cells. The superior and recurrent laryngeal nerves are also connected through the ramus communicans, also called the ansa Galeni or nerve of Galen, which supplies motor innervation to the tracheal and the esophageal mucosa and the smooth muscle of the trachea. It also supplies the chemoreceptors and baroreceptors of the aortic arch. The laryngeal chemoreflex is an interesting phenomenon that produces cardiovascular changes and central apnea in response to chemical stimulation of the larynx.106,107 The laryngeal chemoreflex may be triggered by stimuli such as gastric acid and can produce responses including laryngeal adduction, bronchoconstriction, hypotension, bradycardia, apnea, and possibly sudden infant death syndrome.108 Like sudden infant death syndrome, the laryngeal chemoreflex is seen usually only in infants under the age of 1. It differs from the glottic closure reflex in response to swallowing and from laryngospasm, which involves glottic closure without central apnea or cardiovascular changes. There is also a laryngeal reflex that results in glottic closure in response to gentle supraglottic tactile stimulation.
The larynx also contains low-threshold, rapidly adapting proprioceptors and low-threshold slowly adapting proprioceptors. The low-threshold, rapidly adapting proprioceptors are found in laryngeal joint capsules and control laryngeal muscle tone during joint movement (such as during singing or speech). Low-threshold, slowly adapting proprioceptors are found in the laryngeal muscles and help to fine-tune laryngeal muscle tone during activities such as phonation. The laryngeal proprioceptors are associated with 2 interesting polysynaptic reflex arcs that were identified in 1966.109 When stimulated, the facilitatory reflex arc increases the rate of motor unit firing in the TA and CT muscles. When the inhibitory reflex arch is stimulated, motor unit firing is decreased in the TA, CT, and sternothyroid muscles. Proprioceptors are probably also important in control of laryngeal muscle tone during respiration.110
Larynx: Extrinsic Muscles
Extrinsic laryngeal musculature maintains the position of the larynx in the neck. This group of muscles includes primarily the strap muscles. Because raising or lowering the larynx may alter the tension or angle between laryngeal cartilages, thereby changing the resting lengths of the intrinsic muscles, the extrinsic muscles are critical in maintaining a stable laryngeal skeleton that permits effective movement of the delicate intrinsic musculature. In the Western classically trained singer, the extrinsic muscles maintain the larynx in a relatively constant vertical position throughout the pitch range. Such training of the intrinsic musculature results in vibratory symmetry of the vocal folds, producing regular periodicity of vocal fold vibration. This contributes to what the listener perceives as a “trained” voice.
The extrinsic muscles may be divided into those below the hyoid bone (infrahyoid muscles) and those above the hyoid bone (suprahyoid muscles). The infrahyoid muscles include the thyrohyoid, sternothyroid, sternohyoid, and omohyoid muscles (Figure 1–9). As a group, the infrahyoid muscles are laryngeal depressors. The thyrohyoid muscle originates obliquely from the thyroid lamina and inserts into the lower border of the greater cornu of the hyoid bone. Contraction brings the thyroid cartilage and the hyoid bone closer together, especially anteriorly. The sternothyroid muscle originates from the first costal cartilage and the posterior aspect of the manubrium of the sternum, and it inserts obliquely on the thyroid cartilage. Contraction lowers the thyroid cartilage. The sternohyoid muscle originates from the clavicle and posterior surface of the manubrium of the sternum, inserting into the lower edge of the body of the hyoid bone. Contraction lowers the hyoid bone. The inferior belly of the omohyoid originates from the upper surface of the scapula and inserts into the intermediate tendon of the omohyoid muscle low in the lateral neck. The superior belly originates from the intermediate tendon and inserts into the greater cornu of the hyoid bone. The omohyoid muscle pulls the hyoid bone down, lowering it.
The suprahyoid muscles include the digastric, mylohyoid, geniohyoid, and stylohyoid muscles. As a group, the suprahyoid muscles are laryngeal “elevators.” The posterior belly of the digastric muscle originates from the mastoid process of the temporal bone and inserts into the intermediate tendon of the digastric, which connects to the hyoid bone. The anterior belly originates from the inferior aspect of the mandible near the symphysis and inserts into the digastric intermediate tendon. The anterior belly pulls the hyoid bone anteriorly and raises it. The posterior belly pulls the hyoid bone posteriorly and also raises it. The mylohyoid muscle originates from the inner aspect of the body of the mandible (mylohyoid line) and inserts into a midline raphe on the hyoid, connecting with fibers from the opposite side. It raises the hyoid bone and pulls it anteriorly. The geniohyoid muscle originates from the spine at the mental symphysis of the mandible and inserts on the anterior surface of the body of the hyoid bone. It raises the hyoid bone and pulls it anteriorly. The stylohyoid muscle originates from the styloid process and inserts into the body of the hyoid bone. It raises the hyoid bone and pulls it posteriorly. Coordinated interaction among the extrinsic laryngeal muscles is needed to control the vertical position of the larynx, as well as other positions such as laryngeal tilt.
The Supraglottic Vocal Tract
The supraglottic larynx, tongue, lips, palate, pharynx, nasal cavity (see Figure 1–3A), oral cavity, and possibly the sinuses shape the sound quality produced by the vocal folds by acting as resonators. Minor alterations in the configuration of these structures may produce substantial changes in voice quality. The hypernasal speech typically associated with a cleft palate and/or the hyponasal speech characteristic of severe adenoid hypertrophy are obvious. However, mild edema from an upper respiratory tract infection, pharyngeal scarring, or changes in muscle tension produce less obvious sound alterations. These are immediately recognizable to a trained vocalist or astute critic, but they often elude the laryngologist.
The Tracheobronchial Tree, Lungs, Thorax, Abdomen, and Back
The lungs supply a constant stream of air that passes between the vocal folds and provides power for voice production, which is especially important in singing (Figure 1–10). Singers often are thought of as having “big chests.” Actually, the primary respiratory difference between trained and untrained singers is not increased total lung capacity, as popularly assumed. Rather, the trained singer learns to use a higher proportion of the air in his or her lungs, thereby decreasing his or her residual volume and increasing respiratory efficiency.111
The abdominal musculature is the so-called “support” of the singing voice, although singers generally refer to their support mechanism as their “diaphragm.” The function of the diaphragm muscle in singing is complex, and somewhat variable from singer to singer (or actor to actor). The diaphragm primarily generates inspiratory force. Although the abdomen can also perform this function in some situations,112 it is primarily an expiratory-force generator. Interestingly, the diaphragm is coactivated by some performers during singing and appears to play an important part in the fine regulation of singing.113 Actually, the anatomy and physiology of support for phonation are quite complicated and not understood completely. Both the lungs and rib cage generate passive expiratory forces under many common circumstances; however, passive inspiratory forces also occur. The active respiratory muscles working in concert with passive forces include the intercostal, abdominal, back, and diaphragm muscles. The principal muscles of inspiration are the diaphragm and external intercostal muscles. Accessory muscles of inspiration include the pectoralis major; pectoralis minor; serratus anterior; subclavius; sternocleidomastoid; anterior, medial, and posterior scalenus, serratus posterior and superior; latissimus dorsi; and levatores costarum muscles. During quiet respiration, expiration is largely passive. Many of the muscle used for active expiration (forcing air out of the lungs) are also employed in “support” for singing and acting voice tasks, including abdominal, back, and chest muscles.
Figure 1–9. Extrinsic muscles of the larynx and their actions. (From The Larynx. Clinical Symposia. New Jersey: CIBA Pharmaceutical Company; 1964;16[3]: Plate 4. Copyright 1964. Icon Learning Systems, LLC, a subsidiary of MediMedia USA Inc. Reprinted with permission from Icon Learning Systems, LLC, illustrated by Frank H. Netter, MD. All rights reserved.)
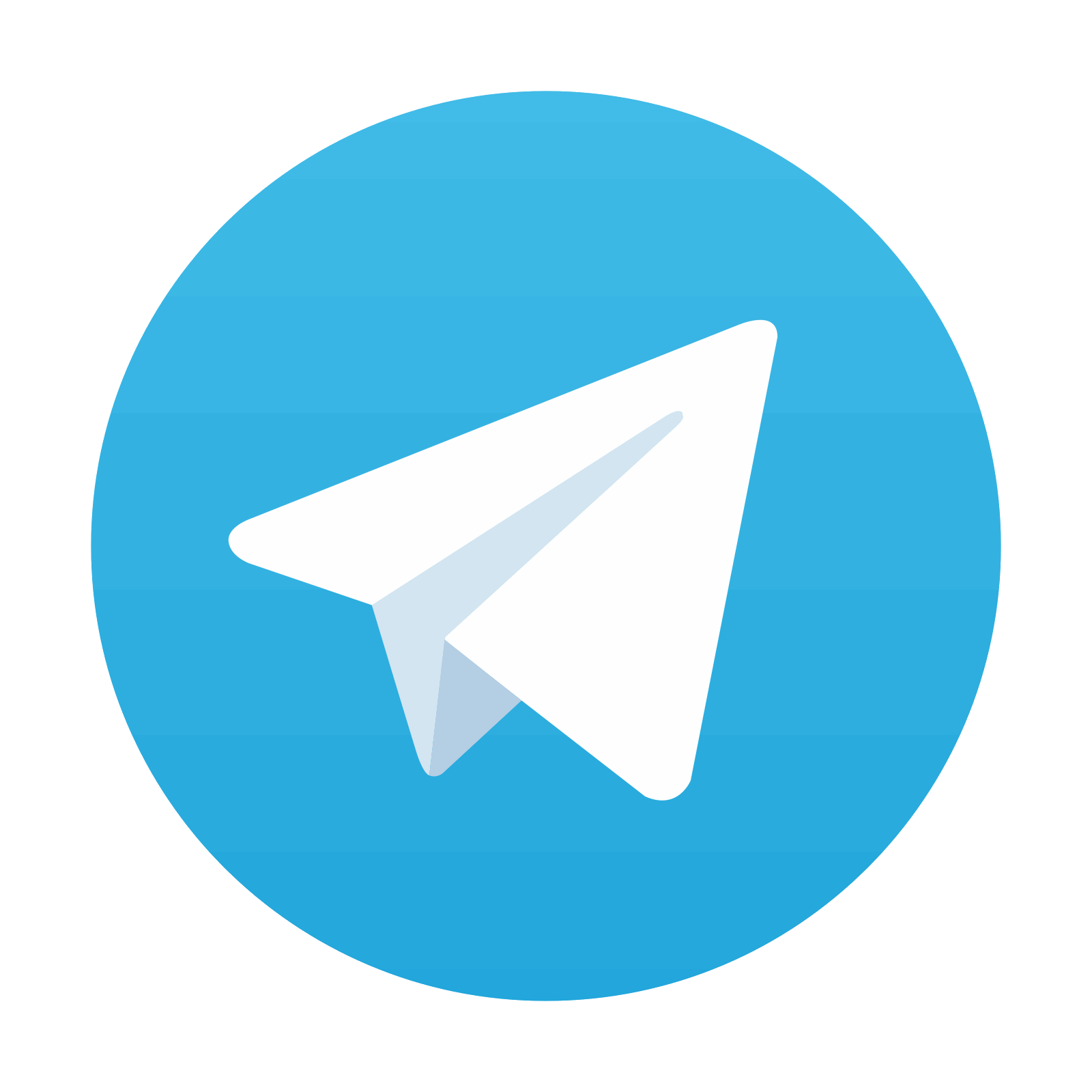
Stay updated, free articles. Join our Telegram channel

Full access? Get Clinical Tree
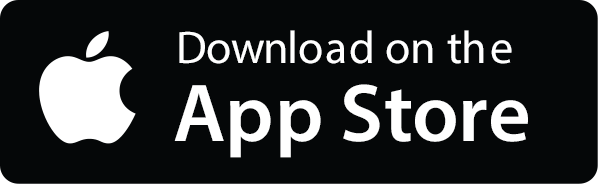
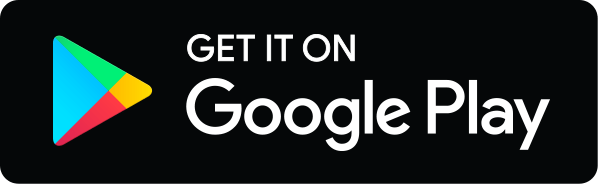