A simple overview of lung function is the intention of this chapter. It is meant to outline basic aspects of respiratory physiology and function, but as with any organ system it is but the tip of the iceberg. The following information is meant as a basic introduction as well as a stimulus for the curious to dig deeper.
Lung volumes can be divided into primary volumes and capacities.
Primary volumes
Tidal volume (TV): The volume of gas that is either inspired or expired during each normal respiratory cycle
Residual volume: The amount of gas that remains in the lungs at the end of a maximal expiratory effort
Capacities
Total lung capacity: The amount of gas contained in the lungs at the end of a maximal inspiratory effort
Vital capacity: The maximum volume of gas exhaled when a patient makes a forceful exhalation after inspiring to the total lung capacity
Functional residual capacity: The volume of gas that remains in the lungs at the end of quiet exhalation
Dynamic lung volumes are as follows:
Forced expiratory volume in 1 second (FEV1): The volume of gas exhaled from the lung after initiation of a forceful exhalation following a maximal inspiration
Forced expiratory volume in 1 second/forced vital capacity (FEV1/FVC) ratio: The ratio of the volume of gas exhaled from the lungs during the first second after forceful exhalation divided by the total volume of gas exhaled after forceful exhalation
Despite being simple, Figure 7-1 is an excellent way to understand the various lung volumes and capacities.
There are three points of reference: (1) total lung capacity which is at the point of maximal inspiratory effort; (2) the total volume at maximal voluntary expiration, or residual volume; and (3) the volume at the end of passive expiration, or functional residual capacity.
This appears to be best reinforced and understood if one performs the maneuvers that are involved in achieving the volumes and capacities.
The lung volumes should be viewed in the context of two opposing forces that are seeking to expand or retract the lung.
The spirometer is widely used in pulmonary function laboratories because it has a nitrogen or helium analyzer, which allows the physician to obtain data concerning lung volumes, capacities, and dynamic lung volumes. By analyzing data obtained with a spirometer, the physician is able to determine whether a patient has normal or abnormal lung function. In addition, the spirometer enables the physician to assess the abnormalities of function and place the individual into one of two major pulmonary disease categories: chronic airflow limitation (diseases such as asthma, chronic bronchitis, and pulmonary emphysema) or restrictive lung disease (diseases such as pulmonary fibrosis).
The maximal expiratory flow volume curve has been widely used by pulmonary laboratories for the past several years. In a spirometer tracing, volume is plotted against time (FEV1 is the volume of gas exhaled during the first second after exhalation from a maximal inspiration). If the volume-time relation is normal, the flow rate is presumed to be normal; flow is never actually measured. In the flow volume loop, however, instantaneous flow is measured by means of a pneumotachograph, and flow is plotted against lung volume. Conditions that produce airflow limitation cause a reduction in measured flow rates throughout the patient’s FVC maneuver.
Figure 7-2 illustrates a normal flow volume loop and a flow volume loop from a patient with chronic airflow limitation (such as chronic obstructive pulmonary disease [COPD]). Note that the shape of the curve is concave in the normal patient but convex in the patient with limited flow. Note also that the flow volume loop characterizes the relations between flow and volume during the inspiratory portion of the respiratory cycle. The uses of this portion of the curve are discussed in a subsequent section of this chapter.
As with patients whose spirometry tracings indicate airflow limitation, bronchodilator medication is administered to patients with abnormal flow volume loops compatible with airflow limitation. Patients with reversible disease demonstrate a 15% to 20% improvement in the flow volume loop after bronchodilator administration. Patients with restrictive lung diseases have abnormal flow volume loops, but because they do not have an abnormality of the airways, their FEV1/FVC ratio is usually normal. The slope of the expiratory loop in these patients is normal, in keeping with their normal airways.
As noted, the flow volume curve can be used to evaluate inspiration as well as exhalation. This feature enables the pulmonary physiologist to evaluate the upper airway and assess the presence or absence of upper airway obstruction; this can also be a useful test for an otolaryngologist.
The ability of the flow volume loop to detect upper airway obstruction is based on the following physiologic principle: on inspiration, pleural pressure becomes more negative than the intraluminal pressure in the intrathoracic airways.
Consequently, on inspiration the caliber of the airways located within the chest increases. In the extrathoracic airways, such as the trachea, however, inspiration leads to a reduction in the intraluminal pressure, making atmospheric pressure greater than the pressure within the tracheal lumen. As a result, during inspiration the caliber of the tracheal lumen tends to diminish because atmospheric pressure exceeds intratracheal pressure.
In patients with variable extrathoracic (upper airway) obstruction, the obstruction tends to narrow the tracheal lumen. On inspiration, this narrowing becomes more pronounced by the effect of inspiration, which also causes a reduction in the size of the tracheal lumen. Hence, on inspiration, patients with variably obstructing lesions of the upper airway show a reduction in inspiratory flow, so that the inspiratory curve often appears flattened. On exhalation, intratracheal pressure becomes greater than atmospheric pressure, which causes the tracheal lumen to expand. This expansion tends to negate the effect of a variably obstructing tracheal lesion, and the expiratory portion of the flow volume curve appears normal even if there is a variably obstructing lesion present. Thus, in a patient with a variably obstructing lesion of the upper airway, the inspiratory curve is flattened whereas the expiratory curve appears normal. Figure 7-3 illustrates the curve produced by variably obstructing extrathoracic (upper airway) lesions.
The compliance of the lung refers to the elastic properties of that organ. Compliance is a measure of the distensibility, or elasticity, of the lung parenchyma. Many physicians are confused by the term elasticity. Elasticity refers to the ability of a structure to resist deformation. A rubber band is often referred to as an “elastic band”; it is an elastic structure not because it can be stretched but because it reverts to its original length when released. Hence, elasticity is the property whereby the original shape is preserved.
Distensibility, on the other hand, is the ease with which shape can be altered. An elastic structure is not distensible, whereas a distensible structure does not possess elastic qualities. In the lung, distensibility refers to the ease with which changes in distending pressure change lung volume. A lung in which small distending pressures produce large changes in volume is a highly distensible (or highly compliant) lung. A lung in which high distending pressures are required to produce even small changes in lung volume is poorly distensible and poorly compliant. It is also a highly elastic (or “stiff”) lung. Clinical examples are (1) the emphysematous lung, which is highly distensible, highly compliant, and poorly elastic secondary to the destruction of the elastic structures of the lung and (2) the fibrotic lung, which is poorly compliant, poorly distensible, and very elastic or stiff owing to the increased deposition of collagen. Figure 7-4 illustrates compliance curves in patients with normal, highly compliant (emphysematous), or “stiff” (fibrotic) lungs.
The diffusing capacity refers to the quantity of a specific gas that diffuses across the alveolar-capillary membrane per unit of time. The diffusing capacity is often used to assess the size of the pulmonary capillary blood volume. A full discussion of the methods employed to measure the diffusing capacity is beyond the scope of this text. In most pulmonary function laboratories, carbon monoxide is used to measure the diffusing capacity. This gas avidly binds to hemoglobin. In clinical practice, the diffusing capacity is thought to represent the volume of capillary blood into which carbon monoxide can dissolve. Diseases such as emphysema, which are characterized by a reduction in capillary blood volume, are associated with a low diffusing capacity.
A simple test that measures a patient’s exercise capacity and essentially measures the distance walked in 6 minutes. The more elaborate test is a cardiopulmonary stress test, which involves measure of exhaled gases and measures of oxygen consumption. It should be noted that there is a good correlation between the 6-minute test and the cardiopulmonary stress test and in patients with COPD has better correlation than the conventionally measured FEV1. It again emphasizes the need to incorporate the muscle pump or bellows into our understanding of the respiratory system.
The appreciation of the lungs as an integral part of our immune mechanisms has been recognized since the late 18th century, but suffice it to say we have made some progress in the understanding. The alveolar macrophage is the effector cell in controlling response to pathogens, but given the ongoing exposure of the alveolar membrane to these triggers the mechanism of control of the response to triggers is equally important.
Drug-induced pulmonary toxicity is an area that may offer an insight into mechanisms that balance the processing of stimuli and mediating the response which could be as damaging.
It bears mentioning that the most interwoven problems relate to the symptom of cough. Not only does sinonasal disease and asthma commonly serve as etiology of a cough that persists greater than an 8-week duration, but there are numerous other associations.
Difficult-to-treat asthma will commonly be related to unrecognized sinonasal disease.
The chronicity of both sinonasal disease and persistent asthma syndromes sometimes may lead to a cough that may develop an independent self-perpetuating process.
Vocal cord dysfunction as the cause of cough will pose a significant clinical challenge to both ENT and pulmonary physicians.
Postulates about the association of sinusitis and asthma include the following:
Bacterial seeding
Enhanced beta-blockade
Nasobronchial reflex
Eosinophil activation
Simple nasal obstruction
If one works through the algorithm of cough management and difficult-to-treat asthma, it is easy to appreciate the interactions between asthma, sinonasal disease, and gastroesophageal reflux disease. The three disease states and the use of angiotensin-converting enzyme inhibitors will be the causative etiology of a cough that persists for greater than 8 weeks in the setting of a normal chest x-ray. A third of the time more than one etiology will be responsible.
The concept or understanding of the factors that alter a patient’s work of breathing and also the sensation of dyspnea is one aspect of respiratory physiology that continues to be better understood. However, one should approach it with the knowledge that we are still learning.
Consider the example of the test used to assess a patient’s ability to be weaned from mechanical ventilation to outline some of the mechanisms. When a patient has been on ventilator support, the assessment for potential exhaustibility is assessed with a spontaneous breathing trial.
If the patient has a “rapid shallow breathing index” (RSBI) of less than 105 over a 30-minute period, he or she has an 85% chance of successful extubation. The RSBI is the frequency of breathing divided by the tidal volume measured in liters. The tachypnea as an indication of failure appears to be intuitive, but the reason for the tachypnea is not. The tachypnea is related to the assessment of the load per breath as a ratio of the maximal sustainable load, and the respiratory center adopts rapid shallow breathing as a protective mode to prevent respiratory muscle fatigue.
A real-life analogy would be to think of a distance runner who works his pace up to a point and then reduces it to “cruising” at which time there is tolerance of respiratory effort that will not cause immediate fatigue. This phenomenon is a contributing factor to what is referred to as a second wind.
This becomes a clinical issue in the elderly patient with common comorbidities of pulmonary disease, cardiac disease, and obesity, where there is commonly an attendant component of deconditioning. This reduces the denominator, that is, maximum work load, and hence increases dyspnea.
The mechanism of dyspnea in various disease states is beyond the scope of this review.
Alveolar ventilation refers to the volume of gas in each breath that participates in gas exchange multiplied by the respiratory rate. Alveolar ventilation determines the level of arterial carbon dioxide; in the clinical setting, the adequacy of alveolar ventilation is assessed by measuring the arterial partial pressure of carbon dioxide (PCO2).
In clinical practice, the most common causes of hypoxemia are simple hypoventilation and ventilation-perfusion inequality. Other causes of hypoxemia include anatomic shunts and abnormalities of diffusion, but these problems are rarely found in clinical hypoxemia.
The otolaryngologist is often confronted with a patient whose blood gas measurements reveal hypoxemia, and it is important that the attending physician be able to distinguish patients who have intrinsic pulmonary disease from those with simple hypoventilation (such as may be produced by anesthetic administration) and normal lungs. One useful technique for evaluating the presence or absence of intrinsic lung disease is the determination of the alveolar-arterial (A-a) gradient. A simple way to calculate the A-a gradient is to assume that the alveolar oxygen tension is 148-arterial PCO2 × 1.2. If the alveolar oxygen tension is calculated and the arterial PO2 measured, the A-a gradient can be estimated. If there is less than a 20 mm Hg gradient between alveolar and arterial oxygen tensions, it is likely that the lungs are normal and that alveolar hypoventilation is the sole abnormality producing the hypoxemia. Patients with normal lungs who have primary alveolar hypoventilation exhibit normal oxygen tensions when the cause of the alveolar hypoventilation is removed. A patient with a sedative overdose has normal oxygenation when the effects of the sedative on respiratory drive wear off.
Diseases that produce widened A-a gradients produce hypoxemia that cannot be corrected by simply increasing the level of alveolar ventilation. As stated, the most common cause of hypoxemia in these patients is maldistribution of alveolar ventilation and pulmonary blood flow. Diseases such as asthma, bronchitis, and emphysema impair ventilation because of abnormal airway flow. The reduction in flow produces abnormal ventilation-blood flow relations, which creates the observed hypoxemia.
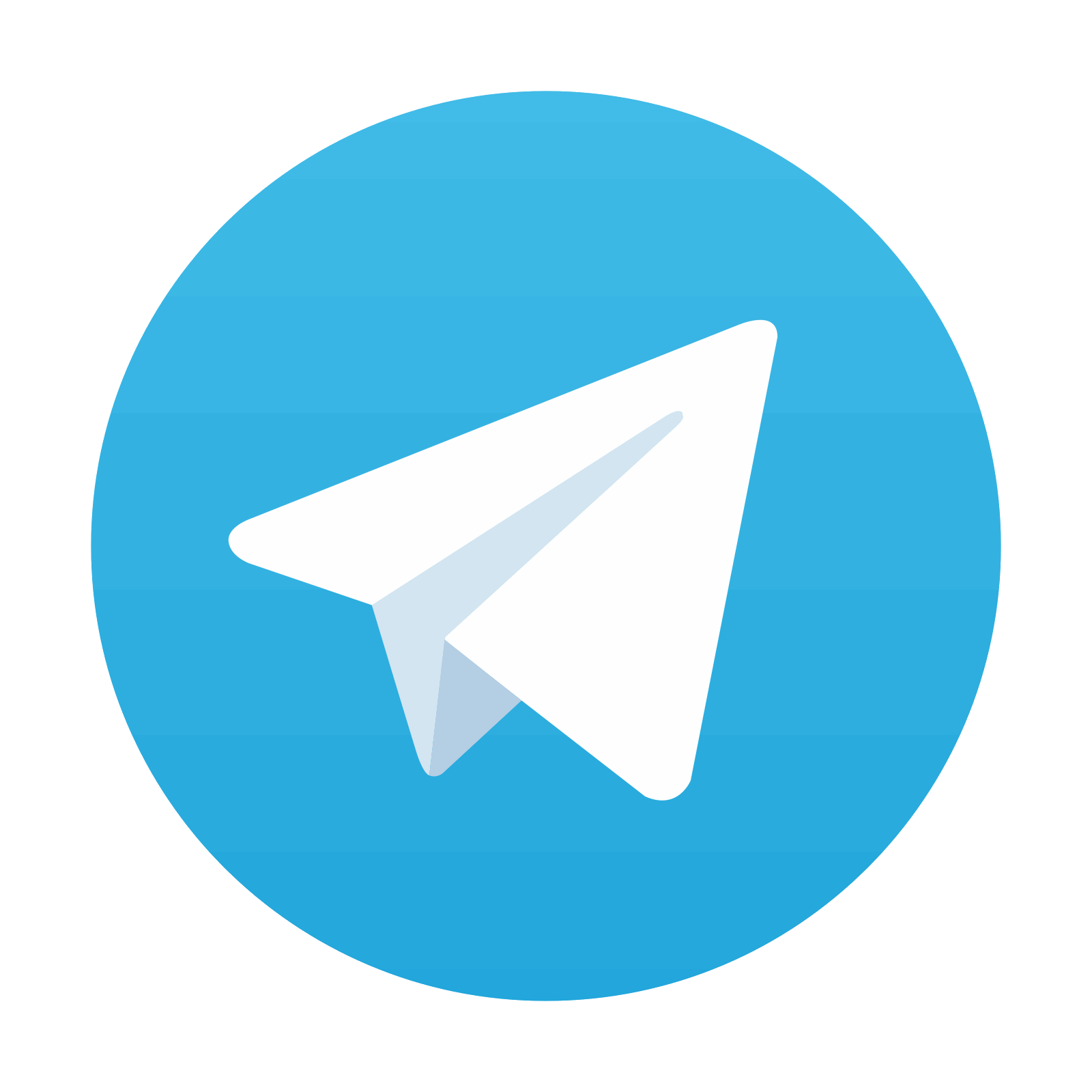
Stay updated, free articles. Join our Telegram channel

Full access? Get Clinical Tree
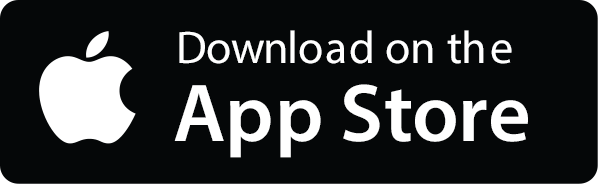
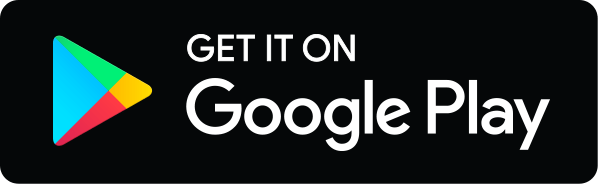
