[31]:

(14.1)

Due to the low compressibility of biological material and the high frequency of Brillouin measurements, the Young’s or shear moduli of the cornea in the physiological regime are several orders of magnitude smaller than M′ measured by Brillouin technology.
To understand the relationship between Brillouin-measured longitudinal modulus and traditional Young’s/shear moduli, we used porcine cornea tissue samples. Brillouin depth profiles were acquired on intact porcine corneas and longitudinal modulus values were computed using fixed index/density data taken from the literature. For shear rheometry , cornea tissue samples were cut with a biopsy punch in order to retrieve thin flaps from anterior, central, and posterior portion of the cornea. The shear modulus of the thin flaps was measured at 0.5 Hz frequency with 0.1 % strain amplitude with a stress-controlled rheometer (AR-G2, TA Instruments). For each flap, thickness was accurately measured in order to calculate the corresponding average longitudinal modulus from the Brillouin depth profile. We found a strong correlation between the Brillouin-derived longitudinal modulus and gold standard Young’s modulus in a log–log linear trend (R > 0.99). The log–log linear relationship allows estimating the sensitivity to elastic modulus changes of our technology:

where ΔM′, ΔE′, and ΔG′ are respective variations. The coefficients “a − b” are intrinsic properties of the material under analysis. From our validation on porcine cornea, we estimate our measurement sensitivity of Young’s modulus to be in the order of 5 %.

(14.2)
14.5 Brillouin Microscopy Technology
Since the 1970s, Brillouin scattering spectroscopy has been used for material characterization [32] using the spectrometer developed by J. R. Sandercock. The same spectrometer was also used in the biological realm in the 1980s to measure collagen fibers [33, 34], cornea, and crystalline lens [35] ex vivo. However, the acquisition time of a Brillouin spectrum was in the 10 min to hour range, which made the technology difficult to use in vivo.
Brillouin scattered light is very close in color (i.e., frequency or wavelength) to the input light. In most optical systems and biological specimens, residual components of the input light or reflections, diffusions of the samples are orders of magnitude stronger than Brillouin scattered light. Ordinary spectrometers lack both spectral resolution, i.e., the ability to resolve Brillouin signal from input light, and spectral extinction, i.e., the ability to detect Brillouin scattered light in the presence of strong light with similar color. JR Sandercock’s spectrometer successfully measured Brillouin light using a multiple-pass scanning Fabry–Perot (FP) interferometers [36, 37]. However, FP interferometers are extremely slow because the spectral scanning is achieved by changing the spacing between the two mirrors forming a FP cavity and because only a particular narrowband spectral component is transmitted at one time while the remainder components of the spectrum are reflected and lost.
In 2008, the throughput of Brillouin spectrometer was greatly enhanced by parallel detection [38] enabled by a diffractive tilted etalon, called virtually imaged phased array (VIPA ) [39, 40]. The VIPA spectrometer has fundamentally superior performances to achieve high spectral resolution with high temporal resolution. The spectral selection is given by the interference of multiple reflections at two optical flats thus providing equivalent performances to FP interferometers in terms of resolution. However, the first surface of a VIPA is totally reflective and coated to allow all the light to enter the interferometer. This feature avoids useful light being wasted in a reflected interference pattern. With respect to an equivalent Fabry–Perot spectrometer , the signal strength in a VIPA spectrometer is improved by about two orders of magnitude. This technical improvement has enabled transitioning Brillouin spectroscopy to Brillouin microscopy by combining a laser-scanning confocal microscope with a VIPA-based spectrometer .
VIPA-based Brillouin spectroscopy has been dramatically improved since 2008 in both spectral extinction and efficiency [41–44]. A clinically viable Brillouin microscope and the first Brillouin measurement of the human eye in vivo were reported in 2012. The clinical instrument, sketched in Fig. 14.1, employs low-power laser light at 780 nm scanned across each location of the eye and a Brillouin spectrometer optimized for the infrared wavelength [45].


Fig. 14.1
Schematic of the in vivo instrument for Brillouin microscopy
14.6 Brillouin Microscopy in Keratoconic Corneas
Recent Brillouin measurements for the analysis of keratoconus both ex vivo and in vivo are promising [46, 47]. For ex vivo investigation , normal tissue samples were obtained from donor corneas used in Descemet’s stripping endothelial keratoplasty (DSEK ) while keratoconic tissue samples were obtained as the discarded tissue of deep anterior lamellar keratoplasty (DALK ) procedure in advanced keratoconus patients. For in vivo investigation , patients with advanced keratoconus were measured just before undergoing DALK surgery and their corneal strength was compared to healthy volunteer corneas. The results of ex vivo and in vivo analysis point at several similar features. Overall, keratoconic corneas had lower Brillouin shift, thus lower elastic modulus and decreased mechanical stability. In addition, while the Brillouin shift of the normal corneas was relatively uniform across the central region of the cornea, in keratoconus patients a strong spatial variation was measured with the cone showing the lowest Brillouin shift. The keratoconus cone showed a decrease in longitudinal modulus of ~2–3 % which corresponds to a ~70 % reduction in Young’s modulus based on our empirical conversion factor measured with porcine cornea tissue samples. Interestingly, away from the cone, the elastic modulus was very similar to normal cornea in both ex vivo and in vivo investigations (Fig. 14.2).


Fig. 14.2
Representative maps of the mean anterior Brillouin shift for (a) a healthy subject and (b) a patient with advanced keratoconus. The panels on the left report the respective curvature and pachymetry maps with outlined Brillouin scanned areas
Brillouin microscopy has thus provided the first experimental evidence of a focal weakening or a lack of spatial asymmetry in the distribution of elastic modulus in keratoconic corneas. From a mechanical standpoint, this has important consequences because the focal loss of mechanical strength is thought to represent a critical factor in the progression of keratoconus [48]. From a diagnostic standpoint, this is encouraging because the spatial variation of Brillouin mechanical signatures could be a superior metric to detect the onset and progression of keratoconus. Evaluating the diagnostic and prognostic potential of Brillouin technology will now require the measurement of nonadvanced keratoconus cases and the comparison with morphological changes (pachymetry and topography).
14.7 Future Directions
Brillouin microscopy has the unique potential of providing high-resolution maps of corneal elasticity. For keratoconus this could have widespread application in both ophthalmic research and in the clinic. Currently, the standard of care is morphological characterization by pachymetry and topography; if properly analyzed, the biomechanical properties of the cornea may become an important indicator of corneal health. Early diagnosis of ectasia and keratoconus could allow patients to receive interventions such as corneal cross-linking (CXL) to halt disease progression. Monitoring CXL mechanical outcome could improve the management of keratoconus progression. The identification of early keratoconus could improve screening procedures for LASIK surgery. Beyond keratoconus, biomechanical measurement of corneal elastic modulus is expected to improve the accuracy of tonometry to estimate the intraocular pressure [49, 50].
In summary, at present Brillouin microscopes are being tested in the clinic to assess the potential to characterize keratoconus and ectasia patients as well as CXL procedures. However, a major component of the future of Brillouin microscopy is tied to its technology development. The difference in Brillouin shift between an advanced keratoconic cornea and a healthy control is not difficult to measure, but earlier stages of keratoconus will probably show much smaller differences in Brillouin shift. At the current level of instrumental sensitivities, about ten separable categories are provided by Brillouin microscopy for the stratification of patients into different disease stages. Improving the sensitivity of mechanical modulus measurement will likely result into higher capability of stratifying patients and detecting early cases. Immediate improvement in speed and sensitivity of the instrument could come by increasing the light power used for the Brillouin measurements as all the clinical tests so far reported have used about 1/8 of the illumination power deemed as safe. Straightforward improvement could also come from engineering efforts aimed at reducing the size, cost, and operation burden of Brillouin instrumentation. Such technological development should improve the availability of Brillouin technology to many medical and research facilities thus accelerating its validation for diagnosis and management of keratoconus.
Conflict of Interest
Giuliano Scarcelli and Seok Hyun Yun declare that they have no conflict of interest.
Informed Consent: All procedures followed were in accordance with the ethical standards of the responsible committee on human experimentation (institutional and national) and with the Helsinki Declaration of 1975, as revised in 2000. Informed consent was obtained from all patients for being included in the study.
Animal Studies: No animal studies were carried out by the authors for this article
References
1.
Krachmer JH, Feder RS, Belin MW. Keratoconus and related noninflammatory corneal thinning disorders. Surv Ophthalmol. 1984;28:293–322.CrossRefPubMed
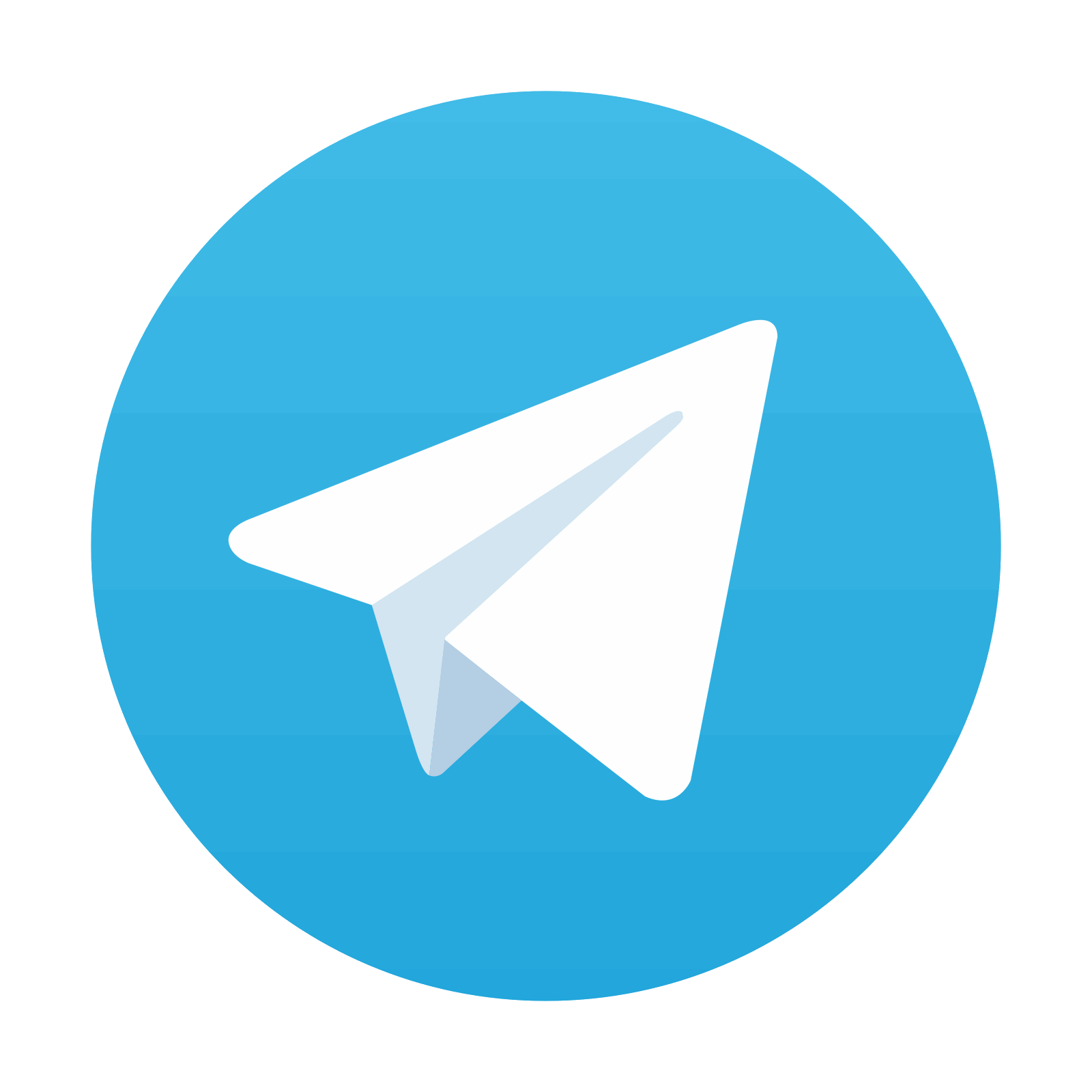
Stay updated, free articles. Join our Telegram channel

Full access? Get Clinical Tree
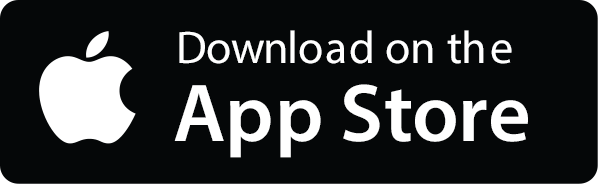
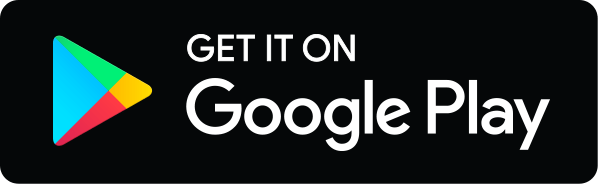