Breathing and Speech Production
The breathing apparatus moves air into and out of the body for the purpose of sustaining life as well as for performing other important functions such as speaking. It includes an energy source and passive components that couple this source to the air it moves.
This chapter begins with detailed consideration of the anatomical bases of breathing, forces and movements of breathing, control variables of breathing, neural control of breathing, and ventilation and gas exchange during tidal breathing. The latter part of the chapter is dedicated to speech breathing and selected variables that influence it.
ANATOMY OF THE BREATHING APPARATUS
The breathing apparatus is located within the torso (body trunk). A skeleton of bone and cartilage forms the framework for the breathing apparatus. This skeletal framework and the subdivisions of the breathing apparatus are considered here. The muscles of the breathing apparatus are covered under Forces of the Breathing Apparatus.
Terms can either enlighten you or get you into verbal quagmires. Respiratory physiologists have gone out of their way to be precise in their use of terms. They’ve even held conventions to iron out their differences in language. It’s a good idea to take a little extra time and care when reading the early sections of this chapter. Let the lexicon of the respiratory physiologist take firm root. Don’t be tempted to skip over parts just because the words in the headings look familiar to you. You may be surprised to find that a term you thought you understood actually has an entirely different meaning to a respiratory physiologist.
The skeletal framework of the breathing apparatus is depicted in Figure 2–1. At the back of the torso, 34 irregularly shaped vertebrae (bones) form the vertebral column or backbone. The uppermost 7 of these vertebrae are termed cervical (neck), the next lower 12 are called thoracic (chest), and the next three lower groups of 5 each are referred to as lumbar, sacral, and coccygeal (collectively, abdominal). The vertebral column constitutes a back centerpost for the torso.
The ribs make up most of the upper skeletal framework. They are 12 flat, arch-shaped bones on each side of the body. The ribs slope downward from back to front along the sides of the torso, forming the rib cage and giving roundness to the framework. At the front, most of the ribs attach to bars of costal (rib) cartilage, which, in turn, attach to the sternum or breastbone. The sternum serves as a front centerpost for the rib cage. The typical rib cage includes upper pairs of ribs attached to the sternum by their own costal cartilages, lower pairs that share cartilages, and the lowest two pairs that float without front attachments.
The remainder of the upper skeletal framework is formed by the pectoral girdle (shoulder girdle). This structure is near the top of the rib cage. The front of the pectoral girdle is formed by the two clavicles (collar bones), each of which is a strut extending from the sternum over the first rib toward the side and back of the rib cage. At the back, the clavicles attach to two triangularly shaped plates, the scapulae (shoulder blades). The scapulae cover most of the upper back portion of the rib cage.
Two large, irregularly shaped coxal (hip) bones are located in the lower skeletal framework. These two bones, together with the sacral and coccygeal vertebrae, form the pelvic girdle (bony pelvis). The pelvic girdle comprises the base, lower back, and sides of the lower skeletal framework.
Figure 2–1. Skeletal framework of the breathing apparatus consisting of the clavicles, ribs, sternum, pelvic girdle, scapulae, and vertebral column.
Breathing Apparatus and Its Subdivisions
The breathing apparatus (breathing pump) and its subdivisions are depicted in Figure 2–2. The torso, which houses the apparatus, consists of upper and lower cavities that are partitioned by the diaphragm. The upper cavity is called the thorax (or colloquially the chest) and is almost totally filled with the heart and lungs; the lower cavity, called the abdomen (or colloquially the belly), contains much of the digestive system and other organs and glands. The structures of the breathing apparatus form two major subdivisions: the pulmonary apparatus and chest wall. These subdivisions are concentrically arranged, with the chest wall surrounding the pulmonary apparatus.
The pulmonary apparatus, portrayed in Figure 2–3, is the air-containing, air-conducting, and gas-exchanging part of the breathing apparatus. It provides oxygen to the cells of the body and removes carbon dioxide from them. The pulmonary apparatus can be subdivided into two components: the pulmonary airways and lungs.
Pulmonary Airways. The pulmonary airways constitute a complex network of flexible tubes through which air can be moved to and from the lungs and between different parts of the lungs. These tubes are arranged like the branches of an inverted deciduous tree. The network, in fact, is commonly referred to as the pulmonary tree.
The trunk of the pulmonary tree (the top part) is the trachea (windpipe). The trachea is a tube attached to the bottom of the larynx (voice box). It runs down through the neck into the torso. The trachea is composed of a series of C-shaped cartilages whose open ends face toward the back where the structure is completed by a flexible wall shared with the esophagus (the muscular tube leading to the stomach). At its lower end, the trachea divides into two smaller tubes, one running to the left lung and one running to the right lung. These two tubes, called the main-stem bronchi, branch into what are called lobar bronchi, tubes that run to the five lobes of the lungs (two lobes on the left and three on the right). The five lobar bronchi branch and their offspring also branch, and so on, through more than 20 generations. Each successive branching leads to smaller and less rigid structures. These include, in succession, segmental bronchi, subsegmental bronchi, small bronchi, terminal bronchi, bronchioles, terminal bronchioles, respiratory bronchioles, alveolar ducts, alveolar sacs, and alveoli. The last of these, the alveoli, are extremely small cul de sacs filled with air. They number more than 300 million and are the sites where oxygen and carbon dioxide are exchanged. The total surface area of the alveoli, if laid out flat, is said to approximate the size of a tennis court.
Figure 2–2. Breathing apparatus and its subdivisions: the pulmonary apparatus (lungs and airways) and the chest wall (rib cage wall, diaphragm, abdominal wall, and abdominal content). From Evaluation and management of speech breathing disorders: Principles and methods (p. 13), by T. Hixon and J. Hoit, 2005, Tucson, AZ: Redington Brown. Copyright 2005 by Thomas J. Hixon and Jeannette D. Hoit. Modified and reproduced with permission.
Figure 2–3. The pulmonary apparatus consisting of the airways (trachea, main-stem bronchi, lobar bronchi, segmental bronchi, subsegmental bronchi, small bronchi, terminal bronchi, bronchioles, terminal bronchioles, respiratory bronchioles, alveolar ducts, alveolar sacs, and alveoli) and lungs (spongy structures that surround the pulmonary airways).
Lungs. The lungs are the organs of breathing. They are a pair of cone-shaped structures that are porous and spongy. Each lung contains an abundance of resilient elastic fibers and behaves like a stretchable bag. The outer surfaces of the lungs are covered with a thin airtight membrane, called the visceral pleura. A similar membrane, the parietal pleura, covers the inner surface of the chest wall where it contacts the lungs. Together these two membranes form a double-walled sac that encases the lungs. Both walls of this sac are covered with a thin layer of liquid that lubricates them and enables them to move easily upon one another. The same layer of liquid links the visceral and parietal membranes together, in the way a film of water holds two glass plates together. This is called pleural linkage. Thus, the lungs and chest wall tend to move as a unit—where one goes, the other follows.
The chest wall encases the pulmonary apparatus. There are four parts to the chest wall: the rib cage wall, diaphragm, abdominal wall, and abdominal content.
Rib Cage Wall. The rib cage wall surrounds the lungs and is shaped like a barrel. The rib cage framework includes the thoracic segments of the vertebral column, the ribs, the costal cartilages, the sternum, and the pectoral girdle. The remainder of the rib cage wall is formed by muscular and nonmuscular tissues that fill the spaces between the ribs and cover their inner and outer surfaces.
Diaphragm. The diaphragm forms the convex floor of the thorax and the concave roof of the abdomen. The diaphragm separates the thorax and abdomen, and thus, gets its name—diaphragm meaning the fence between. The diaphragm is dome-shaped, like an inverted bowl. The left side of the diaphragm is positioned slightly lower than the right (to accommodate the heart above and the liver below). At its center, the diaphragm consists of a tough sheet of inelastic tissue, called the central tendon. The remainder of the structure is formed by a sheet of muscle that rises as a broad rim from all around the lower portion of the inside of the rib cage and extends upward to the edges of the central tendon.
Abdominal Wall. The abdominal wall provides a casing for the lower half of the torso. This casing is shaped like an oblong tube and runs all the way around the torso. The lower portion of the skeletal framework of the torso forms the structure around which the abdominal wall is built. This includes a back centerpost of 15 vertebrae (lumbar, sacral, and coccygeal) that extends from near the bottom of the rib cage to the tailbone and pelvic girdle. Much of the abdominal wall consists of two broad sheets of connective tissue and several large muscles. The two sheets of connective tissue cover the front and back of the abdominal wall and are called the abdominal aponeurosis and lumbodorsal fascia, respectively. Muscles are located all around the abdominal wall—front, back, and flanks (sides)—and combine with the abdominal aponeurosis, lumbodorsal fascia, vertebral column, and pelvic girdle to form its encircling casing.
Ever watch a frog breathe? Did you notice how its cheeks moved? Frogs don’t have a diaphragm to pull air into their lungs. They push the air in using their mouths like pistons. Frogs are positive pressure breathers. People are negative pressure breathers. A frog doesn’t have the ability to emulate us (except, perhaps, Kermit) if its positive pressure pump fails. But we have the ability to emulate the frog if our negative pressure pump fails by doing what is called glossopharyngeal breathing. And what would you suppose is the common name for such breathing? Well, it’s “frog breathing.” In frog breathing, the tongue and throat are used to pump air into the lungs. Air is gulped in small portions (mouthfuls), each held in place by closing the larynx as a one-way valve. Frog breathing isn’t difficult to learn and once mastered can be used to fill the lungs in a stepwise fashion all the way to the top.
Abdominal Content. The abdominal content is everything in the abdominal cavity. This includes the stomach, intestines, and various other internal structures. This content is close to unit density (the density of water) and constitutes a relatively homogeneous mass. This mass is suspended from above by a suction force at the undersurface of the diaphragm and is held in place circumferentially and at its base by the casing of the abdominal wall. Together, the abdominal cavity and the abdominal content are the mechanical equivalent of an elastic bag filled with water.
Pulmonary Apparatus–Chest Wall Unit
The pulmonary apparatus and chest wall are linked by pleural membranes (pleural linkage) to form a single functional unit. As illustrated in Figure 2–4, the resting positions of the pulmonary apparatus and chest wall when linked as a unit are different from their individual resting positions. When the pulmonary apparatus is removed from the chest wall, its resting position is a collapsed state in which it contains very little air. This is represented as a collapsed spring in the figure (lower left). In contrast, the resting position of the chest wall, with the pulmonary apparatus removed, is a more expanded state, represented as a stretched spring in the figure (lower middle). With the pulmonary apparatus and chest wall held together by pleural linkage, the breathing apparatus assumes a resting position between these two positions such that the pulmonary apparatus is somewhat expanded and the chest wall is somewhat compressed. This resting position of the linked pulmonary apparatus–chest wall unit is a mechanically neutral or balanced state in which the force of the pulmonary apparatus to collapse is opposed by an equal and opposite force of the chest wall to expand. The linked pulmonary apparatus–chest wall unit (breathing apparatus) is represented as a combined spring in Figure 2–4 (lower right).
Figure 2–4. Resting positions of the pulmonary apparatus, chest wall, and pulmonary apparatus–chest wall unit (breathing apparatus). Note that when the pulmonary apparatus and chest wall are linked (right side of figure), the resting size of the pulmonary apparatus is larger and the resting size of the chest wall is smaller than when they are separated. When linked, the forces of the pulmonary apparatus and chest wall are equal and opposite and the pulmonary–chest wall unit assumes a mechanically neutral (balanced) state.
Laundry Starch
The stiffness of the breathing apparatus changes across life. The term that pertains to this is compliance and it refers to the tendency to yield to an applied force. In the lexicon of the respiratory physiologist, compliance is quantified in terms of how much volume is displaced for the pressure applied. We are reminded of laundry starch when we think of this concept. You get a little more starch in the “fabric” of your breathing apparatus as time goes by. The compliance of the breathing apparatus of a newborn is relatively high and somewhat like that of a dishrag. In contrast, the compliance of the breathing apparatus of a senescent adult is relatively low and somewhat like that of a plastic garbage can lid. Thus, we start out life being relatively floppy and end up life being relatively stiff (no pun intended on the stiff part, honestly).
Both passive and active forces operate on the breathing apparatus. Passive force is inherent and always present. Active force, in contrast, is applied willfully by skeletal (voluntary) muscles.
The passive force of breathing can be substantial. It comes from: (a) the natural recoil of muscles, cartilages, ligaments, and lung tissue, (b) the surface tension of alveoli, and (c) the pull of gravity. Passive force causes the breathing apparatus to behave like a coil spring (like that illustrated in the lower right of Figure 2–4), which, when stretched or compressed, tends to recoil toward its resting length.
The active force of breathing comes from the actions of muscles of the chest wall. The sign (inspiratory or expiratory) and magnitude of this force depend on which muscles are active and in what combinations. Active force also depends on the amount of air in the breathing apparatus. The more air in the apparatus, the greater the active force that can be generated to expire; and the less air in the apparatus, the greater the active force that can be generated to inspire.
The roles of individual muscles in generating active force are described below for the rib cage wall, diaphragm, and abdominal wall. These descriptions assume that only the muscle under consideration is active and that it is shortening during contraction. It should be noted, however, that several factors might influence the contribution of an individual muscle, including the actions of other muscles, the mechanical status of different parts of the chest wall, and the breathing activity being performed.
The muscles of the rib cage wall are defined to include muscles of the neck and rib cage. These muscles are depicted in different views in Figure 2–5.
The sternocleidomastoid muscle is a broad, thick structure positioned on the front and side of the neck. It originates in two subdivisions, one at the top and front of the sternum and the other at the top of the sternal end of the clavicle. Fibers from these subdivisions pass upward and backward and insert into the bony skull behind the ear. When the head is fixed in position, contraction of the sternocleidomastoid muscle results in elevation of the sternum and clavicle. The force generated is transmitted to the ribs through their connections to the sternum and clavicle. Consequently, the ribs are also elevated.
Sounds like leftovers. Actually, it refers to rotational stress produced when one end of a rib is twisted out of line with the other. Some have suggested that when the ribs are elevated during resting tidal inspiration, they’re twisted outward (placed under positive torque) and store energy, which is then supposedly released during expiration. Not so. The ribs are actually twisted inward (and are under negative torque) at the resting tidal end–expiratory level because the lungs are pulling inward on the rib cage wall at that level. The ribs untwist during resting tidal inspiration, but do not reach neutral (zero torque) until the 60 %VC (percent vital capacity) level is attained (when in an upright body position). Because resting tidal inspiration involves only about a 10% increase in vital capacity, rib torque actually opposes resting tidal expiration rather than assists it. The only thing leftover about rib torque in this context is the folklore.
The scalenus anterior, scalenus medius, and scalenus posterior muscles are three separate muscles that form a functional group. These are positioned on the side of the neck. The scalenus anterior muscle originates from the third through sixth cervical vertebrae and runs downward and toward the side to insert along the inner border of the top of the first rib. The scalenus medius muscle arises from the lower six cervical vertebrae and descends along the side of the vertebral column to insert into the first rib behind the point of insertion of the scalenus anterior muscle. And the scalenus posterior muscle originates from the lower two or three cervical vertebrae and passes downward and toward the side to attach to the outer surface of the second rib. When the head is fixed in position, contraction of the scalenus anterior and/or scalenus medius muscles results in elevation of the first rib, whereas contraction of the scalenus posterior muscle results in elevation of the second rib.
The pectoralis major muscle is a broad, fan-shaped muscle positioned on the upper front wall of the rib cage. This muscle has a complex origin that includes the front surface of the upper costal cartilages, sternum, and inner half of the clavicle. Fibers run across the front of the rib cage wall and converge to insert into the humerus (the major bone of the upper arm). When the humerus is held in position, contraction of the pectoralis major muscle pulls the sternum and ribs upward.
Figure 2–5. Muscles of the rib cage wall. From Evaluation and management of speech breathing disorders: Principles and methods (pp. 19–20), by T. Hixon and J. Hoit, 2005, Tucson, AZ: Redington Brown. Copyright 2005 by Thomas J. Hixon and Jeannette D. Hoit. Modified and reproduced with permission.
The pectoralis minor muscle is a relatively large, thin muscle that lies underneath the pectoralis major muscle. Its fibers originate from the second through fifth ribs near their cartilages. From there, they extend upward and toward the side, where they insert into the front surface of the scapula. When the scapula is fixed in position, contraction of the pectoralis minor muscle elevates the second through fifth ribs.
The subclavius muscle is a small muscle that originates from the undersurface of the clavicle. It runs slightly downward and toward the midline, where it attaches at the junction of the first rib and its cartilage. When the clavicle is braced, contraction of the subclavius muscle elevates the first rib.
The serratus anterior muscle is a large muscle positioned on the side of the rib cage wall. It originates from the outer surfaces of the upper eight or nine ribs. Fibers pass backward around the side of the rib cage, where they converge and insert into the front of the scapula. When the scapula is fixed in position, contraction of the serratus anterior muscle results in elevation of the upper ribs.
The external intercostal muscles are 11 muscles that fill the outer portions of the rib interspaces. Each is a thin layer of muscle that runs between adjacent ribs. The fibers of the muscles are oriented forward and downward. Together, the 11 muscles form a large sheet of muscle that links the ribs to one another. This sheet of muscle is anchored from above to the first rib, the cervical vertebrae, and the base of the skull. When the muscle in any rib interspace contracts, it elevates the rib immediately below and, perhaps, other ribs below through their linkage to the sheet of muscle. The external intercostal muscles may activate individually in different rib interspaces or they may activate collectively. En masse activation causes the ribs to move upward as a unit. Muscle activation also stiffens the tissue-filled rib interspaces. This prevents them from being sucked inward and pushed outward as internal pressure is lowered and raised, respectively.
The internal intercostal muscles are 11 muscles that lie in the inner portions of the rib interspaces. They are located underneath the external intercostal muscles and extend from around the sides of the rib cage to the sternum. The internal intercostal muscles do not fill the rib interspaces at the back of the rib cage. Fibers of the internal intercostal muscles run downward and backward and at a right angle to those of the external intercostal muscles. The internal intercostal muscles form a large sheet of muscle that links the ribs to one another and to the pelvic girdle through other muscles, especially those of the abdominal wall. When muscle in a rib interspace contracts, it pulls downward on the rib immediately above and, perhaps, on other ribs above through the linkage created by the muscle sheet. The internal intercostal muscles may activate individually in any rib interspace or they may activate collectively. When they activate collectively, the ribs tend to move downward as a unit. Muscle contraction stiffens the tissue-filled rib interspaces and prevents them from being sucked inward and bulged outward with changes in internal pressure.
The portion of the internal intercostal muscles that lies between the costal cartilages (the intercartilaginous internal intercostal muscles) is arranged such that the muscle tissue exerts an upward pull on the rib cage wall rather than the downward pull exerted by the portion of the muscle that lies between the bony ribs (the interosseous internal intercostal muscles). Thus, the internal intercostal muscles play a functional role in the intercartilaginous region that is similar to that played by their companion external intercostal muscles throughout the rib cage wall. Stated otherwise, the two layers of intercostal muscles (external and internal) function similarly toward the front of the rib cage, but dissimilarly at other locations.
The transversus thoracis muscle is a fan-shaped structure located on the inside, front wall of the rib cage. It originates at the midline on the inner surface of the lower sternum and fourth or fifth through seventh costal cartilages. From there, it fans out across the rib cage and inserts into the inner surface of the costal cartilages and bony ends of the second through sixth ribs. The upper fibers of the muscle run nearly vertically, whereas the intermediate and lower fibers course at other angles. When the transversus thoracis muscle contracts, it exerts a downward pull on the second through sixth ribs.
The latissimus dorsi muscle is a large muscle positioned on the back of the body. It has a complex origin from the lower six thoracic, lumbar, and sacral vertebrae, along with the back surfaces of the lower three or four ribs. Fibers run upward across the back of the lower torso at different angles to insert into the humerus. When the humerus is fixed in position, contraction of the fibers of the latissimus dorsi muscle that insert into the lower ribs will elevate them. Contraction of the muscle as a whole, in contrast, compresses the lower portion of the rib cage wall. Thus, the latissimus dorsi muscle is capable of generating active force of different signs (inspiratory and expiratory).
The serratus posterior superior muscle is located on the upper back portion of the rib cage wall. It is a thin muscle that originates from the back of the vertebral column. Points of origin include the seventh cervical and first three or four thoracic vertebrae. Fibers course downward across the back of the rib cage and insert into the second through fifth ribs. When the serratus posterior superior muscle contracts, it pulls upward on the second through fifth ribs.
The serratus posterior inferior muscle is a thin muscle positioned on the lower back portion of the rib cage wall. It arises from the lower two thoracic and upper two or three lumbar vertebrae and slants upward across the back of the rib cage where it inserts into the lower borders of the lower four ribs. Contraction of the serratus posterior inferior muscle results in a downward pull on the lower four ribs.
The lateral iliocostalis muscle group includes three muscles located on the back of the torso. These are positioned to the side of the vertebral column and extend between the cervical and lumbar regions. The lateral iliocostalis cervicis muscle originates from the outer surfaces of the third through sixth ribs and courses upward and toward the midline to insert into the fourth through sixth cervical vertebrae. The lateral iliocostalis thoracis muscle arises from the upper edges of the lower six ribs and courses upward to insert into the lower edges of the upper six ribs. And the lateral iliocostalis lumborum muscle originates from the lumbodorsal fascia, lumbar vertebrae, and back surface of the coxal bone. It courses upward and toward the side to insert into the lower edges of the lower six ribs. Contraction of the lateral iliocostalis cervicis muscle causes elevation of the third through sixth ribs, whereas contraction of the lateral iliocostalis lumborum muscle results in depression of the lower six ribs. Contraction of the lateral iliocostalis thoracis muscle stabilizes large segments of the back of the rib cage wall and makes them move in concert with either the rib elevation or depression caused by the cervical and lumbar elements of the muscle group, respectively.
The levatores costarum muscles are 12 small muscles positioned on the back of the rib cage wall. Their origin is from the seventh cervical and upper eleven thoracic vertebrae and they extend downward and slightly outward to insert into the back surface of the rib immediately below the vertebra of origin. When an individual muscle of the levatores costarum muscle group contracts, it elevates the ribs into which it inserts. When the muscle group contracts collectively, its action is similar to that effected by collective contraction of the external intercostal muscles (the ribs elevate as a unit).
The quadratus lumborum muscle is a flat, quadrilateral sheet of muscle located on the back of the torso. It arises from the top of the coxal bone and runs upward and toward the midline where it inserts into the first four lumbar vertebrae and lower border of the inner half of the lowest rib. When the quadratus lumborum muscle contracts, it pulls downward on the lowest rib.
The subcostal muscles comprise a group of thin muscles located on the inside back wall of the rib cage. They differ in number from person to person and are most often located and best developed in the lower portion of the rib cage wall. The subcostal muscles originate near the vertebral column on the inner surfaces of ribs and course upward and toward the side where they insert into the inner surfaces of ribs immediately above, or skip a rib or two and insert into higher ribs. When the subcostal muscles contract, they pull downward on the ribs into which they are inserted.
The muscular features of the diaphragm are portrayed in Figure 2–6. The diaphragm muscle is a large, complex muscle that subdivides the torso into two compartments: the thorax (upper cavity) and abdomen. It originates around the internal circumference of the lower rib cage, including the bottom of the sternum, the lower six ribs and their cartilages, and the first three or four lumbar vertebrae. From this internal rim, muscle fibers radiate upward to insert into the circumference of the central tendon, a broad sheet of inelastic tissue that forms the centermost portion of the diaphragm.
When the diaphragm muscle contracts, it can effect two actions. One of these is to pull the central tendon downward and forward, thus enlarging the thorax vertically; the other is to enlarge the thorax circumferentially through elevation of the lower six ribs. The actions of lowering the base of the thorax and expanding its circumference occur in patterns that depend on the relative stiffness (compliance) of the rib cage wall and abdominal wall.
The muscles of the abdominal wall are depicted in Figure 2–7. They are located on the front and sides of the abdominal wall.
The rectus abdominis muscle is a ribbon-like structure located on the front of the lower rib cage wall and abdominal wall just off the midline. It arises from the upper, front edge of the coxal bone and runs upward vertically to insert into the outer surfaces of the fifth, sixth, and seventh costal cartilages and lower sternum. The rectus abdominis muscle is compartmentalized into four or five short segments by tendinous breaks. The entire muscle is encased in a fibrous sheath formed by the abdominal aponeurosis. The muscle and sheath form a centerpost along the front of the abdominal wall that is a continuation of the front centerpost formed by the sternum on the rib cage wall. When the rectus abdominis muscle contracts, it pulls the lower ribs and sternum downward and forces the front of the abdominal wall inward. The compartmentalized segments of the muscle are also capable of independent contraction.
Figure 2–6. Muscle of the diaphragm shown from different views. Upper panels from Evaluation and management of speech breathing disorders: Principles and methods (p. 25), by T. Hixon and J. Hoit, 2005, Tucson, AZ: Redington Brown. Copyright 2005 by Thomas J. Hixon and Jeannette D. Hoit. Modified and reproduced with permission.
Figure 2–7. Muscles of the abdominal wall. From Evaluation and management of speech breathing disorders: Principles and methods (p. 26), by T. Hixon and J. Hoit, 2005, Tucson, AZ: Redington Brown. Copyright 2005 by Thomas J. Hixon and Jeannette D. Hoit. Modified and reproduced with permission.
The external oblique muscle is a broad structure located on the side and front of the lower rib cage wall and abdominal wall. It originates from the upper surface of the coxal bone and abdominal aponeurosis near the midline. Fibers course upward across the abdominal wall at various angles. The most prominent course is upward and toward the side, with insertions being on the outer surfaces and lower borders of the lower eight ribs. When the external oblique muscle contracts, it pulls the lower ribs downward and forces the front and side of the abdominal wall inward.
The internal oblique muscle is a large muscle positioned on the side and front of the lower rib cage wall and abdominal wall. It lies underneath the external oblique muscle. The internal oblique muscle originates from the upper surface of the coxal bone and lumbodorsal fascia. Its fibers fan out across the abdominal wall to insert into the abdominal aponeurosis and the lower borders of the costal cartilages of the lower three or four ribs. The fibers of the internal oblique muscle run at a right angle to those of the external oblique muscle. When the internal oblique muscle contracts, it pulls the lower ribs downward and forces the front and side of the abdominal wall inward. Thus, its functional potential is similar to that of the external oblique muscle.
The transversus abdominis muscle is a broad structure located on the front and side of the abdominal wall. It lies underneath the internal oblique muscle. The transversus abdominis muscle has a complex origin that includes the upper surface of the coxal bone, lumbodorsal fascia, and inner surfaces of the costal cartilages of ribs seven through twelve. Fibers of the muscle run horizontally around the abdominal wall and insert at the front into the abdominal aponeurosis. The paired left and right transversus abdominis muscles encircle the abdominal wall. When the transversus abdominis muscle contracts, it forces the front and side of the abdominal wall inward.
Summary of Passive and Active Forces
The breathing apparatus can exert passive and active forces that are both inspiratory and expiratory. Figure 2–8 summarizes the passive and active forces for the different parts of the breathing apparatus—the pulmonary apparatus, chest wall, and the individual components of the chest wall that contain muscles (rib cage wall, diaphragm, and abdominal wall). Negative and positive signs in the figure represent forces that tend to inspire (−) and expire (+) the breathing apparatus.
The pulmonary apparatus exerts only passive force. Specifically, it recoils toward a smaller size, like a stretched coil spring. Thus, it operates in the expiratory direction only.
The chest wall can exert both passive and active force. At large chest wall sizes, it recoils inward (expiratory) and at small sizes it recoils outward (inspiratory). Muscles of the chest wall can generate active force to either inspire or expire the breathing apparatus at any chest wall size. Muscles that inspire the apparatus are located in the rib cage wall and diaphragm, and muscles that expire the apparatus are located in the rib cage wall and abdominal wall. Active force available to inspire the breathing apparatus is greater at small chest wall sizes, and active force available to expire the apparatus is greater at large chest wall sizes. This is because the inspiratory and expiratory muscles of the chest wall are on more favorable portions of their length-force characteristics at small and large chest wall sizes, respectively.
Figure 2–8. Passive and active forces of breathing. The pulmonary apparatus has only passive expiratory (+) force, whereas the chest wall (composed of the rib cage wall, diaphragm, and abdominal wall) has both passive and active forces that produce both inspiratory (−) and expiratory (+) force. From Evaluation and management of speech breathing disorders: Principles and methods (p. 31), by T. Hixon and J. Hoit, 2005, Tucson, AZ: Redington Brown. Copyright 2005 by Thomas J. Hixon and Jeannette D. Hoit. Modified and reproduced with permission.
The rib cage wall can contribute both passively and actively to adjustments of the breathing apparatus. It recoils inward at large sizes and outward at small sizes in most body positions. Muscles of the rib cage wall can generate active inspiratory and expiratory force. Those muscles responsible for inspiratory force are generally located in superficial layers of the rib cage wall and those responsible for expiratory force are generally located in deep layers of the wall. Active inspiratory force is greater at small rib cage wall sizes and active expiratory force is greater at large rib cage wall sizes, due to more favorable length–force characteristics for the inspiratory and expiratory muscles at small and large rib cage wall sizes, respectively. The smallest muscles of the breathing apparatus are located in the rib cage wall and provide it with the capability for fast and precise action.
The diaphragm has both passive and active force capabilities. When displaced footward, as it is at large lung volumes, it develops no recoil. In contrast, when displaced headward, as it is at small lung volumes, it recoils in the inspiratory direction. The diaphragm can generate active force in the inspiratory direction only.
The abdominal wall can produce both passive and active force. It recoils in the expiratory direction at large abdominal wall volumes and in the inspiratory direction at small abdominal wall volumes in most body positions. The abdominal wall can generate active force in only the expiratory direction, with greater expiratory force being generated at large abdominal wall volumes because its muscles are on more favorable portions of their length-force characteristics.
The chest wall muscles that exert active force on the breathing apparatus are summarized in Table 2–1. Activation of these muscles can change the volume of the pulmonary apparatus (pulmonary airways and lungs) by increasing or decreasing the circumference of the thorax, increasing or decreasing the vertical length of the thorax (by lowering or raising its floor), or both. Increasing thoracic size (and, thus, increasing the volume of the pulmonary apparatus) is an inspiratory action, and decreasing thoracic size is an expiratory action.
Table 2–1. Muscles of the Chest Wall and Their Potential Actions on the Thorax
Note. Those muscles that increase the circumference or increase the vertical length of the thorax are considered inspiratory muscles and those that decrease the circumference or decrease the vertical length of the thorax are considered expiratory muscles.
Realization of Passive and Active Forces
Forces of breathing are realized in two ways. One way is through pulls on structures and the other way is through pressures developed at various locations. Pulling forces are distributed in a complex fashion. Fortunately, they are uniformly distributed at certain points where they manifest as pressures. The locations of the most important of these pressures are shown in Figure 2–9.
Included among these pressures are alveolar pressure, pleural pressure, abdominal pressure, and transdiaphragmatic pressure. Alveolar pressure is the pressure inside the lungs; pleural pressure is the pressure inside the thorax but outside the lungs (between the pleural membranes); abdominal pressure is the pressure inside the abdominal cavity; and transdiaphragmatic pressure is the difference in pressure across the diaphragm (the difference between pleural pressure and abdominal pressure). Although all of these pressures are critical to the function of the breathing apparatus, the pressure of greatest primacy to the understanding of speech production is alveolar pressure.
Figure 2–9. Pressures of the breathing apparatus. Alveolar pressure is the pressure inside the lungs. Pleural pressure is the pressure between the pleural (visceral and parietal) membranes. Abdominal pressure is the pressure inside the abdomen. Transdiaphragmatic pressure is the difference between the pleural and abdominal pressures.
Breathing is accomplished through movements of the individual parts of the chest wall—rib cage wall, diaphragm, and abdominal wall. These movements can be described for each part individually, as they are below. Nevertheless, the forces that underlie these movements are not necessarily easy to determine. This is because the interactions among the different parts of the chest wall are quite complex.
Movements of the Rib Cage Wall
The rib cage wall is able to move because of two sets of joints, those between the ribs and sternum (costosternal joints) and those between the ribs and the vertebral column (costovertebral joints). Actual movement differs somewhat from rib to rib, owing to differences in the lengths and shapes of individual ribs. Nevertheless, two types of rib movements are typical and are illustrated in Figure 2–10. One type of rib movement is a vertical excursion of its front (sternal) end and is either upward and forward or downward and backward, resulting in an increase or decrease, respectively, in the front-to-back diameter of the rib cage. Each rib rotates through the axis of its neck (at the back near the vertebral column) in a movement pattern that resembles the raising and lowering of the handle on a water pump.
The other type of rib movement is vertical excursion along the side of the rib cage that involves a rotation of the rib around an axis extending between its two ends. The rotation is either upward and outward or downward and inward, the result being an increase or decrease, respectively, in the side-to-side (transverse) diameter of the rib cage. Such movement is similar to the raising and lowering of the handle on a water bucket.
These two types of rib movement occur together and in phase. Thus, the circumference of the rib cage wall increases and decreases along with increases and decreases in its two diameters (front-to-back and side-to-side).
Figure 2–10. Two types of rib movements. They are (1) upward and forward/downward and backward (like the handle on a water pump) and (2) upward and outward/downward and inward (like a bucket handle).
Not Doing What Comes Naturally
The initiation and execution of voluntary breathing movements come naturally for most of us. But, for some individuals with maldeveloped or damaged brains, these may not be easy, or even possible, to do. Their problem is praxis or action. They have dyspraxia when they have difficulty with action, and apraxia when they are unable to carry out action at all. One of the most perplexing clients we ever encountered was a young man who showed difficulties with breathing actions following a traumatic brain injury. When he attempted to initiate voluntary inspirations or expirations on command, he often became frozen in position. Occasionally, his attempt resulted in movement in the opposite direction (he breathed in when trying to breathe out). He knew exactly what he wanted to do, but just couldn’t do it. Imagine the depth of his frustration.
The diaphragm can enlarge the thorax vertically by pulling down on the central tendon or it can enlarge the thorax circumferentially by elevating the lower ribs. It can also do both simultaneously, as depicted in Figure 2–11.
The actions of the diaphragm (Figure 2–11), as well as the actions of the rib cage wall and abdominal wall, can change the configuration of the diaphragm such that it can flatten or become more highly domed, as illustrated in Figure 2–12. Flattening is accompanied by descent of the central tendon and/or elevation of the lower ribs; doming of the diaphragm is accompanied by elevation of the central tendon and/or descent of the lower ribs. In general, the more highly domed the diaphragm, the more active (inspiratory) force it is able to exert. This is because its muscle fibers are stretched and are on more favorable portions of their length–force characteristics.
Movements of the diaphragm are hidden from view and must be inferred from movements of more superficial structures of the chest wall. Diaphragm movements are manifested in changes in the radius of its curvature and depend on the relative fixation of the lower ribs and central tendon. When the rib cage is fixed in position, the movement of the diaphragm is reflected superficially in movement of the abdominal wall; when the central tendon is fixed in position, the movement of the diaphragm is reflected in movement of the rib cage wall.
Figure 2–11. Actions of the diaphragm. The diaphragm can enlarge the thorax vertically by pulling the central tendon downward and forward (downward pointing arrows) and it can expand the thorax circumferentially by lifting the lower ribs (upward pointing arrows). Structural features from Evaluation and management of speech breathing disorders: Principles and methods (p. 25), by T. Hixon and J. Hoit, 2005, Tucson, AZ: Redington Brown. Copyright 2005 by Thomas J. Hixon and Jeannette D. Hoit. Modified and reproduced with permission.
Figure 2–12. Configurations of the diaphragm. The diaphragm can assume a flattened configuration by descent of the central tendon and/or lifting of the lower ribs, and a domed configuration by elevation of the central tendon and/or descent of the lower ribs.
Movements of the Abdominal Wall
When at rest in an upright position, the lower abdominal wall is distended somewhat because the pressure inside the abdomen is greater near the bottom than near the undersurface of the diaphragm. This pressure pushes the lower abdominal wall outward. Inward movement flattens the abdominal wall and outward movement increases the degree to which the abdominal wall is protruded. Most movement occurs in the anteroposterior dimension, as depicted in Figure 2–13.
Relative Movements of the Rib Cage Wall and Diaphragm–Abdominal Wall
The rib cage wall contacts about three-fourths of the surface of the pulmonary apparatus, whereas the diaphragm–abdominal wall unit contacts only about one-fourth of the surface area. This means that a small movement of the rib cage wall can move a substantial amount of air into or out of the pulmonary apparatus or cause a major pressure change within the pulmonary apparatus. In contrast, the diaphragm–abdominal wall unit must move a much greater distance to displace the same amount of air or create the same pressure change. The relative consequences of rib cage wall movement versus diaphragm–abdominal wall movement on pulmonary volume and pressure are illustrated in Figure 2–14.
The breathing apparatus can exert forces that may cause movements, pressure changes, or both. Some of these are confined to the parts of the chest wall in which they occur. Others are the result of actions between different parts of the chest wall. Thus, it is possible for one part of the breathing apparatus to cause movements and/or pressure changes in other parts of the apparatus, as illustrated in Figure 2–15 and discussed in the following examples.
Figure 2–14. Relative movements of the rib cage wall and diaphragm–abdominal wall. The different-sized plungers represent the fact that a small movement of the rib cage wall can cause a large volume and pressure change within the pulmonary apparatus when compared with the same movement of the diaphragm–abdominal wall. This is because the rib cage wall covers approximately three times as much surface area of the pulmonary apparatus compared with the diaphragm–abdominal wall.
Figure 2–15A represents active expansion of the rib cage wall (using inspiratory rib cage wall muscles) and quiescent diaphragm and abdominal wall muscles. In this situation, rib cage wall expansion lowers pleural pressure and pulls the diaphragm headward and the abdominal wall inward. This action is like pulling liquid up a drinking straw (hence the phrase “sucking it in” when referring to the resulting inward movement of the abdominal wall). The opposite situation is shown in Figure 2–15B, where actively compressing the rib cage wall (using expiratory rib cage wall muscles) causes pleural pressure to rise and pushes the diaphragm footward and the abdominal wall outward.
Actions of the diaphragm can move the rib cage wall, abdominal wall, or both, depending on the mechanical status of these two chest wall parts. When the rib cage wall is fixed in position, as depicted in Figure 2–14C, contraction of the diaphragm causes it to move footward and pushes the abdominal wall outward. In contrast, when the abdominal wall is fixed in position, as in Figure 2–14D, diaphragm contraction is resolved into headward displacement (expansion) of the rib cage wall. Usually, neither the rib cage wall nor abdominal wall is rigidly positioned, so that both move in accordance with their relative compliance (stiffness).
Figure 2–15. Examples of how the rib cage wall, diaphragm, and abdominal wall can affect one another. The white arrow in each example indicates which chest wall component is active; the filled arrows represent the effects on the other chest wall components. A. Expansion of the rib cage wall can pull the diaphragm headward and the abdominal wall inward through the lowering of pleural pressure. B. Compression of the rib cage wall can push the diaphragm footward and the abdominal wall outward through the raising of pleural pressure. C. Contraction of the diaphragm can push the abdominal wall outward, if the rib cage wall is fixed. D. Contraction of the diaphragm can lift the rib cage wall upward and outward, if the abdominal wall is fixed. E. Inward displacement of the abdominal wall can push the diaphragm headward and lift and expand the rib cage wall.
Actions of the abdominal wall can cause adjustments in both the rib cage wall and diaphragm. When the muscles of the abdominal wall contract, they force the abdominal wall inward and raise abdominal pressure. As depicted in Figure 2–14E, this action forces the lower rib cage wall outward and the diaphragm headward. The combination of these two effects causes a passive lifting of the rib cage wall.
Actions of the breathing apparatus often seem deceptively simple and can be erroneously ascribed as being caused only by the parts of the apparatus in which the movement is observed. Thus, when observing movements of the rib cage wall, it should not be assumed that the rib cage wall necessarily moved itself. Similarly, movement of the abdominal wall may or may not be ascribed to activation of abdominal wall muscles.
CONTROL VARIABLES OF BREATHING
The breathing apparatus controls a number of variables, some of which are particularly important to speech production. These variables are lung volume, alveolar pressure, and chest wall shape.
Volume is defined as the size of a three-dimensional object or space. The volume of interest here is the volume of air inside the pulmonary apparatus. This volume is termed the lung volume and it reflects the size of the breathing apparatus. Movements of the breathing apparatus can change lung volume by moving air into or out of the pulmonary apparatus. Such movement, sometimes called volume displacement, can occur only if the larynx and upper airway are open.
The volume variable can be partitioned into what are called lung volumes and lung capacities. As shown in Figure 2–16, volume can be displayed in a spirogram, a record of lung volume change over time obtained from a spirometer (a device that records volume displacement; see Chapter 6).
There are four lung volumes. Each covers a portion of the lung volume range that is mutually exclusive of the others.
The tidal volume (TV) is the volume of air inspired or expired during the breathing cycle. When recorded in the resting individual, this volume is termed the resting tidal volume.
The inspiratory reserve volume (IRV) is the maximum volume of air that can be inspired from the tidal end–inspiratory level (the peak of the tidal volume cycle).
The expiratory reserve volume (ERV) is the maximum volume of air that can be expired from the tidal end–expiratory level (the trough of the tidal volume cycle).
The residual volume (RV) is the volume of air remaining in the pulmonary apparatus at the end of a maximum expiration. This volume cannot be measured directly.
There are four lung capacities. Each capacity includes two or more of the lung volumes defined above.
The inspiratory capacity (IC) is the maximum volume of air that can be inspired from the resting tidal end–expiratory level. It is the sum of the tidal volume and the inspiratory reserve volume.
The vital capacity (VC) is the maximum volume of air that can be expired following a maximum inspiration (or inspired following a maximum expiration). It includes the inspiratory reserve volume, the tidal volume, and the expiratory reserve volume.
The functional residual capacity (FRC) is the volume of air in the pulmonary apparatus at the resting tidal end–expiratory level. This capacity includes the expiratory reserve volume and the residual volume. Because this includes the residual volume, it cannot be measured directly.
The total lung capacity (TLC) is the volume of air in the pulmonary apparatus at the end of a maximum inspiration. It includes the inspiratory reserve volume, the tidal volume, the expiratory reserve volume, and the residual volume. This lung capacity cannot be measured directly because it includes the residual volume.
We have seen many young children with cerebral palsy and breathing disorders. Many of these children, especially those who are quadriplegic and show major signs of spasticity, seem to have a governor on them. That is, they behave like there’s a device that is limiting the degree to which they can willfully adjust the breathing apparatus. When asked to perform an inspiratory capacity maneuver (“Take in all the air you can”), they may only be able to inspire to their resting tidal end–inspiratory level. Try it over and over again and the same thing happens. Then, out of the blue, the child shows you a gaping yawn of boredom and takes in an enormous breath. The breath may actually be several times the size the child could generate during voluntary inspiration. Now, you’re faced with a dilemma. What do you record as the child’s inspiratory capacity? Think about it, carefully.
Figure 2–16. A spirogram showing lung volumes and lung capacities. The lung volumes are tidal volume (TV), inspiratory reserve volume (IRV), expiratory reserve volume (ERV), and residual volume (RV). The lung capacities are inspiratory capacity (IC), vital capacity (VC), functional residual capacity (FRC), and total lung capacity (TLC). From Evaluation and management of speech breathing disorders: Principles and methods (p. 36), by T. Hixon and J. Hoit, 2005, Tucson, AZ: Redington Brown. Copyright 2005 by Thomas J. Hixon and Jeannette D. Hoit. Modified and reproduced with permission.
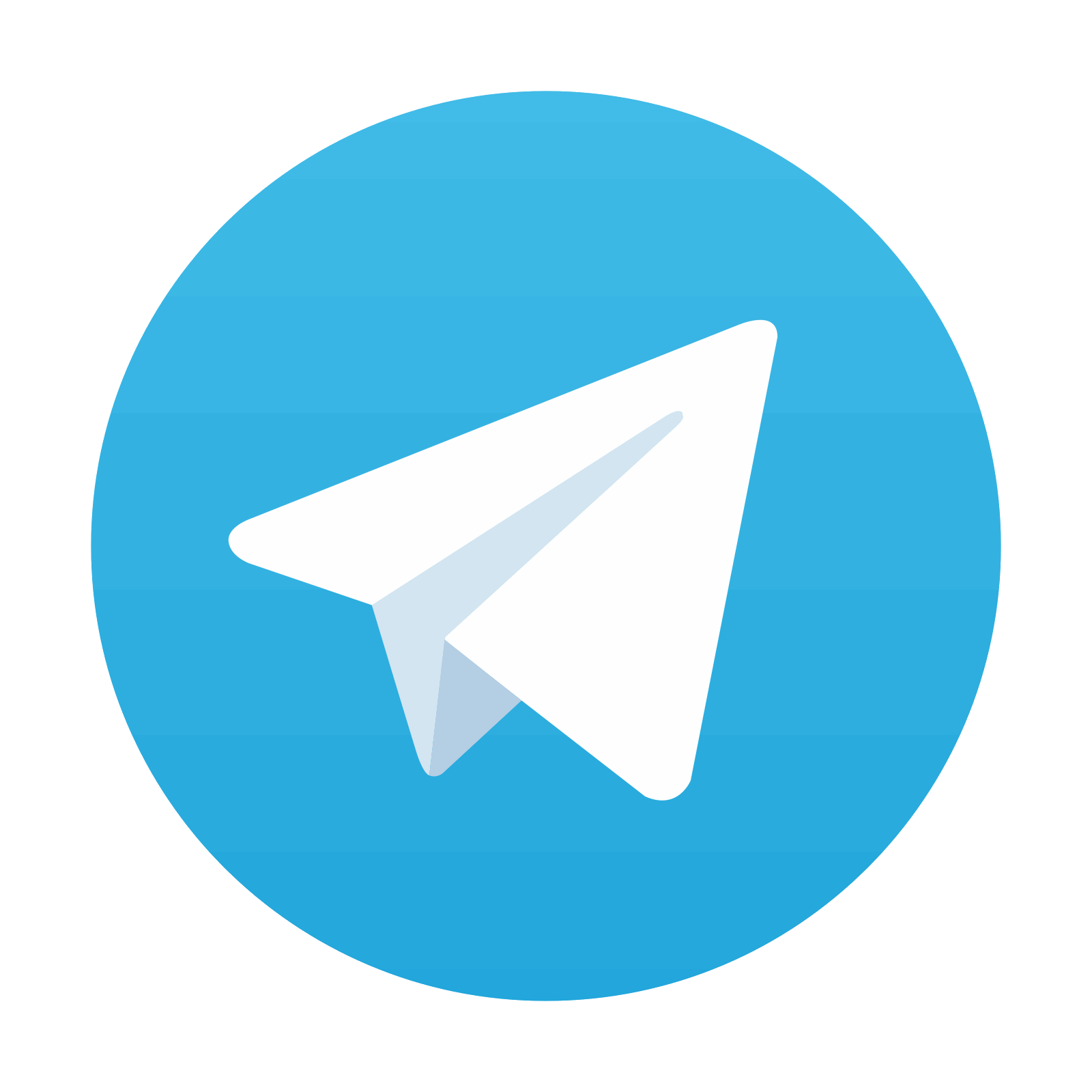
Stay updated, free articles. Join our Telegram channel

Full access? Get Clinical Tree
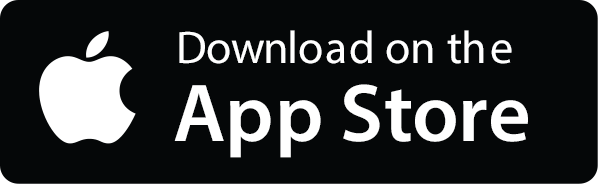
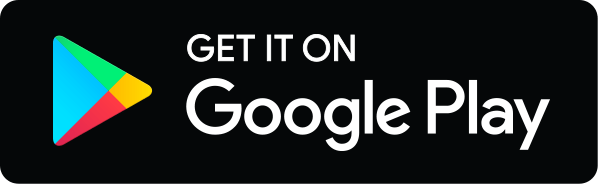