Sound: energy waves of particle displacement, both compression (more dense) and rarefaction (less dense) within an elastic medium; triggers sensation of hearing by vibrating the tympanic membrane.
Amplitude of sound: extent of vibratory movement from rest to farthest point from rest in compression and rarefaction phases of energy waves. In psycho acoustics, sounds with higher amplitudes are perceived as louder than sounds with lower amplitude.
Intensity of sound: amount of sound energy through an area per time; refers to sound strength or magnitude.
Sound pressure: sound force (related to acceleration) over a surface per unit time.
Decibel (dB): unit to express intensity of sound; more specifically the logarithm of the ratio of two sound intensities. One-tenth of a Bel (named for Alexander Graham Bell).
Frequency: number of cycles (complete oscillations) of a vibrating medium per unit of time; psychoacoustic correlate is pitch. Time for completion of one cycle is the period.
Hertz (Hz): in acoustics, unit to express frequency (formerly cycles per second or cps). Human ear capable of hearing from approximately 20 to 20,000 Hz.
Pure tone: single-frequency sound; rarely occurs in nature.
Complex sound: sound comprising more than one frequency.
Noise: aperiodic complex sound. Types of noise frequently used in clinical audiology are white noise (containing all frequencies in the audible spectrum at average equal amplitudes), narrow band noise (white noise with frequencies above and below a center frequency filtered out or reduced), and speech noise (white noise with frequencies > 3000 and < 300 Hz reduced by a filter). However, the term “noise” can also mean any unwanted sound.
Resonant frequency: frequency at which a mass vibrates with the least amount of external force; determined by elasticity, mass, and frictional characteristics of the medium. Natural resonance of external auditory canal is approximately 3000 Hz; of middle ear, 800 to 5000 Hz, mostly 1000 to 2000 Hz; of tympanic membrane, 800 to 1600 Hz; of ossicular chain, 500 to 2000 Hz.
The decibel scale is listed as follows:
A logarithmic expression of the ratio of two intensities.
Nonlinear (eg, the energy increase from 5-7 dB is far greater than the increase from 1-3 dB because it is a logarithmic scale).
A relative measure (ie, 0 dB does not indicate the absence of sound).
It is important to state a reference level when speaking of decibels because decibels are expressed with different reference levels, such as sound pressure level (SPL), hearing level (HL), and sensation level (SL).
The referent of SPL is the most common measure of sound strength.
Decibels SPL are currently usually referenced to micropascals (but can be referenced to dynes per centimeter squared or microbars).
Sound pressure is related to sound intensity.
The formula for determining the number of decibels is
dB Intensity = 10 log Io/Ir
where
Io = intensity of output sound being measured
Ir = intensity of reference
However, intensity is proportional to pressure squared, as
or
where
po = pressure of the output of sound being measured
pr = pressure of the reference, usually 20 µPa
Table 14-1 lists typical SPLs for various environmental noises.
Sound | Decibels (dB SPL) | |
---|---|---|
Rocket launching pad | 180 | Noises > 140 dB SPL may cause pain |
Jet plane | 140 | |
Gunshot blast | 140 | |
Riveting steel tank | 130 | |
Automobile horn | 120 | |
Sandblasting | 112 | |
Woodworking shop | 100 | Long exposure to noises > 90-dB SPL may eventually harm hearing |
Punch press | 100 | |
Boiler shop | 100 | |
Hydraulic press | 100 | |
Can manufacturing plant | 100 | |
Subway | 90 | |
Average factory | 80-90 | |
Computer printer | 85 | |
Noisy restaurant | 80 | |
Adding machine | 80 | |
Busy traffic | 75 | |
Conversational speech | 66 | |
Average home | 50 | |
Quiet office | 40 | |
Soft whisper | 30 |
When the reference is hearing level (HL).
0-dB HL at any frequency is the average lowest intensity perceived by subjects with normal hearing sensitivity 50% of the time.
The HL referent is to ears with normal hearing sensitivity (Table 14-2) and was developed because the ear is not equally sensitive to all frequencies. For the average human to just hear a 250 Hz sound, the sound must be 27 dB SPL, which is then set to 0-dB HL. The human ear is more sensitive to 1000 Hz, thus a SPL of 7.5 dB is sufficient for normal listeners to detect and is equivalent to 0-dB HL at 1000 Hz.
This scale takes into account differences in human sensitivity for the various frequencies: normal hearing is 0-dB HL across the frequency range rather than 47.5 dB SPL at 125 Hz, 26.5 dB SPL at 250 Hz, 13.5 dB SPL at 500 Hz, 7.5 dB SPL at 1000 Hz, and so on.
HL is the reference used on clinical audiometers.
When the reference is sensation level (SL).
The referent is to a specific individual’s threshold.
0- dB SL refers to the number of decibels above the threshold for that same stimulus.
For example, if a person has a threshold of 20-dB HL at 1000 Hz, and a 1000 Hz tone is presented at 50-dB SL for that individual, the tone would equal 70-dB HL.
The outer ear comprises the auricle or pinna, the external auditory canal or ear canal (it is ~1 inch or 2.5 cm in length and 1/4 inch in diameter, with an average volume of 2 cm3 in adults), and the outer surface of the tympanic membrane or eardrum.
The pinna is funnel shaped and collects sound waves, which are directed through the ear canal to create vibratory patterns on the eardrum.
The pinna also aids in the localization of sound, particularly vertically and amplifies high frequency over low-frequency sounds.
As a closed tube, the external auditory canal is a resonance chamber for the frequency region of 2000 to 5500 Hz. The average resonant frequency is approximately 2700 Hz but varies by individual ear canal.
The middle ear is an air-filled space approximately 5/8 inch high (15 mm), 1/8 to 3/16 inch wide (2-4 mm), 1/4 inch deep, and 1 to 2 cm3 in volume in the average adult.
Sound waves from the tympanic membrane travel along the ossicular chain, which comprises three bones (the malleus, incus, and stapes), to the oval window. The displacement of the ossicular chain varies as a function of the frequency and intensity of the sound.
The malleus and incus weigh approximately the same, but the stapes is about one-fourth the mass of the other ossicles. This difference facilitates the transmission of high frequencies.
The tympanic membrane and ossicular chain most efficiently transmit sound between 500 and 3000 Hz. Thus, the ear has greatest sensitivity at those frequencies most important to understanding speech.
The middle ear transforms acoustic energy from the medium of air to the medium of liquid. It is an impedance-matching system that ensures only minimal energy is lost, when structurally and functionally normal. This impedance matching is accomplished by the following four factors:
The area effect of the tympanic membrane: Although the area of the adult tympanic membrane is between 85 and 90 mm2, only about 55 mm2 effectively vibrates (the lower two-thirds of the tympanic membrane); the stapes footplate is 3.2 mm2. Thus, the ratio of the vibrating portion of the tympanic membrane to that of the stapes footplate results in a 17:1 increase in sound energy by concentrating it into a smaller area.
Lever action of the ossicular chain: As the eardrum vibrates, the ossicular chain is set into motion about an axis of rotation from the anterior process of the malleus through the short process of the incus. Because the handle of the malleus is approximately 1.3 times longer than the incus long process, the force (pressure) received at the stapes footplate, through the use of leverage, is greater than that at the malleus by about 1.3:1. Thus, the transformer ratio of the middle ear is approximately 22:1 (the combination of the area effect of the tympanic membrane and the lever action of the ossicles: 17 × 1.3 = 22) which translates to approximately 25 dB.
The natural resonance and efficiency of the outer and middle ears: Enhances sound transmission for 500 to 3000 Hz.
The phase difference between the oval window and the round window: When sound energy impinges on the oval window, a traveling wave is created within the cochlea progressing from the oval window, along the scala vestibuli and the scala tympani, to the round window. The phase difference between the two windows results in a small change (~4 dB) in the normal ear.
Once the sound signal impinges on the oval window, the cochlea transforms the signal from mechanical energy into hydraulic energy and then ultimately, at the hair cells, into bioelectric energy. As the footplate of the stapes moves in and out of the oval window, a traveling wave is created in the cochlea (Bekesy’s traveling wave theory). As the wave travels through the cochlea, it moves the basilar and tectorial membranes. Because these two membranes have different hinge points, this movement results in a “shearing” motion that bends the hair cell stereocilia. This bending depolarizes the hair cells, which, in turn, activates afferent electrical nerve impulses.
The energy wave travels from base to apex along the basilar membrane until the wave reaches a maximum. The basilar membrane varies in stiffness and mass throughout its length. The point of maximum displacement of the traveling wave is determined by the interaction of the frequency of the sound and the basilar membrane’s physical properties. The outer hair cells (OHCs) are motile, reacting mechanically to the incoming signal by shortening and lengthening according to their characteristic (best) frequency. Under strong efferent influence, the OHCs are part of an active feedback mechanism, adjusting the basilar membrane’s physical properties so that a given frequency maximally stimulates a specific narrow group of inner hair cells (IHCs). This effect is the “cochlear amplifier.” The IHCs trigger the preponderance of afferent nerve responses; 95% of all afferent fibers innervate the IHCs.
The cochlea is organized spatially according to frequency, that is, tonotopic arrangement. For every frequency there is a highly specific place on the basilar membrane where hair cells are maximally sensitive to that frequency, the basal end for high frequencies, and the apical end for low frequencies. In the intact auditory system, this tonotopic mapping is maintained as the neural code is transmitted from the hair cells through the auditory system. For sounds comprising multiple frequencies (complex sound), there are several points of traveling wave maxima, and the cochlear apparatus constantly tunes itself for best reception and encoding of each component frequency. The auditory mechanism’s superb frequency resolution is mostly secondary to the highly tuned hair cell response rather than on processing at higher auditory centers.
The cochlea is nonlinear, acting like a compression circuit by reducing a large range of acoustic inputs into a much smaller range. The compression mainly occurs around the OHC’s characteristic frequency. This nonlinearity allows the auditory system to process a wide range of intensities, which is represented by the nonlinear, logarithmic decibel scale. The perception of pitch and loudness is based on complex processes from the outer ear up through the higher auditory centers. However, the major factor is the periphery, where the cochlea acts as both a transducer and analyzer of input frequency and intensity.
Labyrinthine biochemistry is an ongoing area of research but Tables 14-3 and 14-4 provide basic information.
Perilymph | Endolymph | ||||||
---|---|---|---|---|---|---|---|
Serum | CSF | Scala tympani | Scala vestibuli | Cochlea | Vestibule | Endolymphatic sac | |
Na (mEq/L) | 141 | 141 | 157 | 147 | 6 | 14.9 | 153 |
K (mEq/L) | 5 | 3 | 3.8 | 10.5 | 171 | 155 | 8 |
Cl (mEq/L) | 101 | 126 | — | — | 120 | 120 | — |
Protein (mg/dL) | 7000 | 10-25 | 215 | 160 | 125 | — | 5200 |
Glucose (mg/dL) | 100 | 70 | 85 | 92 | 9.5 | 39.4 | — |
pH | 7.35 | 7.35 | 7.2 | 7.2 | 7.5 | 7.5 | — |
Once nerve impulses are initiated, signals continue along the auditory pathway from the spiral ganglion cells within the cochlea to the modiolus, where the fibers form the cochlear branch of the eighth nerve. The fibers pass to the cochlear nucleus at the pontomedullary junction of the brain stem, the first truly central connection. The fibers and nucleus are tonotopically organized. All fibers synapse at the ipsilateral cochlear nucleus. The majority of fibers cross through the acoustic stria and trapezoid body to the contralateral superior olivary complex in the lower pons of the brain stem. This crossing is the first point of decussation where signals from both ears interact to allow binaural function. Fibers ascend to the nuclei of the lateral lemniscus in the pons and to the inferior colliculus in the midbrain. The medial geniculate body in the thalamus is the last auditory nucleus before the cortex. From there, the nerve fibers radiate to the auditory cortex. Tonotopic organization is largely maintained throughout the auditory pathway from the cochlea to the cortex.
Multiple pathways exist in the auditory central nervous system with several crossovers and nuclei. Not all neuronal tracts synapse with each auditory nucleus sequentially in a “domino” fashion but rather may comprise two to five synapses. There is a proliferation of fibers ranging from about 25,000 in the eighth nerve to millions from the thalamus. In addition to the various nuclei, there are afferent and efferent fibers, all exerting a mutual influence on one another. It would be an enormous task to examine all of the possible pathways, nuclei, and processing involved in this neural transmission. A mnemonic for the general simplified sequence of these auditory structures is ECOLI: Eighth nerve, Cochlear nucleus, Olivary complex, Lateral lemniscus, and Inferior colliculus. However, these pathways with sequential and parallel processing are complex and still active areas of ongoing research.
Standard part of the otologic examination.
Can provide useful screening and confirmatory information, but do not replace audiometric test results.
The most useful fork is the 512-Hz fork, but 1024 Hz and 2048 Hz may also be used.
Typically, tuning forks for frequencies below 512 Hz, such as 128 or 256-Hz, are not used because the low-frequency vibrations may be felt rather than heard. This somatosensory stimulation may confuse the patient and compromise findings.
It is essential to strike the fork gently to avoid creating overtones.
See Table 14-5 for a summary of these tests.
Test | Purpose | Fork Placement | Normal Hearing | Conductive Loss | Sensorineural Loss |
---|---|---|---|---|---|
Weber | To determine conductive versus sensorineural loss in unilateral loss | Midline | Midline sensation; tone heard equally in both ears | Tone louder in poorer ear | Tone louder in better ear |
Rinne | To compare patient’s air and bone conduction hearing | Alternately between patient’s mastoid and entrance to ear canal | Positive Rinne: tone louder at ear | Negative Rinne: tone louder on mastoid | Positive Rinne: tone louder by ear |
Bing | To determine if the occlusion effect is present | Mastoid | Positive Bing: tone is louder with ear canal occluded | Negative Bing: tone is not louder with ear canal occluded | Positive Bing: tone is louder with ear canal occluded |
Schwabach | To compare patient’s bone conduction to that of a person with normal hearing | Mastoid | Normal Schwabach: patient hears tone for about as long as the tester | Prolonged Schwabach: patient hears tone for longer time than the tester | Diminished Schwabach: patient stops hearing the tone before the tester |
Purpose: The Weber test is a test of lateralization.
Procedure: The tuning fork is set into motion and its stem is placed on the midline of the patient’s skull. The patient must state where the tone is louder: in the left ear, in the right ear, or at midline.
Interpretation: If the patient perceives the sound
At midline = normal hearing or equal amounts of hearing loss in both ears
In the better ear = unilateral sensorineural loss
In the poorer ear = unilateral conductive loss
Purpose: The Rinne test compares a patient’s air and bone conduction hearing to determine whether a major conductive component, or air–bone gap, is present.
Procedure: The tuning fork is struck and its stem placed first on the mastoid process (as closely as possible to the posterosuperior edge of the canal without touching it), then approximately 2 inch (5 cm) lateral to the opening of the external ear canal. The patient reports whether the tone sounds louder with the fork on the mastoid or just outside the ear canal.
Interpretation:
Positive Rinne: tone is louder outside the ear canal (AC > BC) = suggests normal hearing or sensorineural hearing loss
Negative Rinne: tone is louder when placed on the mastoid (BC > AC) = suggests conductive component of approximately 20 dB or greater to the hearing loss
Purpose: The Bing test is not as commonly conducted as the Weber or Rinne, but uses presence or absence of the occlusion effect to screen for possible conductive pathology.
Procedure: The tuning fork is set into motion and its stem placed on the mastoid process behind the ear while the tester alternately opens and closes the patient’s ear canal with a finger.
Interpretation:
Positive Bing: if occlusion effect is present, the tone becomes louder when the canal is closed and softer when it is open = suggests normal hearing or mild sensorineural hearing loss
Negative Bing: no occlusion effect/no change in the tone’s loudness as the ear canal is opened and closed = suggests conductive component to the hearing loss
Purpose: The Schwabach test compares the patient’s bone conduction hearing to that of a normal listener (usually the examiner). This test is not commonly conducted and requires that the hearing of the examiner/normal listener be confirmed as normal.
Procedure: The tuning fork is set into motion and its stem placed alternately on the mastoid process of the patient and that of the examiner. When the patient no longer hears the sound, the examiner listens to the fork to see whether the tone is still audible.
Interpretation:
Normal Schwabach: patient stops hearing the sound at about the same time as the tester = normal hearing
Diminished Schwabach: patient stops hearing the sound before the examiner = sensorineural hearing loss
Prolonged Schwabach: patient hears the sound longer than the examiner = conductive component to the hearing loss
Audiometer to test hearing for pure-tone thresholds and speech recognition
Immittance analyzer to assess middle ear function and acoustic reflexes
A sound-isolated or acoustically treated room adequate for measuring 0-dB HL thresholds by air and by bone conduction, preferably meeting ANSI S3.1.
The main controls on a diagnostic audiometer include the following:
Stimulus selector (pure tone; warbled tone; pulsed or alternating tones; narrow band, white, or speech noise; microphone; digital speech; external sound source, such as CD/DVD)
Output selector (right, left, or both sides; type of transducer, which may include supra-aural earphones, circumaural earphones, insert earphones, high-frequency earphones, bone oscillator, sound-field speakers)
Frequency selector (125-8000 Hz) or up to 20,000 Hz for high-frequency audiometry
Attenuator control (–10 to +120 dB HL) with maximum intensity limit indicator. The maximum intensity will vary by audiometer. Further, for each audiometer the maximum intensity varies by frequency and transducer.
Stimulus presentation mode selector to designate the stimulus to be either continuously on until interrupted or off until presentation is selected
Interrupter control to present or interrupt the stimulus
Volume unit (VU) meter to monitor presentation level of speech or external signal
Adjustment controls to maintain proper input and output levels of internally generated stimuli as well as stimuli from external devices connected to the audiometer, such as CD players
Talk forward controls enabling one to speak to the patient at a comfortable, adjustable intensity level without requiring the microphone mode
Talk back controls enabling one to hear the patient from the booth at a comfortable, adjustable level
The immittance analyzer usually has the following minimum components:
Probe tip with multiple removable tips of various sizes to achieve a hermetic seal in a variety of ear canals
Selection of probe frequency to allow for appropriate assessment of adult and pediatric patients
Frequency selector (for acoustic reflex and reflex decay testing)
Intensity selector (for acoustic reflex and reflex decay testing)
Probe or supra-aural/insert earphone (for stimulating the contralateral ear reflex)
Pressure control (to increase or decrease the pressure in the ear canal during tympanometry)
For all audiometric equipment, testing will be valid only if the equipment used is appropriate and calibrated. Therefore, selection and maintenance of equipment, including care in use and at least annual comprehensive calibrations, are vital. In addition, the examiner should complete a biologic check, including listening to stimuli, regularly before use.
The purposes of the basic audiologic evaluation are to determine the following:
Presence or absence of normal responses. If responses are not within normal limits:
Characterization of hearing sensitivity
– Degree of decreased hearing sensitivity
– Configuration of hearing responses
– Site of lesion (conductive, sensorineural, mixed, or neural/auditory dyssynchrony)
Recommended management
– Need for medical or surgical management
– Possible nonsurgical intervention, such as hearing aids, assistive devices, aural (re)habilitation, speech-reading, communication strategies
Need for further testing
Acquisition of information about multiple sites in the auditory pathway; ideally will include objective physiologic assessment and functional evaluation.
Allows cross-checking results to judge reliability and validity. This approach is particularly important when assessing special populations, such as infants and young children, who may not be able to provide consistent or comprehensive behavioral information.
Inconsistencies may be secondary to pseudohypacusis (nonorganic hearing loss), an inattentive or uncooperative patient, or a patient who does not understand the instructions. Results of a single test must be interpreted with caution.
A typical test battery for an audiologic evaluation includes the following:
Pure-tone audiometry to assess frequency specific hearing sensitivity (air conduction, and, if needed, bone conduction)
Speech audiometry (speech reception threshold and word recognition score)
Immittance/impedance measures (tympanometry and acoustic reflexes)
Pure-tone audiometry is the foundation of audiometric testing. Thus, its reliability and validity are paramount. Influencing factors include the following:
Test location (quiet enough to measure 0-dB HL thresholds; a sound-treated booth is usually required for both examiner and patient rooms)
Equipment calibration (complete calibration at least annually with routine clinical verification of equipment function)
Properly trained personnel (preferably a licensed audiologist)
Clear and neurodevelopmentally appropriate instructions
Otoscopic inspection
Proper selection and placement of earphones and/or bone oscillator
Patient comfort
Test of sensitivity to pure tones as measured by air conduction; and by bone conduction if air-conduction thresholds are 15-dB HL or greater.
Determines thresholds: lowest levels at which patient responds at least 50% of time
Octave frequencies 250 to 8000 Hz; inter-octave frequencies (eg, 1500 Hz) if 25 dB or more difference between octave frequency thresholds.
3000 and 6000 Hz are also typically acquired for baseline audiograms, for example, persons exposed to high-intensity sound or receiving ototoxic medication, and for fitting of amplification.
Noise introduced into nontest ear to prevent that ear from detecting signals intended for test ear.
Necessary when signal to test ear is strong enough to stimulate the nontest ear by sending vibrations through the skull: crossover.
Reduction in sound energy as it travels from one side of the skull to other is interaural attenuation.
The amount of interaural attenuation varies by individual due to characteristics of the skull. However, in general, interaural attenuation for air conduction with supra-aural headphones is 40 to 65 dB depending on frequency and patient characteristics; interaural attenuation for insert earphones occurs at higher intensities and can be over 70 dB at some frequencies. Interaural attenuation for bone conduction is 0 to 10 dB.
Crossover in air conduction with supra-aural earphones can occur as low as 40-dB HL and in bone conduction as low as 0-dB HL.
The preferred masker used for assessing threshold for pure tones is narrow-band noise centered around the frequency being tested; for speech, the preferred masker is speech-spectrum noise.
Insert earphones allow much higher interaural attenuation, and thus there is much less chance of crossover. Interaural attenuation may be as high as 70 to 90 dB, which often eliminates the need for masking during air-conduction testing.
Air conduction: Mask the nontest ear whenever the air-conduction stimulus level to the test ear exceeds the bone conduction threshold of the nontest ear by 40 dB or more for supra-aural earphones and 70 dB or more for insert earphones (see examples in Figure 14-1).
Bone conduction: Mask the nontest ear whenever there is an air–bone gap greater than 10 dB in the test ear.
Figure 14-1
Examples of applying the rules that determine the need for masking and the results after having masked. A. Right air-conduction threshold, unmasked, is 45 dB poorer than left bone conduction threshold and must be verified by masking. B. Right air-conduction threshold masked (no change with masking). C. Right bone conduction threshold, unmasked, shows an air–bone gap greater than 10 dB. D. Right bone conduction threshold, masked (shifted with masking).

Air conduction: earphones—hearing sensitivity is assessed from the ear canal to the brain stem
Bone conduction: bone oscillator on mastoid or forehead—hearing sensitivity is assessed only from the cochlea to the brain stem; bypasses outer and middle ears
Bone conduction results show degree of hearing loss due to inner ear, nerve or central damage; air-conduction results show degree of hearing loss due to disruption of sound transmission at any level of the auditory system resulting in a conductive, sensorineural or mixed disorder. The difference between air conduction and bone conduction findings, or air–bone gap, reflects the contribution of reduced transmission or conduction of sound through outer and/or middle ears to decreased hearing sensitivity.
Thresholds for air and bone conduction are recorded on an audiogram, a graphic representation of a person’s sensitivity to pure tones as a function of frequency. For each frequency, indicated by numbers across the top of the audiogram, the individual’s threshold in decibels HL (dB HL), indicated by numbers along the side of the audiogram, is plotted where the two numbers intersect. The most commonly used audiogram symbols are shown in Table 14-6.
Pure-tone testing yields one of several audiogram types:
Normal hearing: All air-conduction thresholds in both ears are within normal limits (≤ 25-dB HL for adults and ≤ 15-dB HL for children; Figure 14-2).
Conductive hearing loss: Hearing loss only by air conduction, with normal bone conduction thresholds, indicating outer or middle ear pathology impairing sound transmission to the inner ear (Figure 14-3).
Sensorineural hearing loss: Hearing loss by air and by bone conduction of similar degree, indicating pathology of the cochlea (sensory), nerve (neural), and/or auditory central nervous system (neural) (Figure 14-4).
Mixed hearing loss: Hearing loss by both air and bone conduction, but air-conduction hearing is worse than bone conduction, indicating a conductive pathology overlaid on sensorineural pathology (Figure 14-5).
When describing a hearing loss plotted on an audiogram, configuration of the loss is important information for determining possible etiology and functional impact on speech perception and aural performance. The audiogram may be
Flat (see Figure 14-5)
Rising (see Figure 14-3)
Sloping (see Figure 14-4)
Falling (Figure 14-6)
Notched (Figure 14-7)
Saucer-shaped (Figure 14-8)
Thus, pure-tone air and bone conduction threshold testing provides a profile of an individual’s hearing sensitivity to the frequencies tested. For more information about the integrity of the auditory system, pure-tone results should be interpreted in conjunction with speech audiometry, tympanometry, and acoustic reflex test results.
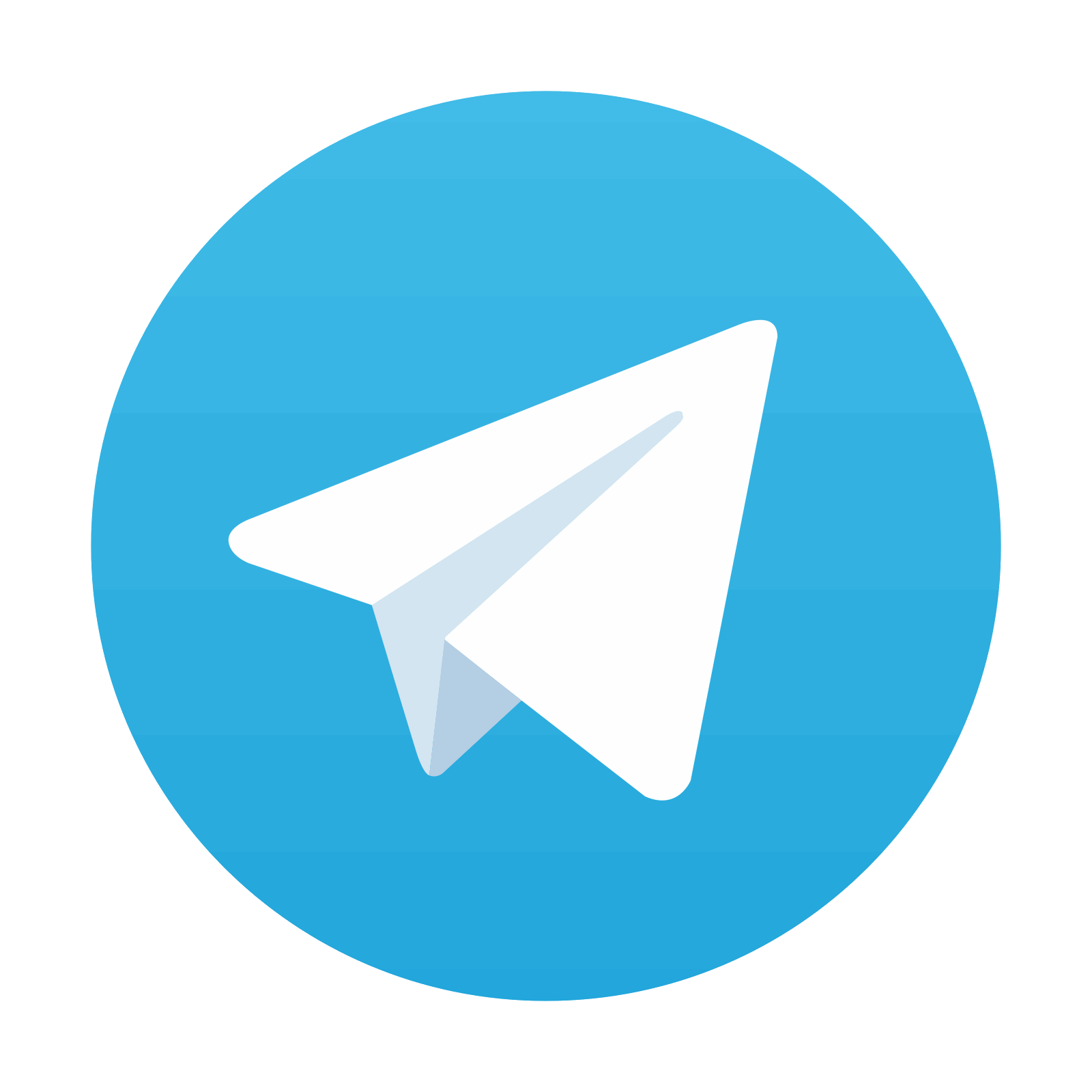
Stay updated, free articles. Join our Telegram channel

Full access? Get Clinical Tree
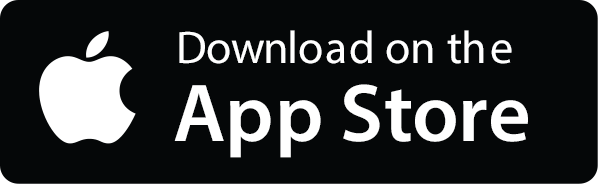
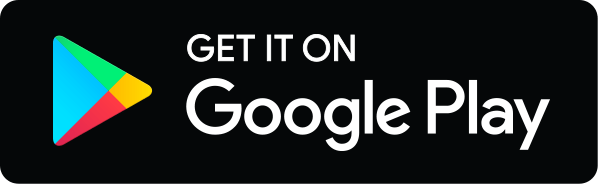
