Passive role
Robotic assistance is limited in scope, and generally aims to assist the surgeon with a specific noninvasive task. Inherently, these systems are low risk
Restricted (semi-active)
The robotic system is more integrated into the surgical procedure, and is involved in more invasive tasks such as stereotactic localization or hand stabilization. These systems receive direct input from the surgeon and tend to limit action. Inherent risk is moderate
Active role
The robot is intimately involved in the surgical procedure. There is a greater freedom of function and movement, and the robot is generally in direct contact with the patient while the surgeon operates the machine remotely
Degrees of Freedom
Maneuverability of surgical instruments becomes increasingly difficult in more confined spaces and with nonlinear trajectories (not in the surgeon’s line of sight). Each degree of freedom allows movement in an additional dimension. One degree of freedom allows for unidirectional motion, for instance, along a linear trajectory. Conventional endoscopic and microscopic procedures in otolaryngology and other surgical fields generally provide four degrees of freedom. Surgical robotic systems such as the da Vinci system (Intuitive Surgical, Mountain View, USA) provide six degrees of freedom. The effect is that of a “wristed” distal end of the surgical instrument that moves much like the human wrist, allowing the head of the instrument to “turn corners” and operate beyond the traditional line-of-site limitation. This effect has potential benefits in small, narrow, anatomic regions commonly encountered in otolaryngology.
Workspace and Resolution
Workspace refers to the area that a robot can physically access. This is limited by the length and maneuverability of the surgical arms. Also, configurations of the surgical arms are limited by interference between the arms outside of the surgical field. Resolution deals with the magnitude of robotic arm movement. Smaller surgical fields will require more precise movement, and will therefore require finer resolution of robotic movement.
Inertia and Stiffness
Inertia and stiffness are greater concerns in the engineering world than in the surgical world, and are important when calculating the forces needed to accelerate or decelerate the robotic arms. Robotic arms that need to move quickly will either need to be lighter or have a larger motor to generate greater force.
Speed and Force
Much like the transmission of an automobile, robots have a transmission that alters the gear ratio between the motor and the surgical arm. This allows forces to be scaled up or down, changing the resolution of the robotic arm movement. Like in an automobile, there is a trade-off between speed and force. In lower gears, there is an emphasis on force, whereas in the higher gears the purpose is speed. Speed and force are not mutually exclusive, but can be limited by expense.
Dynamic Range
The ratio of the highest and lowest force produced by a robot is known as the force dynamic range. The human hand has a high dynamic range, which can be difficult to replicate mechanically.
Advantages and Disadvantages of Robotic Systems
Advantages
Image Guidance and Stereotactic Orientation of the Surgical Instrument
Stereotactic image guidance systems are widely used in clinical practice today. In the broadest sense, image guidance can be considered “robotic,” though the combination of robotic systems discussed here with image guidance is an even more powerful tool than either independently. Planning complex surgical trajectories preoperatively using imaging data can be coupled with robotic systems that carry out the surgical maneuvers in an active-role system.
Instrument Stabilization and Tremor Control
Studies monitoring the precision of the human hand demonstrate decreased reliability within 100 μm of a target for senior surgeons [7]. Accuracy further deteriorates due to the natural development of intention tremor with fatigue. Instrument stabilization can increase precision to 10 μm, which can be maintained over time with a computer-assisted robot to subtract intention tremor from the surgeon’s movements independent of fatigue. In addition, robotic systems can filter the natural 200 Hz eye motion to stabilize the visual field [8].
Binocular Endoscopic Vision
While open and microscopic procedures do allow binocular vision, endoscopic and laparoscopic technology typically suffers from loss of three-dimensional vision and depth perception. The da Vinci system employs stereoscopic video telescopes [8, 9]. Two 5 mm cameras sit within a 12 mm laparoscopic arm. Zero degree and 30° stereoscopic video telescopes are available providing a magnified 60° field of view, or a narrower field of view with higher magnification, depending on the nature of the operative field.
Motion Scaling
Engineers and surgeons interested in microscopic robotic surgery have hypothesized that scaling the surgeon’s hand movements would improve technical abilities and outcomes by converting gross hand movements into fine surgical motion. The da Vinci system can be used in a 1:1 or 4:1 mode although little is known about how beneficial motion scaling is in improving accuracy.
Telepresence and Telementoring
Telepresence refers to the ability of the surgeon to operate at a site that is remote from the patient. Early in the development of surgical robotic, the United States Department of Defense and the National Aeronautics and Space Administration, NASA, became interested in exploring this technology to decrease wartime morbidity and mortality by allowing the surgeon at a base hospital to operate on wounded soldiers in the field and astronauts in orbit, respectively. Telementoring is a logical extension of telepresence and refers to the ability to disseminate new surgical techniques to surgeons anywhere in the world.
Disadvantages
Expense
The cost-effectiveness of robotic surgery has often been questioned. Expenses must consider both upfront and maintenance costs. Despite increasing utilization in the head and neck, a dedicated otolaryngologic device is usually economically unfeasible for most centers. Collaboration between surgical departments as well as research grants can help defray some of the cost burden of individual specialties while disbursing economic risk.
Size
Studies by Hockstein et al. demonstrated the feasibility of laryngologic surgery using 5 mm diameter instruments [10]. According to their preliminary work this is the maximum diameter capable of operating in the extreme confines of otolaryngologic surgery. Five millimeter robotic instrumentation has only recently become available, spurring the use of this technology in otolaryngologic applications. Future development of even smaller instruments may have additional benefits for accessibility and maneuverability.
Loss of Force Feedback/Haptics
One drawback of currently available robotic technology is loss of tactile perception of the tissue being manipulated. Experienced robotic surgeons often feel that this is largely compensated for by the improved visual information provided by the stereoscopic camera. Although sensory substitution methods, such as a visual color scale on the monitor, provide some improvements, there remains a need to develop true haptic feedback between operator and machine.
Specific Surgical Robotic Systems
Specific systems range from highly specialized, task-limited instruments to larger telerobotic surgical systems capable of multitasking application. It is important, therefore, to introduce a few of the individual systems that are in use today, with specific emphasis on ones with applications in otolaryngology. The systems are discussed in order of increasing robot responsibility as described above by the role-based classification. The first systems were basic, single function devices. After FDA approval in 2009 for use in the head and neck, active telerobotic systems have come to dominate the field in various otolaryngologic applications.
One of the first commercially available applications of surgical robotics was AESOP, Automated Endoscopic System for Optimal Positioning (Computer Motion, Santa Barbara, USA). AESOP offers a steady platform for a laparoscope that could be controlled by a surgeon via foot pedal and later voice activation, eliminating the need for an assistant to maintain a steady image with proper orientation. The AESOP system later became integrated into the Zeus surgical robotic system.
Robotic drilling and milling platforms are available for certain surgical applications. ROBODOC (Curexo Technology, Fremont, CA), used in orthopedic surgery, was the first such device approved by the FDA [4]. Its function is to mill femur shafts during total hip arthroplasty to improve accuracy and reliability over hand reaming. Neuromate (Integrated Surgical Systems, Sacremento, CA) is the modern derivative of neurosurgical robots used to place probes, electrodes, and drills under stereotactic guidance into the brain. The newest version of Neuromate uses ultrasonic stereotactic registration obviating the need for painful head frames.
Telerobotic systems have drastically changed the size and complexity of surgical robotics. These systems provide a complete interface between the surgeon and the patient and have changed the nature of robotics from adjunctive to inherent in the surgical process. This “master–slave” configuration converts the surgeons hand movements at the “master” console into a digital signal that is sent to the “slave” robotic arms and converted to movement of the surgical instruments. The digital signals are processed and relayed through a computer system [8, 9]. Previously, the two major competing systems in telerobotic and telepresence surgery were the da Vinci surgical system and the Zeus system (Computer Motion Inc, Santa Barbara, USA). In 2003, a corporate merger between Intuitive Surgical and Computer Motion eventually led to phasing out of the Zeus platform in favor of the da Vinci system.
The da Vinci console is designed to completely engross the surgeon in the surgical field. The console hood acts as “blinders” so the surgeon is unable to see the remainder of the operating theater. The advantage is, in theory, to make the surgical movements more intuitive with fewer distractions. The 3D endoscopic technology incorporates two small cameras in a single endoscope. The da Vinci system isolates the images from each camera which are then independently fed to left and right visual fields in the surgical console.
The da Vinci system allows for the use of up to four arms, one which holds the endoscope, two that hold the left- and right-hand instruments, and a fourth arm which can be used to hold a retractor. Given the confines of head and neck operative fields, in most cases only two arms are used in addition to the endoscopic camera. The surgeon has control of the instrument arms and the endoscopic camera and can toggle between these with the use of foot pedals. Additional instrumentation such as a suction and forceps are provided by the surgical assistant who stands at the head of the bed.
One barrier that robotic technology was able to overcome is the fulcrum effect with laparoscopic instruments. As the length of surgical instruments increased to accommodate minimally invasive surgery the body wall trocar system created a fulcrum point that affected the movement of surgical instruments so that the surgeon’s hand motion was the reverse of the motion at the distal end of the laparoscopic instrument. Because the hand movements in a telerobotic system are not directly connected to the instruments the controllers can be designed to “grasp” the surgical instruments at any point along the robotic arm. The da Vinci system overcomes the nonintuitive fulcrum effect by virtually “holding” the instruments at their distal end. In addition, the ends of the robotic arms are “wristed” allowing human wrist-like movement within the surgical field. The real effect is a serially oriented six degrees of freedom robotic arm that allows pitch, yaw, roll, and in-out movement of the robotic arm at the instrument tip. In addition, instruments with grasping or cutting abilities add a seventh degree of freedom [8]. The Zeus system on the other hand was designed to virtually hold the instruments much like a surgeon would in laparoscopic surgery. This resulted in maintenance of the fulcrum effect with only four degrees of freedom in the surgical arms without the articulating instrument tips.
Applications of Surgical Robotics
History and General Principles
Studies by Hockstein et al. described the feasibility of using the da Vinci robot to access and manipulate the structures of the oropharynx, hypopharynx, and larynx [10, 11]. Their first study evaluated the technical aspects of operating within the confines of the oral cavity and upper airway using presently available da Vinci surgical equipment [10]. The four variables in this study included: (1) Retractor: Lindholm laryngoscope versus McIvor mouthgag. (2) Endoscope: 0° 2D scope versus 30° 3D scope. (3) Surgical instrument diameter: 8 mm versus 5 mm. (4) Positioning of the robotic arms in relation to the “patient.”
Using an airway management mannequin, they demonstrated that the combination of these variables that allowed for the greatest visualization and surgical access was a McIvor mouthgag with tongue blade, 30° 3D endoscope, and two 5 mm instruments, with the operating table rotated 30–45° relative to the robotic arms. This allowed for visualization of the hypopharynx, supraglottis, glottis, and anterior commissure. Other studies validated use of different mouth gags, such as the Crowe-Davis or the Feyh–Kastenbauer, rather than a traditional tubed laryngoscope. Furthermore, by minimizing the size of the surgical arms they were able to manipulate the laryngeal structures, and suture between the vocal fold without difficulty.
Subsequently, Hockstein et al. performed proof-of-principle dissections on a human cadaver using the previously noted surgical setup [11]. Six procedures were performed and timed: (1) Bilateral true vocal cord stripping, (2) Rotation of a mucosal flap from the epiglottis to the anterior commissure, (3) Partial vocal cordectomy, (4) Arytenoidectomy, (5) Partial epiglottectomy and thyrohyoid dissection, and (6) Partial resection of the base of tongue with primary closure. Though they did not compare these procedures with conventional open or laser surgeries, they reported greater ease of operation with the wristed instruments, tremor stabilization, and binocular vision compared to the author’s prior experience. Also, they noted the length of operation was comparable to conventional techniques with a similar safety profile in terms of hemostasis and risk of patient injury [12].
From these initial experiments, the practice of transoral robotic surgery (TORS) was popularized. The results of institutional patient trials at the University of Pennsylvania were presented to the Food and Drug Administration, leading to the 2009 approval of the da Vinci system for treatment of head and neck benign disease and select T1 and T2 malignancies. Since that time, there has been a flurry of publications from multiple institutions detailing the use of surgical robotics in otolaryngologic applications. A review of the literature shows not only technical descriptions of robotic use, but also recent manuscripts regarding the clinical outcomes, comparisons to traditional approaches, as well as discussion of the logistical roadblocks to implementing robotic techniques into existing surgical programs.
There are several general principles of robotic head and neck surgery performed with the da Vinci system regardless of the involved anatomic subsite, as outlined by Newman et al. [13]. These include issues of airway management, intra-operative patient safety measures, and room setup (Fig. 1).
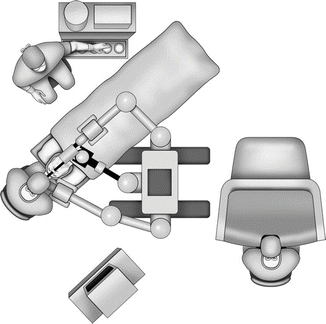
Fig. 1
Diagram of the operating room setup of the da Vinci system with primary surgeon at the console and assistant at the head of the patient. Reprinted from Hockstein NG and BW O’Malley, Transoral robotic surgery, Operative Techniques in Otolaryngology—Head and Neck Surgery, 2008, with permission from Elsevier [13]
Laryngology
Laryngology has benefited tremendously from the introduction of the microscope, microscopic instrumentation, and the CO2 laser into clinical practice. By coupling the operating microscope to the laser, Strong, Jako, and Vaughan showed that a variety of lesions could be excised or ablated from laryngeal cancers to vocal cord papillomas [14]. One major disadvantage of microinstruments and also the CO2 laser is that they cannot turn corners and are restricted to line-of-sight working corridors. Overcoming this obstacle is seen as the primary advantage of using surgical robots in the upper airway.
Transoral laryngeal surgery significantly predates the use of robotics in otolaryngology. Partially in response to a trend toward functional organ preservation in the treatment of laryngeal malignancy, transoral endoscopic partial laryngectomy techniques were developed to spare patients from both total laryngectomy and the morbidity of open partial laryngeal surgery such as the need for tracheostomy and feeding tubes. These techniques were pioneered by the work of Drs. Stuart Strong and Charles Vaughn as well as Wolfgang Steiner among others [15, 16]. Indications for partial laryngectomy are contingent on loco-regional staging and are out of the scope of this chapter. However, it is important to note that the use of robotics in laryngeal surgery, as well as other head and neck subsites, simply represents an alternate technical method, with unique advantages and drawbacks, for achieving the outcomes previously described by the proponents of transoral partial laryngectomy techniques.
Laryngeal TORS has been described for benign disease such as respiratory papillomatosis and laryngeal schwannoma, but the vast majority of the literature concerns the treatment of supraglottic squamous cell carcinoma. Weinstein et al. reported three cases of TORS resection of T2 and T3 supraglottic tumors with successful surgical exposure and access as well as achievement of negative margins [17]. TORS total laryngectomy has also been described, but experience with this technique is limited [18].
Several series reporting experience with TORS supraglottic laryngectomy (TORS-SL) are now available. Exposure is facilitated by the routine use of transnasal intubation to keep the endotracheal tube posterior in the field and the use of the Feyh–Kastenbauer retractor. Clinical outcomes are encouraging. Mendelsohn and colleagues report a series of 18 patients who underwent TORS-SL for T1 and T2 supraglottic tumors [19]. Negative margins were obtained in all patients and no local recurrences on 2-year follow-up. Ozer and colleagues report largely similar results in a series of 13 patients [20]. All achieved negative margins but one patient required conversion to an open approach due to extensive pre-epiglottic space invasion (Fig. 2).
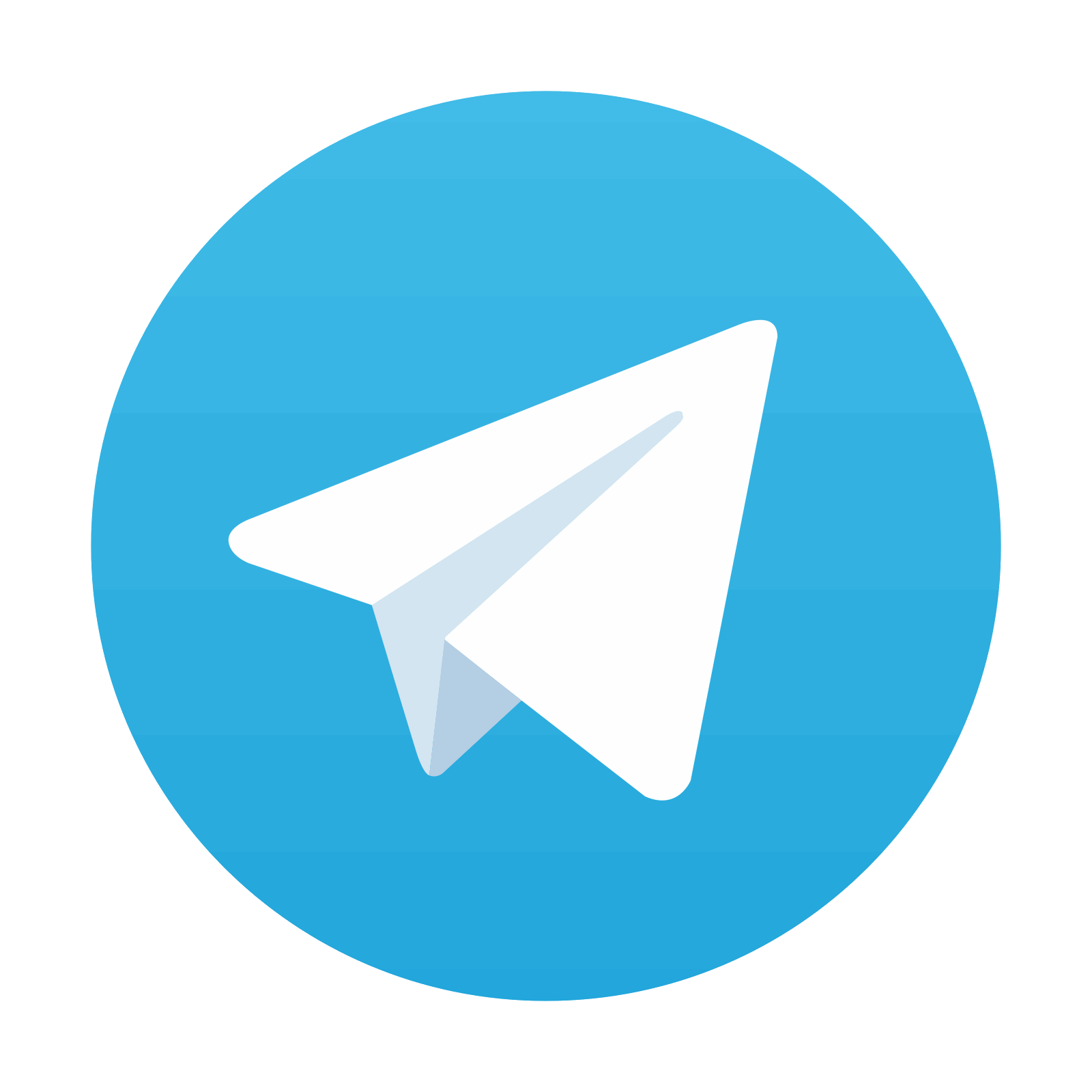
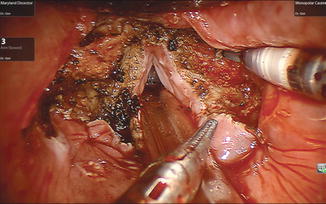
Only gold members can continue reading. Log In or Register to continue
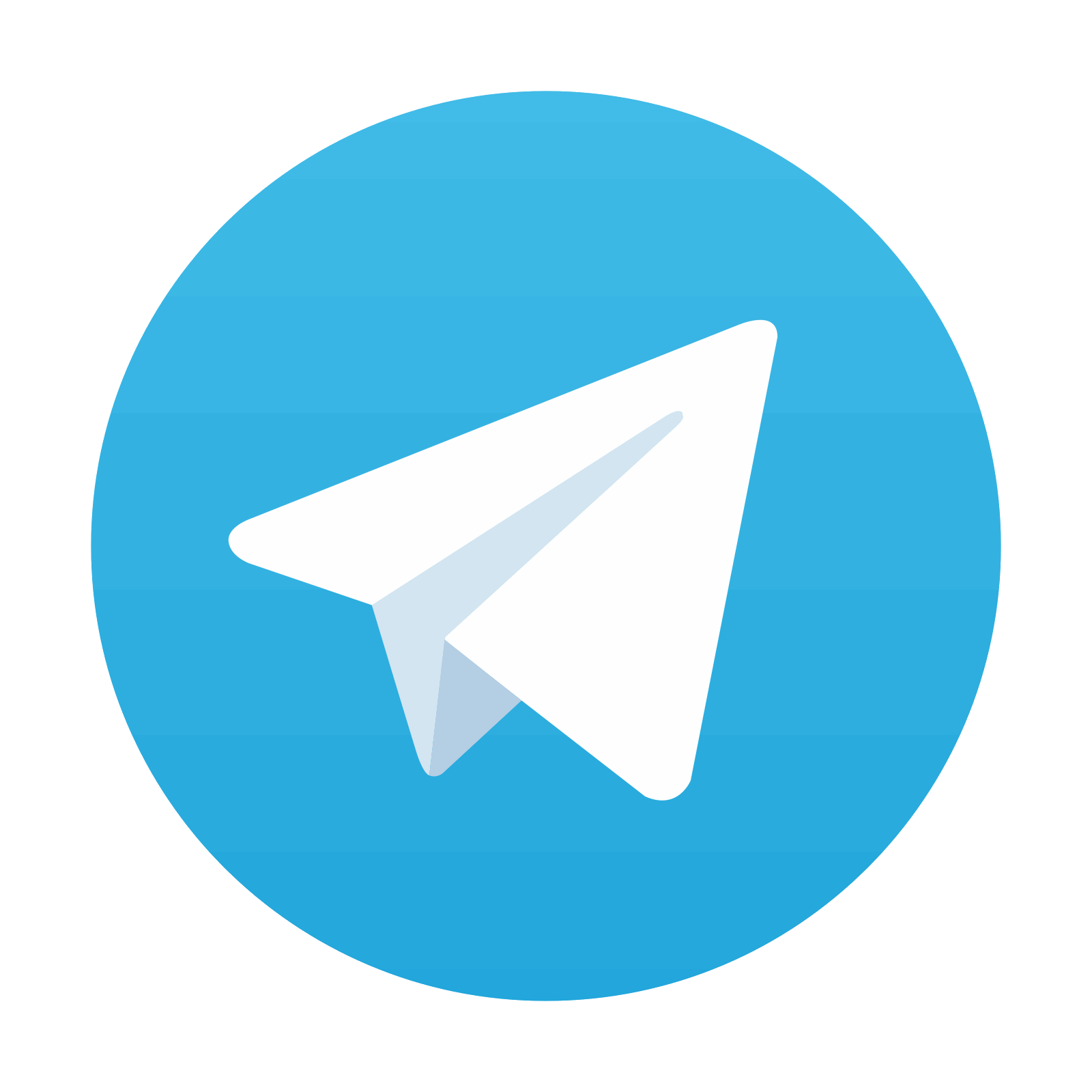
Stay updated, free articles. Join our Telegram channel

Full access? Get Clinical Tree
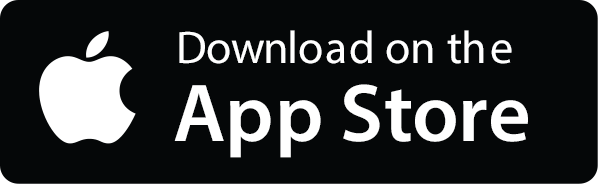
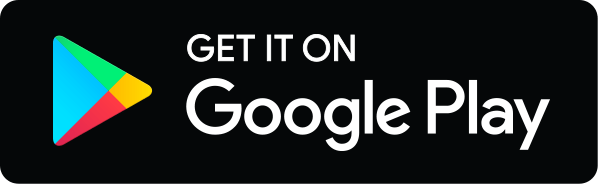