Artifacts in Optical Coherence Tomography Angiography
Simon S. Gao, PhD; Yali Jia, PhD; and David Huang, MD, PhD
Optical coherence tomography angiography (OCTA) is a functional extension of optical coherence tomography (OCT) that uses blood flow-induced signal variation as the intrinsic contrast mechanism to differentiate vasculature from static tissues. It requires taking repeat cross-sectional B-frames at the same position and assessing the change in the OCT signal in each pixel of the B-frame over the multiple scans. Pixels corresponding to vasculature show fluctuations in the OCT signal as a result of flowing blood cells while pixels of static tissue show little change. OCTA algorithms differ in how they assess the change and what part of the complex OCT signal (amplitude, phase, or amplitude and phase) is assessed.1–3
OCTA typically involves capturing volumetric data, which can be visualized as horizontal or vertical (sagittal) cross-sections, as well as en face (coronal) sections using slabs defined relative to anatomic layers. En face presentation of volumetric OCTA data replicates the view of traditional dye-based angiography and greatly aids the clinician in interpretation (Figure 5-1). To produce en face angiograms, the first step is segmentation of relevant anatomic landmarks such as the inner limiting membrane (ILM), outer boundary of the outer plexiform layer (OPL), and Bruch’s membrane (BM). Segmentation can be performed in a manual, semi-automated, or automated manner.4–7 Angiograms can then be generated by taking the maximum flow value within the depth range set by some upper and lower boundary. A few common angiograms are as follows:
- Vitreous: normally avascular (above the ILM)
- Inner retina: the combination of superficial and deep retinal plexi (ILM to outer boundary of the OPL)
- Outer retina: normally avascular (outer boundary of the OPL to the BM)
- Choriocapillaris: normally near confluent (10 to 20 μm below the BM)
- Deeper choroid: larger choroidal vessels (more than 20 μm below the BM)
While OCTA can produce angiograms that provide a noninvasive way of visualizing retinal and choroidal vasculatures, OCTA images suffer from certain image artifacts. Artifacts can arise at different points during the generation of the OCTA image, including data collection, processing, and visualization. These artifacts ultimately result in the false presentation of flow data—either the absence of flow where there should be flow, or the presence of flow where there should be none. This chapter will describe and show examples of the types of artifacts that can be expected and need to be accounted for. The examples in this chapter were obtained using the AngioVue OCTA system (Optovue Inc), but the principles involved are generally applicable to all OCTA systems.
Figure 5-1. OCT angiograms of a normal macula. (A) The volumetric data comprise multiple cross-sections. The flow signal in each frame of this example is computed using the split-spectrum amplitude-decorrelation angiography (SSADA) algorithm. The data spans 3 mm in the x (horizontal) and y (vertical) dimensions and 2 mm in the z (axial depth) dimension. Composite cross-sectional angiogram shows that flow in the inner retinal vessels (purple) are projected onto bright photoreceptor and retinal pigment epithelium (RPE) layers (indicated by white arrows). Image processing software separates the vitreous, inner retinal layer, outer retinal layer, and choroidal layers along the inner limiting membrane (ILM), outer boundary of the outer plexiform layer (OPL), and Bruch’s membrane (BM) (dotted green lines). Five segmented flow volumes are separately projected. The projection algorithm finds the maximum flow value for each transverse position within the segmented depth range (slab), representing the fastest flowing vessel lumen in the segmented tissue layers. (B) The vitreous angiogram shows the absence of vascular flow. (C) The inner retinal angiogram shows normal retinal circulation with a small foveal avascular zone approximately 0.6 mm in diameter. (D) The outer retinal angiogram shows flow projection artifacts cast by flowing blood in the inner retinal vessels onto the RPE. (E) The outer retinal angiogram after removal of the projection artifact using a post-processing algorithm. (F) The choriocapillaris angiogram. (G) The deeper choroidal angiogram. Larger choroidal vessels are not visible because of projection from choriocapillaris. Scale bar shows the flow signal on an arbitrary scale.
PUPIL VIGNETTING ARTIFACT AND OTHER CAUSES OF NONUNIFORM SIGNAL STRENGTH
If the OCT system’s optical axis is not properly aligned with the center of the pupil, or if the pupil is too small, the OCT beam can be partially blocked by the edge of the iris during a portion of the volumetric scan. This results in dimmer reflectance on a portion of the en face structural OCT image and lower flow signal in the corresponding area in the en face OCTA (Figure 5-2). To address this problem, make sure the distance between the OCT system and the eye is adjusted so the fundus image (either infrared video or scanning laser ophthalmoscope) has the widest field of view. The transverse position of the OCT system should be adjusted so the OCT image has a uniformly high signal over the entire scan area. If these measures are insufficient, consider instilling (additional) mydriatic eye drops to enlarge the pupil. Opacity in the ocular media can also produce focal loss of OCT signal strength. If there is a small corneal scar or focal cataract, it may be possible to dilate the pupil and steer the OCT beam around the opacity. In general, there is no way to steer around a vitreous opacity. Post-processing algorithms to compensate for nonuniform OCT and OCTA signal strength can be helpful in such situations.
Figure 5-2. An example of pupil vignetting. (A) En face structural OCT showing reduced reflectance in the lower right corner of the image. (B) En face angiogram of the inner retina (6 × 6 mm) showing lower flow signal in the corresponding area.
Figure 5-3. En face inner retinal angiograms showing the effect of poor focusing. (A) Poor focusing results in an angiogram with weaker flow signal and vessels that appear blurred. (B) Proper alignment of the OCT system and participant produces a high-quality angiogram.
DEFOCUS AND OTHER PROBLEMS THAT REDUCE SIGNAL STRENGTH GLOBALLY
If the OCT beam is not properly focused, small vessels will appear blurred on en face OCTA and the flow signal will be reduced in general (Figure 5-3). The structural OCT image will also appear dimmer and have a lower signal strength index. Astigmatism, higher order aberrations, and dry eye can also lead to similar problems due to deterioration in focusing and reduced beam coupling. Polarization mismatch in the OCT system will reduce beam coupling and OCT signal strength globally as well. Optimizing the focus and polarization matching with the automated subroutines in the OCT system can address these problems. Using artificial tears to improve the corneal surface optical quality is another potential solution. It is important to make sure the signal strength index is within acceptable limits.
MOTION ARTIFACTS
Eye motion is detected by OCTA algorithms as flow and must be reduced to allow for clean visualization of true flow in blood vessels. Fortunately, bulk tissue motion produces a relatively uniform flow signal in the entire OCTA cross-section (Figure 5-4), and therefore can be reduced by subtraction.3,8,9 However, the bulk motion signal is not perfectly uniform. Fast motion could still leave a residual artifactual flow signal, particularly in bright structures such as the retinal pigment epithelium (RPE). These motion artifacts appear as bright lines on en face OCTA. There is typically 1 to 3 of these bright line artifacts per second corresponding to microsaccades. Larger microsaccades may cause vessels to appear disjointed. Blinking appears as dark line artifacts on en face OCTA.
There are 2 main strategies to reduce the effect of motion and correct for microsaccades. The first is to incorporate eye-tracking hardware and software with the OCT system. The eye-tracking system detects fixation changes and modifies the OCTA scan to account for deviations. In cases where the deviations are too great, such as with microsaccades, the particular B-frame can be discarded and reacquired.10 An alternative approach is to take multiple scans and register them. Because motion will be different in each scan, software registration of 2 or more scans can reduce the effect of or remove motion and microsaccades. Orthogonal registration of one horizontal and vertical raster scan has been demonstrated (Figure 5-5).11
Figure 5-4. Angiogram showing the effect of microsaccades. (A) En face inner retinal angiogram. Two bright lines in the lower part of the image is the result of a microsaccade, disrupting vessel continuity (green arrows). (B) Typical cross-sectional angiogram (from the green, dotted line in panel A) without motion artifact. (C) Cross-sectional angiogram at the position of the larger microsaccade shows high flow signal at all reflective structures.
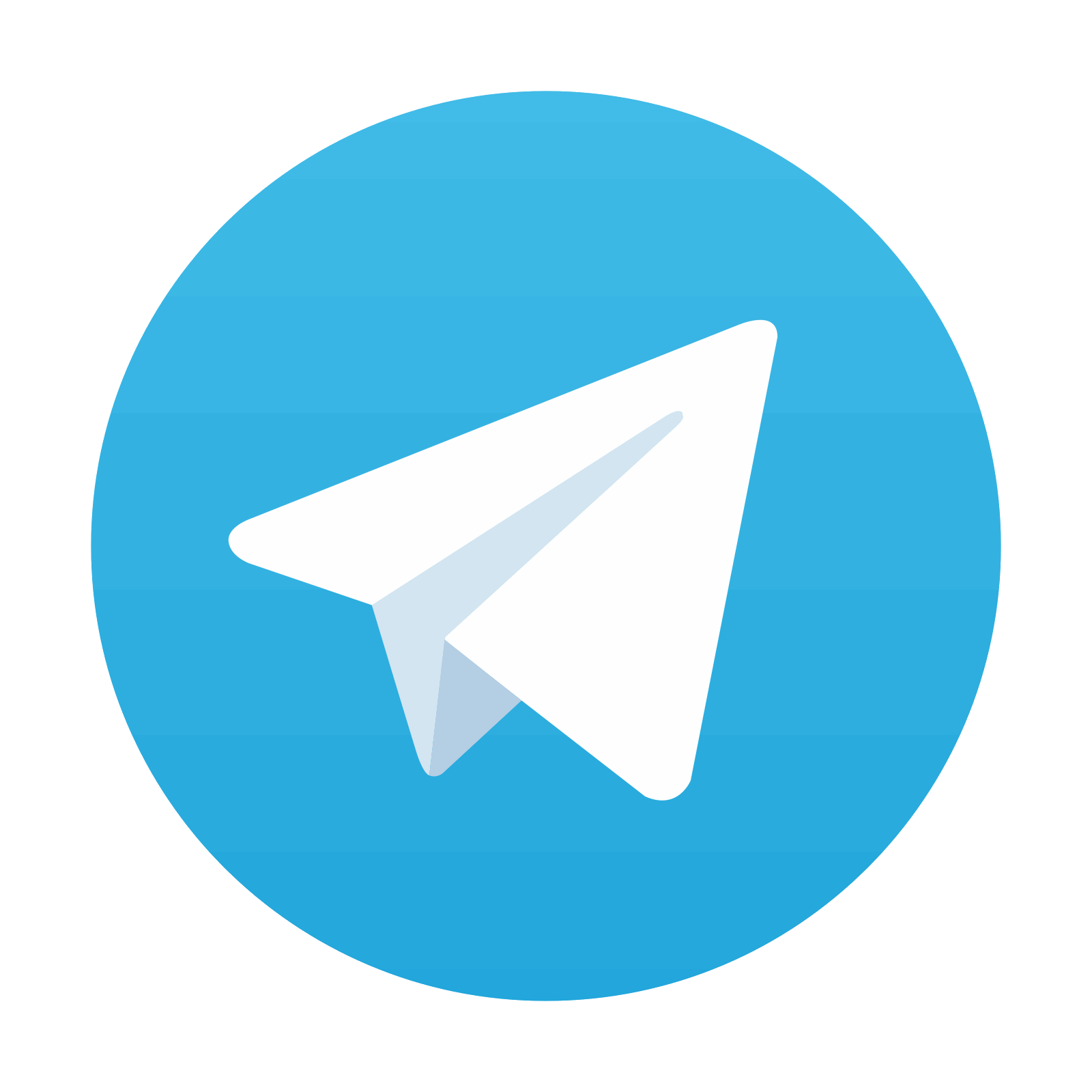
Stay updated, free articles. Join our Telegram channel

Full access? Get Clinical Tree
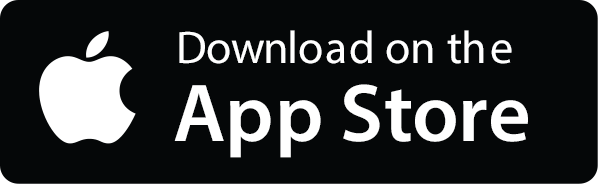
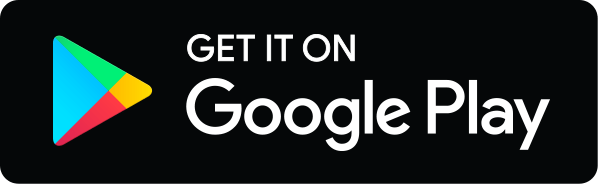