Syndrome
Airway-associated features
Difficult mask ventilation?
Difficult intubation?
Apert (acrocephalosyndactyly)
Midface hypoplasia, soft palate against posterior pharyngeal wall, narrow palate ± cleft
Due to upper airway obstruction
Potential difficult intubation
Cornelia de Lange
Microcephaly, high-arched palate
Potential difficult intubation
Crouzon (craniofacial dysostosis)
Maxillary hypoplasia, soft palate against posterior pharyngeal wall
Due to upper airway obstruction
Potential difficult intubation
Down (trisomy 21)
Small oral cavity in relation to normal tongue size, obstructive sleep apnea, possible atlanto-occipital instability
Due to upper airway obstruction
Potential difficult intubation due to small mouth opening. May require head neutral position during intubation
Goldenhar (oculoauriculovertebral dyplasia)
Micrognathia, facial hypoplasia, and limited cervical neck mobility
Very difficult intubation
Hallermann-Streiff (oculomandibulodyscephaly)
Mandibular and malar hypoplasia, small mouth, faulty dentition, anteriorly displaced temporomandibular joints
May be difficult to open mouth and perform jaw thrust
Potential difficult intubation with small mouth
Robin sequence (Pierre-Robin syndrome)
Micrognathia, glossoptosis, cleft palate, upper airway obstruction
Upper airway obstruction due to large tongue
Very difficult intubation
Treacher Collin (mandibulofacial dysostosis)
Malar and mandibular hypoplasia, cleft palate, incompetent soft palate
Due to hypoplastic mandible making mask seal and jaw thrust difficult
Very difficult intubation
Past Surgical and Anesthetic History
Prior surgeries should be reviewed paying particular attention to those patients with syndromes requiring serial procedures. Though many patients will carry a diagnosis of difficult airway through their entire lives, airway conditions for some may change over time. For example, patients who have had mandibular distractors placed and who have had significant interval growth may have improved airway anatomy now amenable to routine direct laryngoscopy intubation technique. While the most recent anesthetic record may be the most relevant for current planning, older records may reveal sequential changes and/or best practice. All available anesthetic records should be scrutinized for documentation of untoward reactions, challenging vascular access, difficult mask ventilation or tracheal intubation, and variation from expected tracheal tube size. Techniques that have been successful should be included in the development of the anesthetic plan. Evidence of postoperative complications such as prolonged emergence, inadequate pain control, nausea and vomiting, extended supplemental oxygen requirement, and unanticipated hospital admission should be sought. When asked, parents of children with syndromes and/or chronic illness requiring repeated anesthetics will often identify techniques that have resulted in improved (or worse) recovery.
Medications
A list of medications and the conditions for which they are prescribed is essential. Most prescribed medications should be administered on the morning of surgery and may be taken with a small sip of water. Longstanding antihypertensive regimens should be continued. Children with asthma should be treated the night before and morning of surgery with inhaled bronchodilators and with steroids as advised by their pediatrician or pulmonologist for those with moderate to severe disease. Over-the-counter and herbal medicine and, in particular, aspirin should be stopped at least 1 week prior to surgery, unless there is a specific reason to continue them [4]. Other nonsteroidal anti-inflammatory drugs, such as ibuprofen, may be allowed up to 3 days prior to surgery.
Allergies
A history of allergies to medications and other items found in the operating room such as latex, betadine, or chlorhexidine should be noted. Specific adverse reactions to medications should be listed because some, such as diarrhea with a particular antibiotic, do not constitute true allergy. Only 10–15% of patients who report penicillin allergy are found to have actual IgE-mediated allergy upon testing [7, 8]. In patients with proven penicillin allergy, 2.5% will develop an allergy to the cephalosporins commonly used for surgical site infection prophylaxis, while in patients simply reporting penicillin allergy, only 1% are cross-reactive. The incidence of cross-reactivity is substantially lower with third- and fourth-generation cephalosporins [9, 10]. Vancomycin , administered to patients positive for methicillin-resistant Staphylococcus aureus (MRSA) , may cause flushing and pruritis “red man syndrome” when infused too rapidly; this does not represent a true allergy and is generally prevented by administration times of an hour or more [11]. Children with myelomeningocele, bladder exstrophy, or cerebral palsy with ventriculoperitoneal shunts may be at increased risk of latex allergy [12–14], which may lead to life-threatening anaphylactic reactions. However, latex-allergic children can be safely anesthetized if exposure to latex in the medical environment is avoided and prophylactic medications appear to be unnecessary [15]. With the increased use of non-latex products overall, anaphylactic reactions to latex in the operating room have declined significantly in the past two decades and are now rare events.
Review of Systems
Important areas include general health, development, and exercise tolerance. Prematurity and the presence of associated reactive airway disease and/or bronchopulmonary dysplasia may predispose to perioperative respiratory complications. Recent upper respiratory infection (URI) , bronchiolitis, or previous episodes of croup are of particular concern. URI with active symptoms or with symptoms less than 2 weeks prior to surgery is associated with a twofold increase of perioperative respiratory adverse events, such as bronchospasm, laryngospasm, and airway obstruction [16]. In patients with congenital cardiac disease, understanding the nature of the lesion, its correction or palliation, and the current functional status is essential. Consultation with the patient’s cardiologist and repeat echocardiography may be prudent if there have been no such recent evaluations, or the patient’s cardiopulmonary symptoms or exercise tolerance has changed, or the planned procedure is expected to place unusual demands on the heart. For those infants and children with residual complex cardiac disease, such as those with hypoplastic left heart syndrome, management by anesthesiologists with subspecialty training in pediatric cardiac anesthesia is prefered. Renal and hepatic diseases affect drug disposition and metabolism. Anesthetic management is also affected by neurologic disease and autism spectrum disorders, including convulsive disorders, cognitive impairment, and neuromuscular disease.
Family History
A family history suggestive of possible malignant hyperthermia (MH) , pseudocholinesterase deficiency (succinylcholine sensitivity), neuromuscular disease, or coagulation disorder deserves detailed exploration [17]. MH has an incidence of 1:5000 to 1:50,000–100,000 with a higher prevalence in males [17, 18]. The incidence of pseudocholinesterase deficiency is 1:3200–5000 [19]. While several laboratory tests may be useful in determining risk for these disorders, comprehensive genetic testing remains under development.
Physical Examination
Age, Weight, Height, and Vital Signs
Patient age affects the approach to induction, need for preanesthetic medication, and anesthetic risk. Children less than 1 year of age have an increased risk of complications, especially children in the first 2 months of life [20]. If possible, procedures that are entirely elective should be postponed until after 6 months of age. The weight of the child affects the dose of medications administered and reflects the child’s general state of health. Significant medical problems often result in growth failure. A determination of BMI and ideal body weight are essential for dosing in overweight/obese patients. Heart rate, blood pressure, and temperature should be determined prior to induction of anesthesia. Pulse oximetry (SpO2) is measured prior to transfer to the operating room in order to rule out unanticipated lower respiratory tract system or cardiac pathology.
Physical Examination
The examination of the airway focuses on mask fit, mouth opening, neck mobility, tongue size, thyromental distance, and mandibular size. Patients who are less than a year old, weigh less than 10 kg, have short thyromental distance, and have preexisting airway or craniofacial abnormalities are at risk for difficult intubation [21–24]. Examination of mouth opening in the uncooperative infant may be facilitated by removing and inserting a pacifier and observing the extent of opening. The modified Mallampati grading system for mouth opening divides patients into four groups: Class 1: the soft palate, uvula, fauces, and pillars can all be visualized; Class 2: the soft palate, uvula, and fauces are visible; Class 3: the soft palate and base of uvula are visible; and Class 4: only the hard palate is visible [25, 26]. If the Mallampati score can be obtained, higher incidences of difficult laryngoscopy are seen in patients with Mallampati scores 3 and 4 [23].
Protruding or loose teeth (especially in children aged 5–9 years) should be noted to assess whether in the former case they may interfere with laryngoscopy or whether in the latter case they should be removed to minimize pulmonary aspiration risk. Adequate neck mobility is important in the alignment of the oropharyngeal and tracheal axis for the best laryngoscopic view.
The chest examination should include observations on the symmetry of chest excursion, ease of respiratory effort, and auscultatory findings. Elective procedures should be postponed if rhonchi are heard, indicating active bronchitis or pneumonia. Mild to moderate wheezing may be treated with a bronchodilator in advance of induction to see if it improves and to prepare the airway for the stimulus of intubation. The cardiac examination should note the color of the extremities and the quality of peripheral pulses. Heart murmurs are most commonly innocent functional murmurs, but can be indicative of serious undiagnosed pathology. Functional murmurs are soft systolic murmurs that are best heard along the left sternal border, change with respiratory cycle and patient position, and have no diastolic component or clicks. If the child’s pediatrician has noted the murmur on previous examinations and believes it to be innocent, no additional workup is generally needed. Cardiology is consulted if the patient is symptomatic or if there is any doubt about murmur type.
Preoperative Testing
Routine preoperative laboratory testing for healthy patients is unnecessary. Determination of the patient’s hemoglobin level is needed only if anemia is suspected or for operations where substantial blood loss is anticipated, such as craniofacial reconstruction. Similarly, in asymptomatic patients, coagulation studies are reserved only for procedures associated with large blood loss. All menstruating girls are screened for pregnancy with an urine or serum ß-HCG level.
Preoperative Preparation
A child’s fear of strangers, needles, pain, foreign environments, and separation from parents must be addressed [27]. Having informed, calm parents is the best way to ensure that the child will be calm and relaxed on the day of surgery. A visit to the hospital prior to the planned surgery allows the child and parents to have their questions answered in a relaxed setting and provides the information necessary for the parents to prepare their child for the day of surgery. This visit has shown to reduce parental anxiety on the day of surgery [28]. Telephone interviews, brochures, and web resources are alternatives when a preoperative hospital visit is not possible.
In an age-appropriate manner, children should be informed about what to expect on the day of surgery, using an honest, positive, and non-threatening approach. They should know that they will be in a deep sleep brought on by medicines and will not awaken until after the operation is over. They should be informed of the plan for separation from their parents, induction of anesthesia, and postsurgical process, including staying in the recovery room and management of postoperative pain.
Risks of Anesthesia
Although anesthesia is generally considered very safe for healthy children, the preoperative meeting with the family should still include a discussion on the potential risks and complications of anesthesia. Some common adverse reactions to anesthesia include postoperative nausea and vomiting (6%) and sore throat. Less common complications include respiratory complications such as airway obstruction (1.1%), laryngospasm (1.7%), bronchospasm (0.2%), aspiration (0.04%), and allergic reactions (0.01–0.1%) [29–32]. Severe complications, such as cardiac arrests that are attributed to anesthesia, are rare (0.65–1.4:10,000 procedures) [33, 34]. The incidence of both respiratory complications and cardiac arrest are much higher in children under 1 year old and especially children under 1 month old.
Neurotoxicity
Recent investigations have raised concern about the potential harm of anesthetic exposure to a developing child’s brain. Animal studies, including several in nonhuman primates, demonstrate that many general anesthetic agents used in human clinical practice can be neurotoxic to the developing brain. The issue was brought to scientific attention in 1999 when Ikonomidou et al. showed that blockade of N-methyl-D-aspartate (NMDA) glutamate receptors for only a few hours during late fetal or early neonatal life triggered widespread apoptotic neurodegeneration in the developing rat brain [35]. Early exposure to ethanol, which blocks NMDA glutamate receptors and activates gamma-aminobutyric acid (GABAA) receptors , results in similar pathology [36]. Neonatal rats exposed to a combination of midazolam (GABA-agonist), isoflurane (combined GABA-agonist and NMDA-antagonist), and nitrous oxide (NMDA-antagonist) maintained at a surgical depth of anesthesia experienced both immediate brain cell loss and impaired long-term neurocognitive function [37]. A variety of neurotoxic effects, including acute neuronal cell loss, altered dendritic architecture, reduced synaptic density, decreased levels of neurotrophic factors, mitochondrial degeneration, destabilization of the cytoskeleton, and cell cycle abnormalities, have been described following exposure to standard anesthetics and sedatives in a broad range of immature animal species [38].
Whether these findings translate to human risk remains unclear. There is no accepted unified mechanistic theory to explain the neurotoxic effects, and the pathologies themselves vary across animal species. Many of the animal studies utilize anesthetic agents in doses and durations that far exceed clinical equivalents for humans [39]. Furthermore, anesthetic management is less controlled in experimental neonatal animal models than in humans; the neonatal animal’s small size precludes continuous hemodynamic monitoring, assessment of acid-base status, and glucose levels, all of which normally dictate changes in anesthetic management of a human neonate [38]. Unrecognized hypotension, hypercarbia, metabolic acidosis, and hypoglycemia associated with anesthesia in a neonatal rodent could lead to pathology and neurologic deficits without direct biochemical neurotoxicity [38–42].
Several large retrospective human cohort studies evaluating behavioral and cognitive effects of anesthetic exposure in the developing brain have been completed. Researchers from the Mayo Clinic compared standardized aptitude test score in 5357 students, out of which 593 had general anesthesia before age 4 with their unexposed peers. In a follow-up study, 350 children who were exposed to anesthesia before age 2 were matched to 700 unexposed controls. Both studies showed there were no differences in test scores between children who were unexposed and children who had had a single anesthetic exposure. However, in both studies, children who had multiple anesthetic exposures did incrementally worse than their peers who received none [43, 44]. A study consisting of 2689 Danish children who had surgery in infancy were age-matched to a control group of 14,575 children who did not. Academic tests scores at ninth grade showed no differences between these groups [45]. Using the Western Australian Pregnancy Cohort Study data, consisting of 2608 children with 321 of them exposed to anesthesia before 3 years old, Ing et al. found some associations between anesthetic exposure and language, cognitive function, motor skills, and behavior outcomes at age 10 y [46]. However, the results were neither consistent within nor across modalities: one language test, the Clinical Evaluation of Language Fundamentals, showed clear differences in receptive language ability, whereas no differences were seen in the Peabody Picture Vocabulary assessment and no differences in visual tracking and attention (Symbol Digit Modality Test), fine and gross motor function (McCarron Assessment of Neuromuscular Development), or behavior (Child Behavior Checklist) related to exposure status.
Although the results of these retrospective cohort studies have not been conclusive, in aggregate they suggest that a brief, single anesthetic exposure is not associated with future learning disability and/or lower test scores. For outcome differences that are suggested in these nonrandomized studies, the child’s underlying pathology or surgery itself may confound effects otherwise attributable to anesthesia [38].
Researchers conducting the large multinational prospective trial, General Anesthesia versus Spinal (GAS Study), sought to more definitively answer the neurotoxicity question. The study design minimized the confounding effects of surgery by comparing only infants who had a single surgery – inguinal hernia repair. Subjects were randomized to general or regional anesthesia, the latter generally acknowledged being non-neurotoxic. Cognitive development at 2 y of age using the Bayley Scales of Infant Development III test were no different between the two groups when <1 hr of sevoflurane anesthesia was administered [47]. A 5-year follow-up intelligence test is planned.
Although these results were reassuring, there are still many important concerns and questions that cannot be answered by the GAS study. Brain function is very complex and cannot be fully measured with a single cognitive test. How does exposure to anesthetics affect the development of other functions such as memory, language, motor skills, and emotional development? Do these effects change when multiple anesthetic drugs are combined? Are the results the same for procedures other than hernia repair? Animal and epidemiological data suggest that the risk of anesthetics to the developing brain increases with longer duration exposure or multiple exposures. Is this true for children? Further research will be required to address these questions [48].
At present, however, healthcare providers may best be advised to follow and share the consensus statement released by SmartTots.org, a partnership between the US Food and Drug Administration (FDA) and the International Anesthesia Research Society (IARS), which states the following [48, 49]:
…It is not yet possible to know whether anesthetic drugs are safe for children in a single short duration procedure…Concerns regarding the unknown risk of anesthetic exposure to the child’s brain development must be weighed against the potential harm associated with cancelling or delaying a needed procedure. Each child must be evaluated individually based on age, the type of procedure, level of urgency, and other health factors…
Preoperative Fasting
The purpose of the fast is to decrease the risk of aspiration of gastric contents after the patient undergoes anesthesia. The volume of gastric contents depends on continuous gastric secretion, swallowed saliva, ingested solids and liquids, and rate of gastric emptying. Gastric contents empty at a variable rate depending on the volume and constituents of the content. The most recent fasting recommendations are outlined in Table 5.2 .
Table 5.2
Preoperative fasting recommendations for healthy patients undergoing elective procedures
Ingested material | Minimum fast (hr) |
---|---|
Clear liquids | 2 |
Breast milk | 4 |
Infant formula | 6 |
Nonhuman milk | 6 |
Meal (toast and clears) | 6 |
Other meal | >8 |
Clear Liquids
Clear liquids, including water, oral electrolyte solution (Pedialyte®), juices without pulp, carbohydrate drinks, and coffee or tea without milk, move rapidly out of the stomach; the 50% emptying time is 12 min [50]. Two hours after a clear liquid drink, most individuals’ gastric volume will be similar to someone who had completed an overnight fast [51–54]. Therefore, most pediatric anesthesiologists allow healthy children to drink clear liquids up to 2 hr prior to induction, which appear to be safe and has other benefits [55]. Children are less irritable, less thirsty and hungry, and tolerate the preoperative wait better if they are allowed to drink. They are also less likely to be hypoglycemic.
Milk and Formula
A minimum of a 4-hr fast is advisable after breast milk and 6 hr after nonhuman milk or infant formula [56–59]. Healthy infants <6 mo of age may be eligible for formula fasts relaxed to 4 hr [60]. Scheduling surgery early in the day helps minimize the waiting period and avoids some of the feeding problems for young infants.
Solids
Solid meals have linear gastric emptying patterns with the mean half-time of emptying of approximately 5 hr for a filling meal [55]. Based on current evidence, it is recommended to fast for 6 hr after a light meal and 8 hr after a full meal [61]. Chewing gum is considered equivalent to drinking clear liquids, as it generates saliva and stimulates gastric secretion. However, swallowing chewing gum would be considered as solid intake and delay the procedure for 6 hr [62].
Patient compliance is a potential problem for children, regardless of preoperative instructions. Explicit written instruction for patients and their families helps avoid feeding policy violations, and these instructions should be reinforced during preoperative visits or phone calls. On the day of surgery, the anesthesiologist should also verify the patient’s PO intake status.
Reduction of Anxiety During Induction
Up to 60% of young children undergoing anesthesia and surgery experience significant anxiety [63, 64]. Some of the risk factors associated with the development of preoperative anxiety include age (highest with children between 1–5 years), temperament and baseline anxiety, past medical encounters, and parental anxiety [65]. Anxiety is not only psychologically stressful for the patient and family, it has also been linked to significantly higher postoperative pain, delayed hospital discharge, and higher incidence of emergence delirium, sleep disturbances, and other maladaptive behavioral changes that last up to a few weeks following surgery [66, 67]. Given the negative consequences of preoperative anxiety, several approaches have been used to reduce anxiety in the child and to some extent the parent. Some common methods include parental presence during induction, distraction techniques using toys or electronic devices, eliciting the help of child-life specialists, and sedative premedications.
Parental Presence
The idea that a parent could lessen the anxiety of anesthetic induction is not new, and although parental presence at induction (PPIA) is used at many institutions, often at the request of the parent, the evidence of its effectiveness is mixed. Earlier studies indicate that children will be calmer with a parent present, while some other studies showed no effect to the child, but a reduction in anxiety of the parents [63, 68, 69]. A recent Cochrane review showed that parental presence made no difference in the child’s anxiety and was far less effective than premedication [70]. Of note, children of anxious parents do not benefit by having their parents present during induction. The ultimate choice of whether or not to include the parent rests with the anesthesiologist and the institution. If a PPIA is chosen, it is important to mentally prepare the parents who are about to witness the physiological changes of their child during the induction of anesthesia.
Preoperative Sedation
Compared to PPIA, sedative premedication may lower children’s preoperative anxiety and improve compliance with face mask on induction [71]. At present the most commonly used medication is oral midazolam (0.5 mg/kg to 10–15 mg maximum doses). Midazolam is a short-acting benzodiazepine, where an oral dose of 0.5–0.75 mg/kg permits satisfactory separation in 15–30 min and results in 45–60 min of sedation [72]. Oral midazolam is generally well tolerated although some children may refuse it and rare adverse reactions such as disinhibition and dysphoria have been recorded [73]. Midazolam may also be administered intranasally at a dose of 0.3 mg/kg.
Although not commonly used, ketamine is an effective preanesthetic medication at an oral dose of 6–8 mg/kg [74, 75]. Sedation begins in approximately 20–25 min after administration, and almost all patients are calm at the time of induction. Oral ketamine (3 mg/kg) can also be added to oral midazolam (0.5 mg/kg) to supplement inadequate sedation or to heavily sedate a difficult patient. For patients with severe behavioral problems, such as autism, 4–5 mg/kg intramuscular ketamine can be extremely effective. Regardless of its route of administration, ketamine stimulates the secretion of saliva and should be given in conjunction with an antisialagogue, such as glycopyrrolate or atropine. Although ketamine has been associated with hallucinations in adults, the incidence was not significant in the pediatric population and may be countered by concomitant midazolam administration [76].
Operative Management
General Anesthetic Induction Techniques
Inhalation and intravenous induction are the most common techniques for the induction of general anesthesia. An inhalational induction is often chosen for fasted healthy children without preexisting intravenous lines. However, if a child requires an emergency procedure under general anesthesia and is not appropriately fasted, a rapid sequence intravenous induction is usually indicated. Children with complicated medical conditions such as a known or suspected difficult airway, cardiac disease, and severe gastroesophageal reflux require special induction strategies.
Inhalation Anesthetics
Inhalation induction of anesthesia may be facilitated by either preoperative sedative administration or parental presence. Distraction techniques such as singing songs, playing a movie or game on a tablet, and using flavors to disguise the smell of the anesthetic all help to smooth the induction process. Of the many inhalation anesthetics, only nitrous oxide, sevoflurane, isoflurane, and desflurane remain commonly used in the United States. Nitrous oxide is not a potent agent and is typically used to supplement the potent inhalation agents or intravenous anesthetics. The minimum alveolar concentration (MAC) is the alveolar concentration at which 50% of patients do not move to skin incision and is the standard measure for potency of an anesthetic gas [77]. MAC increases with age, reaching a maximum value in infants 1–6 months of age, and gradually decreases through adulthood [78–80] (Table 5.3). Despite having a higher MAC than older children, neonates and infants are more susceptible to myocardial depression from the inhalation agents and are more prone to cardiac arrest secondary to overdose [29, 34, 81, 82].
Table 5.3
Inhalation anesthetics : effect of age on potency
Minimum alveolar concentration (MAC) | ||||||
---|---|---|---|---|---|---|
Agent | λB/G | Neonate | 1–6 mo | 1–3 y | 3–10 y | Adult |
Nitrous oxide | 0.46 | … | … | … | … | 104 |
Isoflurane | 1.3 | 1.6 | 1.8 | 1.6 | 1.6 | 1.2 |
Desflurane | 0.42 | 9.2 | 9.9 | 8.6 | 8.0 | 7.0 |
Sevoflurane | 0.66 | 3.3 | 3.2 | 2.5 | 2.5 | 2.1 |
Sevoflurane has become the anesthetic of choice for inhalation induction, as it is the least irritating agent to the airway and provides excellent cardiovascular stability [83, 84]. These qualities plus its insolubility make anesthetic induction very rapid and also readily allow tracheal intubation without the use of muscle relaxants [85, 86]. For most healthy children without cardiovascular disease, an inhalational induction can be safely performed with sevoflurane up to 8%, mixed with nitrous oxide 70% and oxygen 30%. Desflurane is the newest inhalational agent, introduced 2 years after sevoflurane in 1992. Although it is associated with a rapid emergence and recovery due to its low blood solubility [87], it is highly irritating to the upper airway and is not recommended as an induction agent [88]. Furthermore, unlike other inhalation agents that have bronchodilation effects, desflurane can cause bronchoconstriction at levels above 1 MAC [89]. Isoflurane was introduced in the 1980s and is characterized by its higher blood solubility [90]. It is also irritating to the airway and is therefore not recommended for inhalation induction, but can be used for maintenance of anesthesia. It is significantly less expensive than either sevoflurane or desflurane.
Intravenous Anesthetics
Intravenous induction may be chosen as a technique to manage a known or suspected difficult airway, or if there is concern about aspiration risk, or because an intravenous line is already in place. Compared to an inhalation induction, it provides a faster induction but is also more likely to result in patient apnea [91]. For patients who do not have an established intravenous catheter, several techniques may facilitate catheter placement. Cutaneous analgesia can be achieved with the topical application of an eutectic mixture of local anesthetics (EMLA 5% cream, Astra Pharmaceuticals) or lidocaine 4% ointment (ELA-Max) 30–60 min prior to placing the catheter [92]. Unfortunately, EMLA may cause significant cutaneous vasoconstriction, making cannulation more difficult [93]. Application of 50% nitrous oxide in oxygen by face mask provides effective analgesia within 2–3 min and is commonly practiced. Prior to venous cannulation, local infiltration of 1% lidocaine with a small (30 gauge) needle may further decrease discomfort. This approach has been shown to be superior to EMLA by one set of investigators [94].
Propofol, ketamine, and etomidate are viable choices for intravenous induction of anesthesia. Propofol dominates current practice due to its rapid induction of anesthesia, fast recovery profile, and low incidence of nausea and vomiting. The usual induction dose for children ranges between 2.5 and 3.0 mg/kg [95], but infants may require higher doses [96]. Intravenous injection of propofol may cause a localized burning sensation. Injecting 2% lidocaine (1 mg/kg) immediately prior to propofol administration can help minimize this. Ketamine, an NMDA receptor antagonist, is not commonly used as an induction agent in oculoplastic patients due to drug-induced nystagmus and, at high doses, increased intraocular pressure [97, 98]. Its use is typically reserved for patients who are unable to cooperate or sedate by other means (intramuscular ketamine as described above), or in patients with a difficult airway where preserving spontaneous respiration is important. The usual induction dose is 2–4 mg/kg IV [74, 75, 99]. Etomidate is a GABA receptor agonist and is typically reserved for patients who are hemodynamically unstable or in patients with congenital heart disease where it is important to maintain systemic blood pressure.
Propofol infusions (50–100 mcg/kg/min) can be used for sedation during procedures performed under local or regional anesthesia. The level of sedation is readily adjusted to the patient’s needs, and it dissipates rapidly after short-term infusions are discontinued. Higher propofol infusion rates (100–300 mcg/kg/min) may be used in place of inhalation agents for general anesthesia. When combined with ultrashort-acting intravenous agents such as remifentanil, total intravenous anesthesia (TIVA) can replace the need for inhalational agents. TIVA is particularly useful when inhalational agents are to be avoided, such as in patients who are at risk or have a history of malignant hyperthermia. A recent comparative study in pediatric strabismus surgery, however, showed no significant differences in incidences of postoperative hypoxemia, vomiting, and time to PACU and hospital discharge between intravenous and inhalation techniques [100]. A study in adults, however, showed that the TIVA technique compared to inhalational agents was associated with a lower incidence of postoperative nausea and vomiting [101].
Although not an induction agent, dexmedetomidine is a valuable adjunct agent used to supplement the effects of other anesthetic agents. It is an alpha-2 adrenergic agonist and causes sedation, anxiolysis, and analgesia, without the respiratory depression associated with propofol. Dexmedetomidine can be used alone to provide sedation or with other agents to decrease the total anesthetic requirement [102]. One of the drug’s common side effects is bradycardia [103].
Muscle Relaxants
Muscle relaxants may be used to facilitate tracheal intubation and/or to maintain paralysis during surgery. The specific choice of neuromuscular blocking agent is based on the duration of surgery and the patient’s needs (Table 5.4). The volume of distribution and the plasma concentration of these agents are related to the patient’s age and other comorbidities, which the anesthesiologist must be attuned to. Several reviews detail the pharmacology of these agents [104, 105].
Table 5.4
Properties of common neuromuscular relaxants
Drug | Intubation dose (mg/kg) | Onset time (min) | Recovery time to 25% twitch height (min) |
---|---|---|---|
Depolarizing | |||
Succinylcholine | 1–2 IV 4 IM | 1 | 4 10–20 |
Non-depolarizing | |||
Rocuronium | 0.6 IV 0.9 IV 1.2 IV 1.8 IM | 1–1.5 1–1.25 1 2.5–3.7 | 27–37 53 73 89 |
Atracurium | 0.5 IV | 1.5 | 33 |
Cisatracurium | 0.1 IV | 1.7 | 31 |
Vecuronium | 0.1 IV | 1.3 | 24 |
Pancuronium | 0.1 IV | 2.5 | 60–90 |
Succinylcholine is the only depolarizing relaxant regularly used today. It has the fastest onset of any of the muscle relaxants but is associated with numerous side effects, some of which are of particular interest to the surgeon. It increases intraocular pressure and should be used with caution depending on the nature of the proposed procedure (open eye wound or eye pressure measurement). After a single dose of succinylcholine, increased intraocular pressure peaks at 25 mmHg after 90 s and returns to baseline (15 mmHg) in 5 min [106]. Anecdotes of extrusion of ocular contents from succinylcholine have been reported, but recent reviews on this issue do not substantiate this risk [107]. Of greater concern, succinylcholine can trigger malignant hyperthermia in susceptible individuals and life-threatening hyperkalemia in patients with myopathies, recent burns, or major trauma. Having earned a black box warning from the Food and Drug Administration in 1993, succinylcholine is currently indicated for rapid sequence induction and treatment of laryngospasm, and it remains the only drug recommended for intramuscular administration in emergency circumstances.
Non-depolarizing agents do not increase intraocular pressure. Rocuronium has a rapid onset of action and may be used as part of a rapid sequence induction for emergency surgery. Should early reversal of its associated neuromuscular blockade be required, a new agent, sugammadex, may be used. Vecuronium and cisatracurium are intermediate in onset and duration and are appropriate choices for many ophthalmologic procedures. Pancuronium is an older, inexpensive drug and remains an appropriate choice for surgical procedures that exceed 45–60 min. In general, the effects of non-depolarizing muscle relaxants may be reversed with neostigmine after the neuromuscular junction begin to recover. This reversal is preceded by a dose of an anticholinergic drug such as atropine or glycopyrrolate to prevent bradycardia associated with neostigmine administration. Because many of the non-depolarizing agents require 20 min or longer to reach the point of being reversible, the anesthesiologist should be kept informed of the estimated surgical duration to assure administration of the appropriate type and dose of non-depolarizing agent.
Intraoperative Monitoring and Operating Room Environment
Basic monitoring for pediatric patients consists of pulse oximetry, electrocardiography, automated noninvasive blood pressure cuff, capnography, temperature monitoring, and agent-specific gas analysis. For brief procedures, such as nasolacrimal duct probing, an intravenous catheter may not be essential, but it may be beneficial for administration of intravenous fluids and medications to reduce the risk of nausea and vomiting, and management of pain and postoperative agitation.
Infants and children can rapidly cool in the operating room environment. The room temperature during induction and emergence should be appropriately warm; young infants should be kept warm with an underbody and overhead warmer. A warm blanket helps diminish heat loss during the procedure, as does a heat moisture exchanger in the anesthesia circuit [108]. For longer cases, the addition of a forced warm air blanket that covers or surrounds the child helps minimize the risks of either hypothermia or hyperthermia.
Airway Management
General anesthesia can be maintained either via face mask, laryngeal mask airway (LMA), or tracheal tube. The type of procedure, experience of the anesthesiologist, and age and comorbidities of the patient will determine the choice of appropriate airway.
Face Mask
Pediatric face masks come in many different sizes to fit patients of all ages. It should readily conform to the face and allow the anesthesiologist to visualize the mouth and nose. In patients who have upper airway obstruction after induction of anesthesia, an oral or nasal airway can help relieve the obstruction. Short procedures such as probing and irrigation of blocked nasolacrimal ducts can be done with a face mask, but LMAs are often preferred for better surgical access and control of secretions.
Laryngeal Mask Airway
Developed in the 1980s, the LMA has become an essential device for both routine and difficult airway management. Several designs and models are available (Fig. 5.1). The LMA provides a direct route for oxygen and anesthetic gases to reach the trachea without the need for visualization of the larynx or insertion of a tube into the trachea. The tip of the LMA tip sits in the esophageal inlet; the cuff extends to the base of the tongue and forms a low-pressure seal around the larynx. A fenestrated opening surrounds the larynx with a semirigid tube connecting to a circuit or ventilator outside the mouth. The LMA is included in the American Society of Anesthesiologists difficult airway algorithm and can be lifesaving in the “cannot ventilate, cannot intubate” scenario [109]. Seven sizes based on the weight of the patient are available ranging from newborn to large adult. Although investigators have reported excellent success with the smallest LMA size [110], many are more reluctant to use the #1 LMA for maintenance of anesthesia in infants and reserve it for brief procedures or as an emergency airway device. The occasional failure of the #1 LMA is thought to be due to the neonate’s relatively larger and more flaccid epiglottis blocking the LMA inlet [111].


Fig. 5.1
Comparisons of different laryngeal mask airways (LMA) . From top clockwise, trademarked brands are in () low-profile flexible LMA (Ambu), LMA with gastric suction port (LMA Supreme), intubating LMA (Cookgas Air-Q), regular LMA (Ambu)
The LMA should be thought of as a mask and not a tracheal tube. Laryngospasm and aspirations of regurgitated gastric contents can occur with the LMA in place if an adequate depth of anesthesia is not maintained. On the other hand, the LMA protects the airway from secretions from above the larynx, such as injected methylene blue or fluorescein dye administration during probing and irrigation of blocked nasolacrimal ducts [112]. It allows the surgeon complete access to the eye and nose for probing of blocked nasolacrimal ducts, silastic intubation, examination under anesthesia, strabismus repair, chalazion drainage, and other minor oculoplastic procedures. The shaft of a flexible LMA may be taped closely to the patient’s chin, maintaining a low airway profile, thereby further increasing surgical work space.
Anesthetic maintenance can be conducted with an LMA using spontaneous or controlled ventilation. The LMA “leaks” when the peak inflating pressure exceeds 15–20 cm H2O. When used with controlled ventilation, the LMA can be used in conjunction with neuromuscular blocking agents, though many practitioners view the necessity to control ventilation as an indication for tracheal intubation. At the end of the procedure, the LMA may be removed while the patient is still anesthetized (“deep removal”) or fully awake. The decision is based on the anesthesiologist’s experience, patient characteristics, and recovery area standard practices. A recent systematic review showed that the safety profile is similar for either approach; coughing was less frequent with deep removal, and airway obstruction was less frequent with awake removal [113].
Tracheal Intubation
Tracheal intubation is the most definitive method for airway management in children. As with LMAs, a variety of tracheal tubes are available to meet specific needs (Fig. 5.2). A number of systems have been used to predict the appropriate tracheal tube size for infants and children. Cole’s formula (age (yr) /4 + 4) correctly predicts the correct uncuffed tube size approximately 60% of the time and 95% of the time is within 0.5 mm of the correct size [114]. Uncuffed tracheal tubes have traditionally been recommended for infants and children until the age of 8–10 years, in order to mitigate against post-extubation stridor. This concept has been refuted in studies showing that the incidence of post-extubation stridor is the same between uncuffed and cuffed tracheal tubes [115]. Furthermore, using cuffed tubes resulted in fewer tube exchanges due to leaks around the tube, lower fresh gas flow usage, and less waste gas contamination [116]. As a result, most pediatric anesthesiologists use cuffed tubes downsized 0.5–1.0 mm ID from uncuffed ones unless there are strong reasons not to [117]. To ensure that the tube fits properly, the pressure at which gas leaks around the tube is measured (leak test) and the cuffed filled to allow a leak between 15 and 25 cm H2O [118].


Fig. 5.2
Comparison of different endotracheal tubes. From left to right, trademarked brands are in (): uncuffed tracheal tube (Mallinckrodt); cuffed tracheal tube (Mallinckrodt); microcuffed tracheal tube (Halyard Microcuff); tracheal tube adapter used to connect to a shortened tube; wire-enforced flexible tracheal tube (Bivona); bottom, curved nasal RAE tube (Rusch); top, curved oral RAE tube (Mallinckrodt)
For many pediatric oculoplastic procedures, it is convenient to use a tracheal tube with a preconfigured bend, such as the oral RAE tube (Ring-Adair-Elwyn , Mallinckrodt). These tubes are inherently less secure, especially in the smaller sizes, but allow the anesthesia circuit to remain out of the way of the surgeon. Care must be taken during positioning not to dislodge the tube. Flexion and extension of the head in infants may move the distal tip of a tracheal tube by ±1.7 cm and may lead to unintended endobronchial intubation or extubation [119]. Wire reinforced (anode) tubes provide an alternative to the oral RAE tube. They allow more precise positioning of the tip of the tube since they do not have a preformed bend and will not kink or occlude when the head is repositioned. Anesthesiologists should continuously monitor airway pressures and breath sounds for signs of endobronchial intubation or tracheal tube obstruction during surgery.
The Difficult Pediatric Airway
Normal Anatomy and Physiology
The anatomic differences between the normal pediatric and the normal adult airway present several challenges for the anesthesiologist. The child’s larynx is more cephalad and has an anterior tilt compared to that of the adult, making visualization by direct laryngoscopy more difficult. Furthermore, the infant’s larger tongue in proportion to the oral aperture and floppy omega-shaped epiglottis can also make intubation more challenging.
To support a higher basal metabolic rate of 7–8 mL O2/kg/min compared to 3.5 mL O2/kg/min in the adult, infants and young children must increase their minute ventilation by doubling respiratory rate. This places a greater physiologic demand on the airway. Functional residual capacity (FRC) , which provides oxygen storage, is approximately the same on a per kilogram basis in infants and adults. During intubation, the anesthesiologist relies on the FRC to provide oxygen while the patient is apneic. Because of the doubled oxygen consumption and the fixed per kilogram FRC, the anesthesiologist has a shorter amount of time to secure the infant airway before oxygen desaturation begins [120].
Because of the major morbidity and mortality associated with airway loss in any patient, anesthesiologists are always concerned with the nature of a patient’s airway. Concern is heightened for those pediatric patients with congenital syndromes or abnormal airways, where additional factors may preclude straightforward ventilation and/or intubation.
Airway Evaluation
Evaluation of the airway begins during the preoperative assessment. The patient’s medical history, past anesthetics with particular reference to airway management, and airway examination are vital in this process. A patient’s ability to lie flat and sleep without substantial airway obstruction suggests that the patient may be safely anesthetized prior to insertion of a tracheal tube. Induction techniques used in the past, specific nature of the airway difficulty (mask ventilation versus intubation), and airway equipment used (nasal or oral airways, laryngeal mask airway, type of laryngoscope blade, fiber-optic bronchoscope, smaller tracheal tube size) should be noted. Since the airway may change with time, the most recent anesthetic record will likely provide the most useful information. If several years have elapsed since the last anesthetic and signs and symptoms have changed, an entirely new approach to the airway may be required. The anesthesiologist should review the records, perform a thorough airway examination, and discuss the proposed anesthetic with the patient and family. Induction of anesthesia should take place in a setting where the anesthesiologist will have access to all necessary equipment and the assistance of other experienced anesthesiologists and consulting otolaryngology surgeons.
Management of the Difficult Airway
The American Society of Anesthesiologists (ASA) developed the difficult airway algorithm as a set of guidelines for the management of the recognized and unrecognized difficult airway [109]. This algorithm was designed for use in adults and presents a general airway management algorithm with an emphasis on planning, preparing, and having multiple backup strategies. Among its recommendations are availability of experienced personnel and equipment, recognizing the likelihood and clinical impact of difficult mask ventilation, supraglottic airway (LMA) placement, laryngoscopy, intubation, and surgical access, and supplemental oxygen delivery during management (Fig. 5.3).


Fig. 5.3
Difficult airway algorithm . Decision points to consider: 1. Awake intubation vs. intubation after induction of general anesthesia. 2. Noninvasive technique vs. invasive techniques for the initial approach to intubation. 3. Video-assisted laryngoscopy as an initial approach to intubation. 4. Preservation vs. ablation of spontaneous ventilation. a. Other options include (but are not limited to) surgery utilizing face mask or supraglottic airway (SGA) anesthesia (e.g., LMA), local anesthesia infiltration, or regional nerve blockade. b. Invasive airway access includes surgical or percutaneous airway, jet ventilation, and retrograde intubation. c. Alternative difficult intubation approaches include (but are not limited to) video-assisted laryngoscopy, alternative laryngoscope blades, SGA (e.g., LMA or ILMA) as an intubation conduit (with or without fiber-optic guidance), fiber-optic intubation, intubating stylet or tube changer, light wand, and blind oral or nasal intubation. d. Consider re-preparation of the patient for awake intubation or canceling surgery. e. Emergency noninvasive airway ventilation consists of a SGA (Adapted from Apfelbaum et al. [109], with permission from Wolters Kluwer Health, Inc.)
While many parts of the ASA difficult airway algorithm have their place in pediatric practice, there are some aspects that may not easily apply to infants and children. Most importantly, awake/sedated intubation and tracheostomy facilitated by local anesthesia and sedation play prominent roles in the management of adult patients with difficult airways, but have limited application in children. Pediatric patients who are not expected to present difficulties with mask ventilation may undergo an inhalation induction with O2/N2O/sevoflurane. Spontaneous ventilation offers a margin of safety during induction and may be more difficult to achieve using intravenous agents. Although an intravenous induction may not be chosen, it is extremely helpful to have a working intravenous line in place prior to managing the difficult airway in order to administer antisialogues, sedatives, or emergency medications. To maintain a patent airway, the anesthesiologist may need to apply continuous positive airway pressure (CPAP) between 5 and 15 cm H2O and relieve airway obstruction from the tongue with a jaw thrust maneuver or an oral airway. If ventilation can be controlled with bag and mask or LMA, then the use of muscle relaxants may be considered prior to attempting tracheal intubation [121].
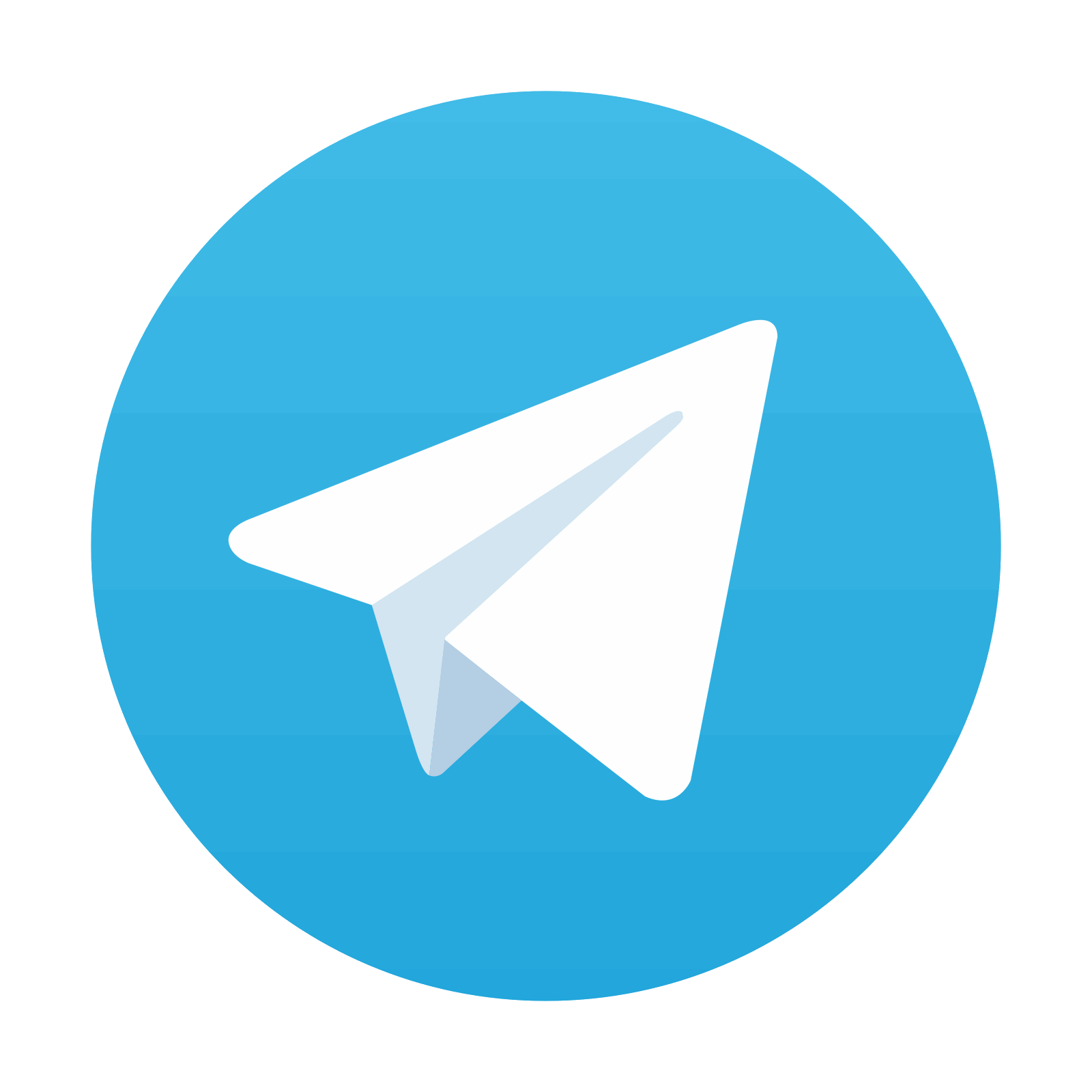
Stay updated, free articles. Join our Telegram channel

Full access? Get Clinical Tree
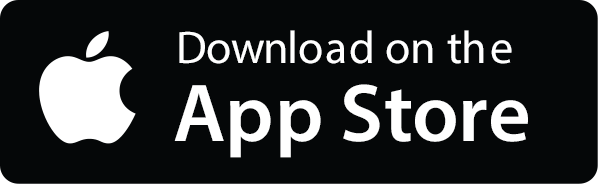
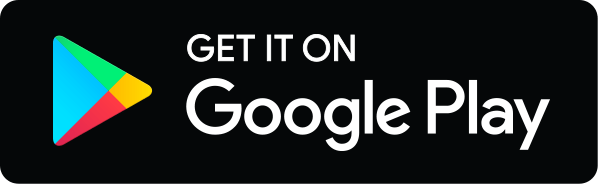