4 Biological Mechanisms of Lymphatic Spread Abstract The biological mechanisms of lymphatic spread are intricate, highly regulated active processes involving the concert of trophic factors, lymphatic growth, and tumor chemotaxis. Dissemination of head and neck cancer beyond its primary site has a significant detrimental impact on treatment outcomes with clinical neck disease decreasing survival by 50%. For years, tumor spread was considered a passive process dependent on tumor volume and interstitial pressure leading to tumor shedding into regional lymphatics. During the last few decades, however, the discovery of specific lymphatic immunohistochemical markers has enabled researchers to study the mechanisms in lymphangiogenesis and lymphatic spread in greater detail. These investigations have revealed that lymphatic spread instead involves highly active and regulated mechanisms. Although much remains to be elucidated, several of the most critical steps have been identified. The process begins with tumor lymphangiogenesis and the epithelial-to-mesenchymal transition of malignant cells. Through specific chemotactic signaling and extracellular matrix degradation, tumor cells intravasate into peritumoral lymphatics to reach the draining nodal basin. Although multiple trophic factors influence this sequence, the vascular endothelial growth factor C (VEGF-C)/VEGF-D/VEGFR-3 signaling axis has been shown to play a central role in the regulation of lymphatic spread. Intervention through downregulation of this axis has been identified as an attractive target for pharmacotherapy, preventing or limiting lymphatic dissemination. Although many agents have held early promise in preclinical animal experiments, human studies are just beginning. The exact role of these agents in the future remains to be seen. Keywords: lymphangiogenesis, lymphatic system, metastasis, vascular endothelial growth factor, epithelial-to-mesenchymal transition, chemotaxis For most cancer patients who succumb to their disease, it is not the result of an uncontrolled local tumor but from dissemination of cancer cells through lymphatic or hematogenous spread to regional nodes and distant organ systems. When these metastases have progressed far enough, they become resistant to conventional therapies. For certain tumors, including many sarcomas, renal cell carcinoma, and follicular thyroid carcinoma, early dissemination is typically hematogenous. For many others, however, including upper aerodigestive tract squamous cell carcinoma (SCC), initial spread is primarily to the regional lymphatics. While the reasons for these differences remain to be fully elucidated, the prognostic value of lymph node metastasis in head and neck cancer is substantial, decreasing survival probability by 50%.1 Although distant metastasis is an even more ominous prognostic sign, less than 10% of patients with head and neck cancer demonstrate overt distant metastatic disease at initial presentation. Given the propensity of head and neck cancer to develop lymphatic metastasis and the associated decrement in survival, a great deal of work has focused on the diagnosis and treatment of regional nodal disease. Patients who present with clinical regional metastasis are treated with conventional surgical or nonsurgical management paradigms. For patients who present with clinically negative regional nodes, however, management of the neck becomes more complicated. Neck dissection with pathological assessment of the resected nodal levels is the current “gold” standard staging tool for regional metastases in a clinically negative neck. This invasive procedure comes with risk, however, and is beneficial only if the probability of detecting occult regional disease is high enough. A significant proportion of clinically negative patients are also pathologically negative and will, therefore, undergo an unnecessary invasive staging procedure. Nonetheless, in the population of patients at approximately a 20% or greater risk of harboring occult metastasis, regional control and survival are overall improved in those undergoing lymphatic staging by neck dissection as compared to observation.2 There are no current widely accepted minimally invasive techniques to achieve accurate regional staging. Although sentinel lymph node biopsy has been used, it is limited in general to SCC of oral cavity subsites and is performed at specialized centers. It is still an invasive procedure, nonetheless, and comes with its own risks and challenges. Imaging techniques are limited in resolution, and positron emission tomography scanning can identify nodal involvement as low as 7 mm in diameter but cannot reliably detect occult micrometastatic disease.3 A predictive genetic or morphologic signature of a resected primary tumor would be ideal to determine the risk of occult metastasis and help better select patients for elective neck treatment or observation. Although molecular profiling of primary head and neck tumors has been investigated to identify genetic markers of lymphatic spread, none have been widely adopted or validated in large cohorts.4 Examining the biological mechanism of lymphatic spread, including trophic factors, signaling pathways, and morphological changes, provides another possible method to identify primary tumors at high risk for regional metastasis. This requires a detailed knowledge of how tumors spread to the regional lymphatics and may provide an opportunity to develop therapeutic agents to block regional dissemination of disease. This, in turn, necessitates an understanding of the structure and function of the lymphatic system, methods for microscopic assessment, and an overview of the major molecular mechanisms driving lymphatic spread. Nutrients in the bloodstream reach tissues by extravasation from capillary vessels under high pressures from the arterial system. This milieu of extravasated fluid and macromolecules makes up the interstitial fluid, allowing cells to take up these nutrients and dispose of waste products. Through both active and passive processes, this fluid and its components are recycled and returned to the circulation through the lymphatic system. Initial lymphatics are blind-ended channels composed of a single layer of endothelial cells with minimal basement membrane and large gap junctions to allow uptake of interstitial fluid. Anchoring filaments tether the initial lymphatics to the extracellular matrix to prevent vessels collapse under interstitial pressures. Lymph then proceeds into precollecting and larger collecting lymphatic vessels propelled by perivascular smooth muscle contraction along with extrinsic skeletal muscle pressure. These larger lymphatic vessels are less permeable, limiting extravasation, and one-way valves prevent lymphatic backflow. Lymph is first filtered through draining regional lymphatic basins, centers for antigen recognition, and initiation of any requisite immune response. From these lymphatics, lymph is ultimately returned to the venous circulation through the thoracic or right lymphatic ducts. An appreciation of lymphatic embryology can aid in understanding how tumor trophic factors may lead to lymphangiogenesis or invasion of existing host lymphatics. Due to difficulties with visualization and tracking of nascent lymphatics, development of the lymphatic system has been a subject of controversy for over 100 years. Although the exact origins of the lymphatic and venous systems have been debated, lymphatic development appears to begin through the formation of lymphatic sacs that sprout from the embryonic venous system as early as the fifth week of fetal development. These paired lymphatic sacs, which ultimately give rise to the thoracic and the right lymphatic ducts, expand and ramify into a lymphatic plexus. Lymph node formation follows as specialized myofibroblasts proliferate and form a scaffold for lymphoid tissue, through which immune cells are recruited. Although aspects of this process remain unclear, several key factors contributing to lymphatic development and proliferation have recently been identified. An early and critical developmental step, determining the fate of lymphatic endothelial cells, is expression of the transcription factor, Prospero-related homeobox protein 1 (Prox1). Prox1 knockout mice are devoid of lymphatics and deletion at any point during development results in lymphatic regression. Although the exact mechanisms that leads to induction of Prox1 expression remain unclear, bone morphogenic protein and Notch signaling pathways have been identified as inhibitors of Prox1-induced lymphangiogenesis and drive venous cell differentiation, while Wnt signaling appears to increase Prox1 expression in lymphatic progenitor cells. Certain transcription factors, as well, have been associated with induction of Prox1 signaling, including Sox18 and Coup-TF2. Ultimately, the Prox1 molecular switch results in expression of requisite proteins for lymphatic endothelial cell growth and migration.5 A significant advance in understanding lymphangiogenesis came with the discovery that vascular endothelial growth factors (VEGF) played a central role in stimulating lymphatic proliferation. These growth factors are composed of a family of subtypes, with five described members in mammals, including VEGF-A through VEGF-D and placental growth factor (PlGF). Each exerts its effects through specific membrane receptors coupled to downstream intracellular pathways. VEGF-A, VEGF-B, and PlGF are primarily involved in vasculogenesis and angiogenesis. VEGF-A was the first-described growth factor of this group and represents the dominant trophic signal for blood vessel endothelial cell proliferation and migration. VEGF-A binds to vascular endothelial growth factor receptors 1 and 2 (VEGFR-1 and VEGFR-2), although the majority of angiogenic effects are mediated through VEGFR-2. A third vascular endothelial growth factor receptor (VEGFR-3) was subsequently discovered, and its major ligands, VEGF-C and VEGF-D, were identified as a target of Prox1 activation and a key trophic axis for lymphatic proliferation. Early in embryogenesis, however, VEGFR-3 signaling is important for vascular development, and VEGFR-3 knockout mice die at an early embryonic stage of cardiovascular defects. Later in development, VEGFR-3 expression becomes restricted to lymphatics and is critical to lymphangiogenesis and lymphatic migration. VEGFR-3 heterozygous animals exhibit substantial lymphatic defects and similarly, the human condition Milroy’s disease is associated with a VEGFR-3 mutation and results in congenital lymphedema. Further, lymphatic sprouting is at least in part a VEGF-C-dependent process, as endothelial cells have been shown to migrate toward a VEGF-C gradient and VEGF-C-deficient mice develop a hypoplastic lymphatic system. Although VEGF-D is also a VEGFR-3 ligand and a known lymphangiogenic trophic factor, lesser congenital defects in lymphatic migration are seen in VEGF-D-deficient mice, including smaller caliber and less functional superficial lymphatics.5,6 A second EGF system, the angiopoietin–Tie (Ang-Tie) axis, is involved in angiogenesis and lymphangiogenesis as well. Angiopoietin 1 (Ang1) and 2 (Ang2) along with their receptors Tie1 and Tie2 are the best characterized members of this signaling pathway. Ang1 is an obligate agonist of Tie2, while the inhibitory or stimulatory effects of Ang2 are context dependent. Although the Ang-Tie system is primarily known for its role in vascular and cardiac embryology, it has been shown to be critical to the stability and remodeling of lymphatic endothelial cells later in development. As understanding of these pathways continues, the molecular picture becomes increasingly complex ( In addition, all these factors are closely regulated by extrinsic and intrinsic processes. VEGF-C is regulated by collagen and calcium-binding EGF domains 1 (CCBE-1), a protein necessary for normal lymphatic development in animal models, and mutations of which lead to lymphatic dysplasia in humans. CCBE-1 plays a role in proteolytic processing of VEGF-C to its most active form. Nrp-2 signaling, which results in lymphatic proliferation when binding VEGF-C, has been shown to be regulated by semaphorins, a family of signaling proteins. When binding Nrp2, class 3 semaphorins result in inhibition of lymphatic cell proliferation and induction of apoptosis. VEGFR-3 is also highly regulated by additional pathways independent of Prox1 signaling. Ephrin-B2, a transmembrane ligand for Eph receptor tyrosine kinases, promotes lymphatic sprouting through VEGFR-3 modulation. Similarly, T-box transcription factor 1 regulates VEGFR-3 expression and appears to be necessary for lymphatic endothelial cell growth and maintenance. Finally, VEGFR-3 may be activated independently of a VEGF ligand through binding of extracellular matrix components.8
4.1 Introduction
4.2 The Lymphatic System
4.2.1 Lymphatic Function
4.2.2 Lymphatic Origins and Development
Fig. 4.1). There appears to be crossover between the function of VEGFR-2 and VEGFR-3, including both homodimer and heterodimer formation leading to specific angiogenic and lymphangiogenic effects. VEGF-C is also known to bind the receptor neuropilin2 (Nrp2). Nrp2 is seen in high levels in the developing lymphatic plexus, and Nrp2-deficient mice exhibit normal central lymphatics but absent superficial lymphatic networks.7
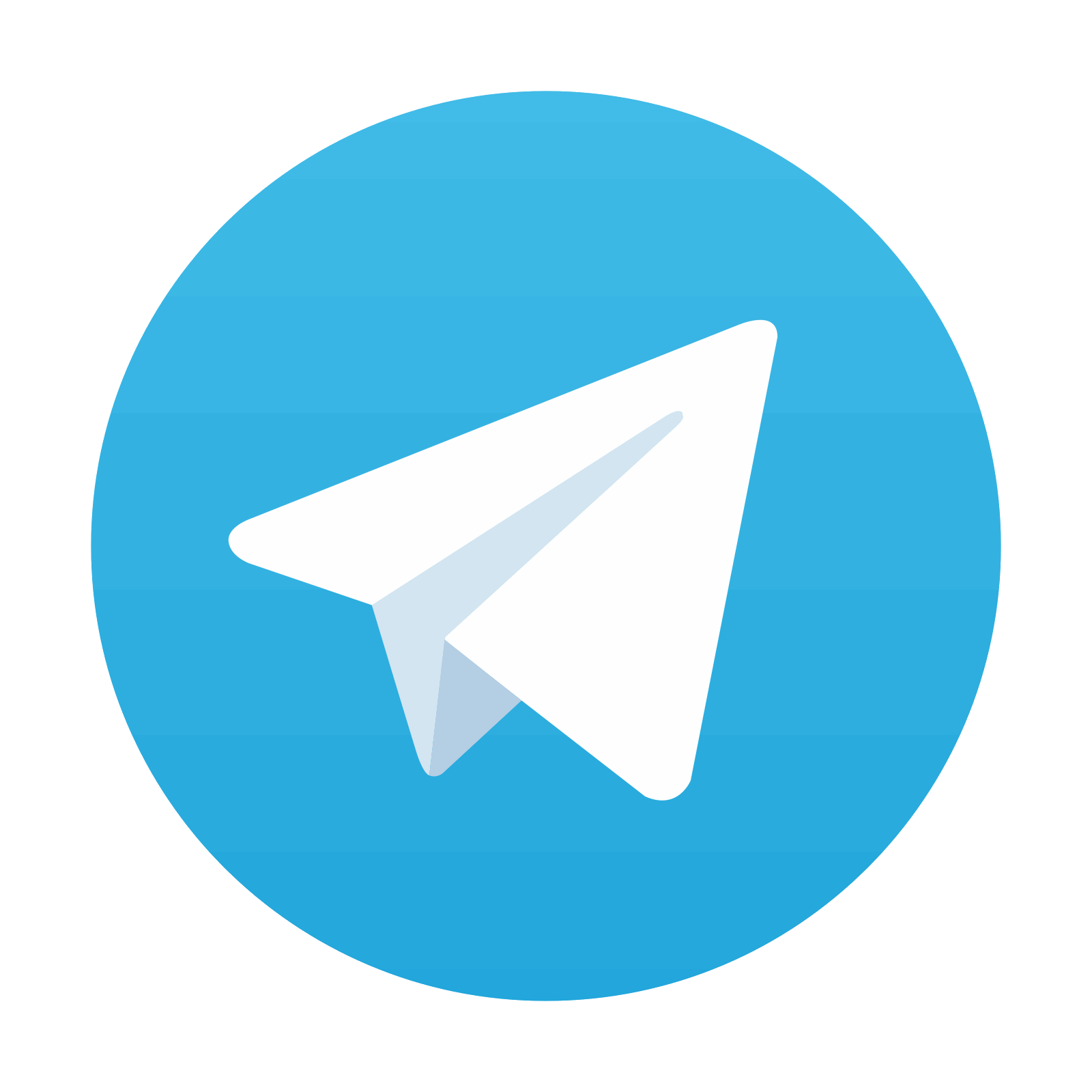
Stay updated, free articles. Join our Telegram channel

Full access? Get Clinical Tree
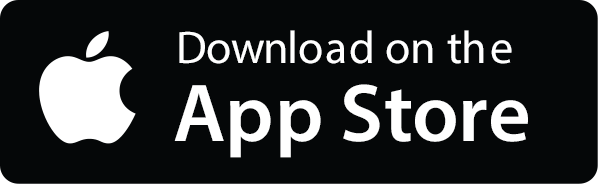
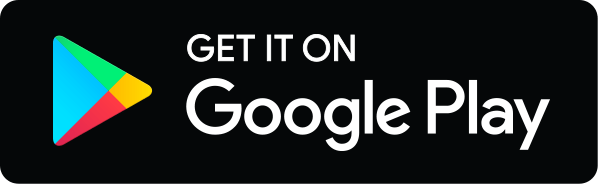