Family tree of an autosomal recessive hearing disorder
16.1.3 Clinical Examination
During the examination neonates and smaller children will usually sit on the lap of the caregiver. Older children may sit alone on the examination chair.
Examination begins with an assessment of the head and neck. Any craniofacial abnormality is to be regarded as suspicious when found in relation with a hearing disorder. For example, heterochromia of the iris and a patch of white hair are observed in Waardenburg syndrome. An enlarged thyroid gland may be a sign of hypothyroidism (Pendred syndrome). Microgenia is related to Pierre Robin sequence. Malformations of the jaw occur in syndromes such as Franceschetti or Goldenhar. Branchiogene developmental disorders such as cervical cysts or fistulas may be related to hearing disorders as well.
The pinnae and their surrounding area should be examined for malformations such as pre-auricular pits or pre-auricular tags, atresia or microtia.
Pre-auricular pits may be connected with a fistula and an epidermal cyst at its end. Some authors recommend surgery before superinfection (Shoman et al. 2014).
I: malformation of the external ear with identifiable structures and a small but present external ear canal
II: partially developed ear with a closed or stenotic external ear canal
III: absence of the external ear with a small tissue structure, absence of the external ear canal
IV: absence of the total ear
These abnormalities may be associated with middle ear malformation and congenital or progressive hearing loss (Germiller 2007).
A binocular inspection with the microscope is essential to assess the ear canal and the tympanic membrane. The width or possible atresia of the external canal should be examined. Cerumen should be removed until at least a part of the tympanic membrane is visible—if tolerated.
The tympanic membrane is judged for decline, translucency, colour, integrity and morphology of manubrium of malleus. The most common pathological findings are effusions and acute or chronic otitis media.
By inspection of the nose and nasopharynx, mucosal swelling, erythema and secretion may give hints to allergies, inflammation or reflux (Alexiades and Hoffmann 2008).
A nasal pit may be associated with a fistula and an epidermal cyst at its end extending into the nasal cavity or even intracranially. Because of the risk of infection, surgery should be performed; preoperative MRI is strongly recommended (Rizzi and Dunham 2014).
The size of the adenoids should be assessed. Choanal opening can be judged indirectly by strained expiration through the nose on a Link mirror. If necessary flexible or rigid endoscopes can be used. Examination of the oral area should include the teeth, tongue and tonsils. Additional attention should be paid to the soft and hard palate (high palate, short velum, cleft palate). Cleft or bifid uvula may hint to submucous cleft palate. Palpation and inspection of the velum during phonation are useful to exclude submucous cleft palate. Submucous cleft palate may affect the soft or the bony palate.
If a hearing disorder has been diagnosed, a full clinical examination is recommended to look for signs of syndromal disease, for example, café au lait spots in neurofibromatosis (Ruben 2001; Potsic and Lando 2012; Gibbin 2007).
During the examination every care should be taken to ensure that the child remains as comfortable as possible. In this way the cooperation of the child is maintained for the further audiological evaluations.
16.2 Basics of Technical Standards and Calibration
Selection of standards important in audiometry
ISO 266 | Preferred frequencies |
ISO 389 | Standard reference zero for the calibration of audiometric equipment (all kinds of signals and sound sources) |
ISO 7029 | Statistical distribution of hearing thresholds as a function of age |
ISO 8253 | Audiometric test methods (pure tones, narrowband tones, frequency-modulated tones with air and bone conduction and one or more loudspeakers; minimum requirements of precision and comparability between different test procedures; basic methods of speech recognition tests and requirements for the composition, validation and evaluation (not the content) of speech material; noise for masking and as competing sound) |
EN 15927 | Services offered by hearing aid professionals |
ISO 16832 | Loudness scaling by means of categories |
EN 60118 | Hearing aids |
EN 60318 | Simulators of human head and ear (ear simulator for the measurement of earphones, acoustic coupler for the calibration of earphones, occluded-ear simulators, coupler for the measurement of hearing aids and earphones coupled to the ear by means of ear inserts, mechanical coupler for the measurement of bone vibrators, head and torso simulator for the measurement of hearing aids) |
EN 60645 | Equipment for audiometry: pure-tone audiometry, speech audiometry, test signals, high-frequency audiometry (8–16 kHz), instruments for the measurement of aural acoustic impedance/admittance, otoacoustic emissions and auditory brainstem responses |
EN 60942 | Sound calibrators |
EN 61094 | Specification for measurement microphones |
EN 62489 | Audio-frequency induction loop systems for assisted hearing |
European Committee for Standardization www.cen.eu.
International Organization for Standardization www.iso.org.
Sound and Vibration Standards acoustic-standards.co.uk/index.htm.
Freestd Standards Worldwide www.freestd.us.
For detailed overview see Wilber (2002). It is important to implement good calibration practice into the daily performance of audiometry. Various guidelines and protocols for audiometry mention calibration as part of essential practice (e.g. British Society of Audiology 2011a).
16.3 Pure-Tone Audiometry
16.3.1 Introduction
Pure-tone audiometry (PTA) is the most common test within audiology, apart from newborn hearing screening tests, and forms the backbone of audiological diagnosis and management. PTA is a behavioural test (i.e. responses require the conscious cooperation of the patient) of the threshold of hearing (the lowest level of an acoustic signal that the patient can hear). It is appropriate for patients of approximately 3–5 years of developmental age and older.
Various protocols and guidelines for PTA have been created by different international organisations, such as the American Speech-Language-Hearing Association (1978), British Society of Audiology (2011a), US National Health and Nutrition Examination Survey (National Centre for Health Statistics 2003), Ireland’s Health and Safety Authority (2007) and World Health Organization (2013). A well-structured PTA protocol, if correctly followed, has high test-retest reliability (Stuart et al. 1991), and intra-subject deviation of less than 10 dB is expected in >94% of subjects (Schmuziger et al. 2004). This article gives an overview of the core concepts of PTA which underlie most modern pure-tone audiometry protocols.
16.3.2 Resources
PTA is performed with an audiometer (which generates the sound stimuli), transducers (which deliver the stimuli to the patient) and a patient response button (which the patient presses to indicate that they have heard the sound stimulus). Audiometers can be stand-alone devices but are these days often attached to conventional computers and controlled by software, allowing results to be digitally recorded and connected with patient medical systems.

Beyerdynamic DT 48 headphones. Image copyright and with permission from Beyerdynamic

Etymotics ER-3C insert earphone. Image copyright and with permission from Etymotic Research, Inc.

Radioear B71 bone-conduction transducer. Image copyright and with permission from Radioear
Calibration of each audiometer (together with its dedicated transducers) must be performed at least annually (Stage B calibration, defined in ISO 8253-1 (2010)) in order to ensure the accuracy of the stimuli delivered to the patient. Simple checks (also known as Stage A checks) ought to be performed before each session of use to flag any technical issues or drift in the calibration.
PTA is typically performed within a sound-treated room, which reduces both background noise from outside the room and resonance within it. Soundfield audiometry is not covered in this article (see Sect. 16.4 for more details).
16.3.3 Key Principles of PTA
Stimulus Type
The stimuli (also known as ‘signals’ or ‘tones’) used in PTA are pure tones (i.e. sine waves) (see Sect. 1.4), which are the most frequency-specific stimuli possible.
Frequency and Level of Stimulus
PTA is typically performed over the frequency range 250 Hz to 8 kHz and includes frequencies at each octave (250, 500, 1000, 2000, 4000 and 8000 Hz). Additional testing at intermediate frequencies, such as 1.5, 3 and 6 kHz, is sometimes done for specific purposes, such as to enable the gain of hearing aids to be set more accurately. Air-conduction testing can be performed at all of the above standard frequencies and is occasionally performed at higher frequencies (known as extended high-frequency audiometry), for example, in younger patients at risk of sensorineural hearing loss, such as those receiving ototoxic medication. Specific headphones are required for high-frequency audiometry, such as the Sennheiser HDA 200.
Bone-conduction testing is typically only performed between 500 Hz and 4 kHz, though some centres test up to 6 kHz. Bone-conduction signals can lead to vibrotactile sensation at lower frequencies (Boothroyd and Cawkwell 1970) and be airborne at higher frequencies (Lightfoot 1979) because of poor distortion performance of bone-conduction transducers at both low and high frequencies (Lightfoot 2000; Lightfoot and Hughes 1993). For this reason, precautions should be taken when using bone conduction above 2 kHz, such as covering the test ear with a supra-aural headphone (BSA 2011a). Both air- and bone-conduction stimulations are limited in their maximum output levels (air conduction is limited to approx. 120–130 dB HL output and bone conduction to approx. 60 dB HL output (both varying at different frequencies and dependent on audiometer manufacturer).
16.3.4 Test Procedure
Before Testing
Otoscopy before PTA is recommended. PTA is not advised in cases of obstructed ear canal (owing to a risk of falsely raised air-conduction thresholds) or ear infection (owing to a risk of discomfort and cross infection). See more detailed protocols, such as those named in the introduction, for other specific contraindications.
The patient should be clearly instructed to press the response button every time he hears a sound, even if the sound is very quiet. Other responses, such as the patient’s raising their hand or saying ‘yes’, are reasonable if the patient is physically unable to push the button. Adaptations for patients who do not understand their task (e.g. younger children or people with cognitive difficulties) are covered in Sects. 16.4 and 16.24.
Care should be taken to position the transducers accurately in order to avoid artificially lowering the level of the signal delivered to the ear. Supra-aural headphones should rest on the pinnae with the centre of each headphone-loudspeaker in line with the auditory meatus. The bone-conduction transducer should be placed on the mastoid behind the ‘test’ ear (i.e. the ear which is being tested at the time), not touching any part of the ear and with as little hair between the transducer and the skin as possible.
Test Protocol


(a–d) Four examples of tone presentations, ascending trials and responses in pure-tone audiometry, in the search for the auditory threshold, based on the modified Hughson-Westlake procedure (Carhart and Jerger 1959). The + symbol indicates a patient response; the − symbol indicates no patient response. (a) Two positive responses at 10 dB HL out of two ascending trials: threshold = 10 dB HL. (b) Two positive responses at 10 dB HL out of three ascending trials: threshold = 10 dB HL. (c) Two positive responses at 5 dB HL out of three ascending trials: threshold = 5 dB HL. (d) Three positive responses at 10 dB HL out of five ascending trials: threshold = 10 dB HL
Presentation of the test tones should be arrhythmic with silences in between; otherwise the patient could (consciously or not) predict the presence of a tone which they cannot actually hear, thus resulting in falsely lowered thresholds. The tones should vary in length, and the patient should be instructed to hold the response button for as long as the sound is present, rather than just pressing it when they first hear the sound. This enables the tester to check the response to both the onset and offset of the tone, thereby increasing the certainty of the response.
This procedure is typically carried out beginning with air-conduction stimuli (starting at 1 kHz) on the suspected better ear. All desired air-conduction frequencies are usually tested on the same ear and then moving to the other ear and then finally using bone conduction.
If air-conduction thresholds are within the normal range (usually accepted as <20–25 dB HL; see Sect. 1.4 and the ‘Interpretation’ subsection), bone-conduction testing is usually not clinically indicated.
Masking
Munro and Agnew (1999) measured inter-aural attenuation levels (i.e. the level at which a signal played from a transducer to one ear could be ‘cross-heard’ by the other ear) of 40 dB for Telephonics TDH 39 supra-aural headphones and 55 dB for Etymotics ER-3A insert earphones. In clinical terms, this means that a large asymmetry in air-conduction thresholds between the two ears may mistakenly appear to be no greater than 40 dB (using supra-aural headphones) or 55 dB (using insert earphones). Masking is the essential process by which we can measure the ‘true’ threshold in these cases.
In masking, a noise (usually 1/3-octave narrowband noise, though white noise or others are used in some clinics) is played to the non-test ear at the ‘effective masking level’ (the lowest level of masking noise required to prevent the threshold-level pure-tone signal from being heard). The patient is asked to ignore the noise and only respond when they hear the pure-tone signals (played to the test ear). Using the common ‘plateau’ technique, the levels of noise and signal are increased to the point at which responses occur consistently at a particular signal level despite the noise level increasing further. This is taken as the true threshold.
Masking as part of bone-conduction testing is somewhat more problematic because the inter-aural attenuation of bone-conduction signals can be as low as 0 dB. Bone-conduction masking (in which a bone-conduction signal is masked by noise via an air-conduction transducer) is therefore essential in cases of asymmetrical air-conduction thresholds and where bone-conduction thresholds appear significantly better than air-conduction thresholds (the ‘air-bone gap’). It is, however, clinically inefficient to mask all incidences of an air-bone gap because they are actually more likely than not to occur (Studebaker 1967). Small air-bone gaps should not necessarily be taken as evidence of middle ear pathology (Margolis 2015). The BSA protocol therefore recommends bone-conduction masking only where there is a ≥10 dB gap between the not-masked bone-conduction threshold and the air-conduction threshold of either ear (BSA 2011a).
The tester must be careful not to play uncomfortably loud sound into the non-test ear and must be aware of the risks of central masking and cross-masking. In central masking the patient is unable to identify a tone when masking noise is present. In cross-masking the masking noise is itself cross-heard, with the effect of masking the test ear. In cases such as this, masking to find the true threshold may not be possible.
A more comprehensive guideline for masking is beyond the scope of this article. See specific protocols (e.g. BSA 2011a) for further detail.
16.3.5 Recording the Results of PTA
The Audiogram

An audiogram showing hearing thresholds for a hypothetical bilateral high-frequency sensorineural hearing loss with a low-frequency conductive component on the left. Results for the right ear are shown in red on the left side and results for the left ear in blue on the right side. The circles and crosses represent air-conduction thresholds; triangles represent not-masked bone-conduction thresholds; square brackets represent masked bone-conduction thresholds. These symbols are standard in the UK. Different symbols are used in different countries and clinics
Interpretation of Normal Range of Hearing
Various descriptions of the ‘normal range’ of hearing can be found (see Sect. 14.1) and are generally accepted as being hearing thresholds better than 20–25 dB HL.
Interpretation of Conductive Hearing Loss

Audiogram showing typical hearing thresholds found in conductive hearing loss caused by otitis media with effusion. This audiogram shows right-sided air-conduction thresholds (circles) and masked bone-conduction thresholds (square brackets)
Interpretation of Sensorineural Hearing Loss
Where air- and bone-conduction thresholds are outside of the normal range to the same degree, a sensorineural hearing loss is indicated (see Fig. 16.6, right ear).
Interpretation of Mixed Hearing Loss

Audiogram showing possible hearing thresholds found in mixed hearing loss. This audiogram shows right-sided air-conduction thresholds (circles) and masked bone-conduction thresholds (square brackets)
Different threshold levels are typically found at different frequencies. For example, otitis media with effusion is typically characterised by conductive hearing loss in the lower frequencies and normal thresholds in the higher frequencies (see Fig. 16.7). Presbyacusis (age-related sensorineural hearing loss) is characterised by normal or near-normal-hearing thresholds in the low frequencies and a sensorineural hearing loss affecting higher frequencies (see Fig. 16.6, right ear).
16.3.6 Limitations of PTA
As with all audiological tests, the cross-check principle (Jerger and Hayes 1976), which encourages the use of other tests (such as tympanometry; see Sect. 16.9) and the subjective impression made by the patient to corroborate the result, is worth following. As the test results rely on behavioural responses, falsely raised thresholds (whether conscious or not) could be obtained. See Sect. 15.5 for insight into nonorganic hearing loss (NOHL) and Lightfoot and Kennedy (2006) on the use of cortical electric response audiometry (which measures the electrical response to auditory stimulation within the auditory cortex) as ‘objective pure-tone audiometry’. Expectation bias can lead to inaccuracy during testing (Margolis et al. 2015), and there is a risk of responses being falsely interpreted by the tester (especially where time pressure and insufficient training are factors).
Classic pure-tone audiometry only tests the threshold of hearing in response to pure tones. Although this is sufficient for the diagnosis of many audiological conditions, it does not provide full evidence about the patient’s functional hearing (e.g. his/her ability to process suprathreshold speech and other functional sounds or their tolerance of loud sound). See Sect. 16.7 for information on speech audiometry. Loudness investigations are outlined in the following subsection.
16.3.7 Loudness Tests
‘Loudness’ refers to the subjective perception of a sound, whereas ‘sound level’ is an objective measure (see Sects. 1.3 and 1.4). Uncomfortable loudness level (ULL) (also known as ‘loudness discomfort level’ (LDL)) testing measures the lowest level at which a sound becomes uncomfortable for the subject. This can be useful in providing additional accuracy regarding the patient’s degree of loudness recruitment (in which a small increase in sound intensity is perceived as a large increase, as is typically found in sensorineural hearing loss) (see Sect. 1.4), thereby informing hearing aid gain settings, and is also often used in cases of suspected hyperacusis (see Sect. 15.3).
The basic procedure of ULL testing is to increase the level of a sound stimulus gradually until it just becomes uncomfortably loud for the patient (BSA 2011b). It is important to remember that the ULL is not the same as the threshold of pain. Agreeing an appropriate response method with the patient and issuing clear instructions are obviously crucial. ULLs are usually shown on the audiogram using the and
symbols for right and left ears, respectively. Sherlock and Formby (2005) surveyed previous literature and proposed a normal ULL of around of 100 dB HL, but they noted considerable variance in normal ULLs reported between different studies across different signals, frequencies and transducers.
Loudness scaling seeks to investigate the subjective loudness of sound in more detail. Various scales exist, and most use verbal descriptors, e.g. very soft, soft, OK, loud, very loud and too loud (Loudness Growth in 1/2-Octave Bands, LGOB scale) (Allen et al. 1990), and specific test procedures to measure the subjective perception of loudness. The highly subjective and non-linear nature of (loudness) sensation can lead to potentially significant interindividual and inter-scale differences, e.g. Elberling (1999); however the international standard ISO 16832 (2006), based on Brand and Hohmann’s Adaptive Categorical Loudness Scaling (ACALOS) (Brand and Hohmann 2002), has sought to increase reliability. The ‘Würzburger Hörfeld’ and ‘Oldenburger Hörfeld’ loudness scaling tests are well established within German clinics (Lehnhardt and Laszig 2009).
16.3.8 Dead-Region Testing
A cochlear dead region is an area of the cochlea in which the inner hair cells or auditory neurons are damaged to the extent that none are functional (Moore 2001). Given the tonotopic nature of the cochlea and the spread of acoustic sensation over the basilar membrane (see Sect. 1.4), stimulation of a cochlear dead region by using a signal of a frequency to which the non-functioning hair cells/neurons were tuned results in ‘off-frequency listening’, in which functional hair cells/neurons of a neighbouring region of the cochlea respond. Off-frequency listening is not detected on classic PTA, so specific tests are required. The threshold-equalising noise (TEN) test is one such test (Moore et al. 2000).
In the TEN test, a pure tone is played at the frequency of the suspected dead region, and a specifically designed TEN stimulus is played at 10 dB above the notional threshold of the suspected dead region. If the pure-tone threshold in the presence of TEN stimulus is <10 dB above the level of the TEN stimulus, then no dead region is judged to be present; if the pure-tone threshold is >10 dB above the level of the TEN stimulus, then a dead region is present. If a dead region is identified in a hearing aid user, the gain of the hearing aid could be adjusted to avoid stimulation at the affected frequencies, thus avoiding the distorted perceptual effects of off-frequency listening which can include impaired speech perception (Moore et al. 2000).
16.4 Paediatric Behavioural Audiometry (0–6 Years)
16.4.1 Introduction
Behavioural audiometry is an essential part of the diagnosis of hearing loss in very young children, even those younger than 6 months of age. Physiological measures (i.e. tympanometry, otoacoustic emissions, ABR and ASSR) should be complemented with behavioural measures to be able to determine the type and degree of hearing loss. The purpose of behavioural audiometry is to obtain air- and bone-conduction thresholds for the whole frequency range. Cross-checking of all measurements is needed before starting hearing aid fitting. This cross-check principle was described 40 years ago by Jerger and Hayes (1976) and is still up to date.
Behavioural audiometry needs well-experienced audiologists and is a time-consuming measurement that has to be done in optimal conditions. The test protocol will vary with the chronological age of the child. In case of retardation, it follows the developmental age of the child. Therefore you have to know the level of development and physical status (i.e. visual, motor abilities) before starting testing.
Testing can be done in a single or double soundproof booth. In a single soundproof booth, the examiner(s) and the child are in the same room. In a double soundproof booth, two examiners are needed, one with the child and one outside with the audiometer. The choice depends on personal preference. It may be useful to have both options.
To select the appropriate test method and setup, look into the developmental status of the child, especially in case of prematurity and mental or motor retardation.
Try to collect the results of all previous measurements. This information helps you to find the optimal starting level.
Always start with bone conduction if possible. It gives you the opportunity to use a vibrotactile stimulus (65 dBHL at 500 Hz or 45 dBHL at 250 Hz) (Cole and Flexer 2011), which you can use for conditioning the child when testing visual reinforcement audiometry (VRA) or conditioned play audiometry (CPA) (both outlined below). Even deaf children can feel these stimuli. At the same time, you obtain the result of the best inner ear, which you can compare with air-conduction results. Middle ear problems are very common in this age group. Moreover keep in mind that not every conductive hearing loss can be excluded with tympanometry.
Continue with air conduction. Always try to obtain ear-specific thresholds. Insert earphones are recommended for this population to avoid a collapsed ear. A higher inter-aural attenuation makes masking less necessary. Using the child’s earmould instead of the foam tip is even better.
Use warble tones, narrowband noise or fresh noise (a more frequency-specific stimulus than typical narrowband noise (which usually has a 1/3-octave range)) instead of pure tones. Sometimes it will be necessary to find other more interesting frequency-specific stimuli to get the child’s attention.
Be very flexible during the test. The proposed flowcharts can be a support for those who are not used to working in this way. It will be important to maximise the child’s attention span.
Sometimes it will be necessary to repeat the test. Some children need more time, are not in the best condition or ask for a different way of working. Tests may need to be conducted over several sessions in order to obtain appropriate diagnostic information.
16.4.2 Behavioural Observation Audiometry (BOA): 0–5 Months
Leading-In
Since universal hearing screening has been introduced in many countries, diagnosis will often happen before the age of 3 months. At this age behavioural observation audiometry can be an added value with the results of objective measurement, not only as an essential part of the cross-checking but also in counselling and supporting parents.
Although BOA is not generally accepted as an integral part of the paediatric audiometry test protocol, some paediatric audiologists provided evidence of BOA in this target group many years ago (e.g. Delaroche 2001; Delaroche et al. 2004, 2005; Madell and Flexer 2008).
BOA’s purpose is to obtain air- and bone-conduction data as close as possible to the hearing threshold for a broader frequency range of at least 500–4000 Hz.
This can only be performed in optimal conditions and by following a strict protocol.
Developmental Milestones
In the first 3 months of life, auditory reactions are unisensory and are based on reflexes. The most common reflexes are the cochleo-palpebral reflex, rudimentary head spin, changes in sucking, breathing, etc.
Between 3 and 5 months of age, unisensorial development becomes intersensory (i.e. the child becomes more able to combine input from more than one sensory system) (Piaget and Inhelder 1969). Most babies are already able to make a head turn to the sound, which can be reinforced by multimodal communication and personalised relationships. This has been described in the Delaroche protocol (Delaroche et al. 2011) as ‘congratulating with smiles, signs, caresses and speech’.
In the following paragraphs, test equipment and setup are explained:
Conditions
It is not the purpose of behavioural audiometry to search for strong reflexes as a reaction to loud sounds, but to look for minimal changes in behaviour as a reaction to soft sounds. These reactions are called the minimal response levels (MRLs). They can only be obtained in optimal conditions, as in a light sleep or while feeding or sucking (awake but peaceful). If the child is in a deep sleep, it will not respond, so its sleep state should be confirmed, by touching the eyelashes (the eyelids will move slightly when the child is not in a deep sleep).
When the child is hungry or does not feel comfortable, it may be too distracted to respond to the quietest sounds it can hear.
Testing has to be done in a soundproof booth with minimal distraction. Minimise tactile or visual impulses; no people other than the parents are allowed. When the mother is feeding, ideally the test can start from the moment the sucking becomes effortless and regular.
Transducers
It is preferable to use the vibrator for bone conduction on an adapted diadem or softband. Air conduction with insert earphones gives ear-specific information with a great inter-aural difference. Therefore soundfield is not recommended. Furthermore, soundfield stimulation is less reactive (i.e. clear responses are more difficult to observe) and limited in loudness for finding the minimal response level (MRL) at this age.

BOA with vibrator (left) and insert earphones with foam tip (right). Photo with kind permission of the parents
Stimuli
To obtain a proper response of the child, use warble tone, fresh noise or narrowband noise. At this age pure tones are not attractive enough. It may be necessary to change the nature of the stimulus while testing.
Procedure
To obtain the minimal response level of the child, it is necessary to start below the expected threshold. A proper reaction can only be obtained when reaching the minimal response level for the first time for each frequency.
Start with bone conduction. Place the vibrator on the mastoid (Fig. 16.9 left), and observe the child’s behaviour for a few minutes before presenting any sound in order to know the behaviour without auditory stimulation. If a bone-conduction hearing loss can be expected, start at the MRL of a normal-hearing child at this age, which will be 30–40 dBHL (Delaroche et al. 2011).

Flowchart BOA bone conduction
Continue with air conduction using insert earphones (Fig. 16.9 right) on the basis of information from objective measurements, if available, or start from the bone-conduction thresholds you have just measured.
If click ABR testing was performed, start at 2 kHz, 20 dB below the ABR threshold level, and choose the better ear. Preferably it is necessary to obtain ear-specific information as soon as possible. Eventually binaural stimulation by insert phones can be used to have an initial idea about the type of hearing loss and the responsiveness of the better inner ear.

Flowchart BOA air conduction
Characteristics of Responses
Most common responses are opening eyes, moving eyelashes and starting or stopping sucking.
The stimulation and response delay are different for each child. Make sure that this delay remains the same for the same child.
The number of observable responses is limited because of the problem of habituation. With BOA it is not possible to confirm each response as with conditioned behavioural audiometry with older children.
Minimal Response Level (MRL)
In threshold estimation with BOA, a clear distinction has to be made between neonates with normal hearing and neonates with a sensorineural hearing loss. While normal-hearing babies have higher response levels, hearing-impaired babies with a loss of 50 dBHL or more react closer to their real hearing threshold. These findings are well described by Delaroche (Delaroche et al. 2011). One possible cause is the phenomenon of recruitment, which results in faster loudness scaling in case of sensorineural hearing loss. Another cause is the effect of surprise, which makes babies with a hearing loss respond faster and clearer to a sound that is right on or just above their threshold level.
16.4.3 Visual Reinforcement Audiometry (VRA): 5–24 Months
Leading-In
From the age of 5–6 months, most children can be conditioned to make a head turn response towards an auditory stimulus (Northern and Downs 2001). This will be the start of visual reinforcement audiometry (VRA) and conditioned orientation reflex audiometry (COR). In both cases a child’s turning response is rewarded with a visual incentive. In VRA we use one reward. COR, using two rewards, requires adequate ability to localise a sound source. In this chapter we only discuss VRA.
Developmental Milestones
In a normal developing child of 5–6 months of age, the intersensory phase is expanding rapidly. Every time an audible sound is presented, we use the natural strong interest in coloured and illuminated animation to condition the child to look at a visual reward as reinforcer.
This will not be possible in children with intersensory problems such as autism spectrum disorders.
Conditions
VRA can be performed in a single or double soundproof booth where the lights can be dimmed. The younger the child, the more we prefer to work in a single booth. The test room should not be cluttered because, as in BOA testing, it is important to have fewer potential distractions. The examiner and test assistant keep the child attentive by being relatively quiet and not interfering while presenting the test stimuli. The presence of one parent, without interfering with the process of testing, can keep the child calm. The child needs to be seated in such a way that a head turn can easily be carried out in the direction of the visual reward.
Transducers
All transducers can be used.
Stimuli
Do not use pure tones but more reactive stimuli such as warble tone, frequency-specific fresh noise or narrowband noise. With hard-to-test children, it can be necessary to change the nature of sound during the test or even use familiar sounds (i.e. barking dog, melody, crying baby, etc.).
Animation and Distractors
A variety of visual animations and moving toys can be used as reinforcement. Moore et al. (1975) investigated in the effect of different kinds of visual animation. They demonstrated that auditory localisation behaviour of infants is influenced by the type of reinforcement. At the youngest age, three-dimensional animations are preferable, such as a flashing lamp and brightly illuminated mechanical toys (i.e. clown playing drums, dancing bear).
These toys have to be enclosed in a cloudy Lucite box so the child cannot easily see the animation until it is turned on. Older children, who are no longer interested in moving toys, can be reinforced with two-dimensional reinforcements such as a video or animation on a screen.
The test assistant keeps the child attentive, often by quietly playing with (interesting, but not fascinating) toys in front of the child. The tester and assistant should be relatively quiet, making sure not to interfere while the test stimuli are being presented. If the child finds the visual reinforcement very interesting, they might keep ‘checking’ (i.e. looking at the reinforcer even when there is no sound stimulus). In this case, the test assistant can help to distract the child from the visual animation, perhaps by temporarily increasing their level of play.
Procedure
The test procedure consists of two phases: the conditioning and the testing. It is recommended to start with bone conduction on a vibrotactile level (500 Hz at 65 dBHL or 250 Hz at 45 dBHL) in order to condition the child to respond by consistently turning the head to the auditory stimulus and to determine the bone-conduction thresholds.
Continue with insert earphones or headphones to determine ear-specific and air-conduction thresholds.

Flowchart VRA starting with bone conduction
In case of uncooperative behaviour when the vibrator or headphones cannot be used, it is better to work in free field in the first contact. In a second stage, ear-specific information should be obtained.
Free-field measurements are also useful for looking for the functional gain with hearing aids or cochlear implants. Soundfield can also be necessary to give the parents insight into the level of hearing impairment.
In cases where any contact with a vibrator or headphones is refused, it will be necessary to start in a soundfield setting.

Flowchart VRA starting in free field
Minimal Response Level
Normal and hearing-impaired children can respond close to their hearing threshold at this age. Madell and Flexer (2008) refer to several studies that demonstrate that infants with normal hearing respond to sound no higher than 15–20 dBHL and children at the age of 1 respond at an adult level. But it is still advisable to consider MRL because of the variability between the children. The responses in normal-hearing infants should not be higher than 20 dBHL for all frequencies.
16.4.4 Conditioned Play Audiometry (CPA): 2–5 Years
Leading-In
CPA demands the child’s active cooperation by the need to perform an action after hearing an audible sound. This is a conditioned response. The ‘listen and drop’ task is the simplest level. The child may drop a block in a box or put a ring on a ring bar. The ‘button-pushing response’ to activate a rewarding animation requires a higher cognitive level, so is reserved for older children. Nowadays an interesting PowerPoint programme can be used on a laptop that is controlled by a remote mouse operated by the audiologist. Madell (Madell and Flexer 2008) called this special technique a ‘computer-assisted reinforcement’ procedure.
Developmental Level
Most normally developing children are near 2 or 2.5 years of age before they are able to participate in play audiometry. Children with hearing loss, who frequently have hearing tests, are able to learn the ‘listen and drop’ task earlier.
Infants at this age are not always cooperative. The paedaudiologist will have to be creative and flexible to obtain enough testing information. Good paedaudiologists should be able to swap quickly between a repertoire of games with different levels of engagement aimed at children with different attention and cooperation. More complex games can be reserved for older or more mature children. Similarly, different types and levels of reward are appropriate. Even CPA may need to be conducted over several sessions in order to obtain appropriate diagnostic information.
Conditions
If one audiologist is performing the test, it will be necessary to work in a single soundproof booth. The test room should be orderly to avoid distraction. Parents can be involved during the training.
Transducer
Air- and bone-conduction transducers can be used in order to determine the type and the degree of hearing loss.
Stimuli
Use warble, fresh noise, speech sounds or familiar sounds.
Setup
The infant can sit at a children’s table or in a highchair.

An example of CPA test setup
Material
A variety of toys is needed, according to the interest and developmental age of the child, to be able to maintain the child’s attention.
The ‘listen and drop’ task is the simplest level.
When using ‘computer-assisted reinforcement’, the child uses a push button that is not actually connected to the computer. The button-pushing responses of the child will only be rewarded if there was a preceding stimulus. Even a simple PowerPoint presentation with popular characters can maintain the child’s interest.
Procedure
The test procedure consists of two phases: conditioning and testing. To start the conditioning session, it is important to use a test stimulus that the child is expected to hear. In case of doubt, bone conduction on a vibrotactile level (e.g. 500 Hz, 65 dBHL) can be used.
Conditioning
Demonstrate the task: the audiologist and the child hold a toy to the ear to highlight the listening task. When sound (for at least 5 s) is presented, the audiologist clearly shows the presence of sound by dropping the toy. The child has to do the same. Parents can be involved as model in this process.
After a few presentations, the child should hold the toy to the ear alone. When the sound is presented, the child must drop the toy on his/her own. Encouragement is important in this stage.
When the child is able to do the task without assistance, the test can start.
Remark: conditioning should be repeated at any point during the test if the child’s responses seem to indicate that it is no longer cooperating.
Testing
Rapidly decrease the level of the sound that you used for the conditioning task and continue with the ‘5-up-10-down method’ (Hughson-Westlake method) to find the threshold.
Change frequency and start approximately 20 dBHL above the measured response.
If the child gets bored, the toys may need to be changed.
When testing with insert earphones or headphones and the attention span seems to be very short, it may be necessary to change to the left or right ear after each frequency.
Response Behaviour
At this age the child may respond at an adult level, so the term ‘minimal response level’ is no longer appropriate.
16.4.5 Hard-to-Test Populations
Testing children with special needs follows the same principles as testing children without additional problems. Some adaptations and specific knowledge may be necessary.
For children with an intellectual disability, the response can be significantly delayed because of the time needed to recognise the sound. Therefore it can be necessary to hold the stimulus for a longer time.
For children with motor problems, modifications of the child’s position may be necessary in order to enable clearly visible responses (i.e. when a child does not have a good trunk control, an adapted chair is needed).
Children with visual impairment can benefit from dimming the room light in order to make the reinforcers more visible.
For blind children, tested with VRA, air-puff reinforcement can be used instead of a visible reinforcer.
A special technique for this population is TROCA (tangible reinforced operant conditioning audiometry), where food or tokens are used as reinforcers instead of flashing lights or mechanical toys.
With disabled children, it will be important to keep the carers involved in behavioural tests to be able to choose the right procedure and material and interpret the child’s responses.
16.5 Assessment of Tinnitus: A Specialist Otorhinolaryngology Perspective
16.5.1 Introduction
Tinnitus evaluation may differ according to the time of onset (acute vs chronic), classification (objective vs subjective) and accompanying symptoms; however the following steps are important and performed for almost all cases.
16.5.2 Medical History
An adequate, detailed medical history should gather information about possible causes (such as hearing loss, use of ototoxic drugs including salicylates, quinine and aminoglycosides), otological diseases (sensation of aural fullness, sporadic rotatory vertigo lasting hours or temporarily reduced hearing may give a hint towards Meniére’s disease), non-otological diseases (head trauma, noise exposure and the presence of metabolic diseases symptoms) and the tinnitus percept: date of onset (sudden or gradual), localisation (unilateral, bilateral, in the head), subjective loudness, type of sound (ringing, buzzing, hissing, pulsating), temporal aspects (continuous, intermittent), anything that improves or worsens the tinnitus and impact upon the patient (depression, anxiety, concentration, disrupted sleep). Questionnaires to evaluate the psychological influence of the tinnitus (e.g. the Tinnitus Handicap Inventory (Newman et al. 1996)) are useful.
16.5.3 Clinical Examination
Ear, nose, throat and neck examination is essential for each patient in order to investigate possible objective causes. During this assessment, a temporomandibular joint examination, evaluation of the cranial nerves as well as auscultation of the neck and pressure on the ipsilateral internal jugular vein are very important. If the tinnitus percept is reduced during these manoeuvres, venous aetiology may be indicated (Baguley et al. 2013). In a very small percentage of tinnitus patients, the vascular aetiology may be due to a serious underlying disease such as carotid artery dissection or aneurysm.
16.5.4 Audiological Examination
Audiological Tests
Given that subjective tinnitus results primarily from dysfunction within the auditory system, an age-appropriate assessment of hearing thresholds and middle ear status may be worthwhile, both to investigate the aetiology and to guide later treatment. Such audiological investigation may include pure-tone audiometry, speech discrimination testing and tympanometry; however, uncomfortable loudness-level measurements and acoustic reflexes should be treated with caution as high-level sound may exacerbate the symptoms. Some evidence suggests that a causative auditory system change may not be detected by standard audiological tests (e.g. Fabijańska et al. 2012); therefore testing in an extended high-frequency range and the use of otoacoustic emissions may be useful. Electrocochleography (e.g. to diagnose Meniére’s disease), auditory brainstem response audiometry (e.g. as one diagnostic element in detecting a cerebellopontine tumour) and videonystagmography (to find vestibular system dysfunction) (Jozefowicz-Korczynska et al. 2005) may be performed if needed.
Psychophysical and Other Tinnitus Assessment Procedures
Audiological tests, such as pitch and loudness matching, are often performed in order to define the parameters of the tinnitus percept. Note that these tests can only be carried out when the patient’s tinnitus is audible to them during testing.
The basic procedure of pitch matching involves presenting low-level pure tones or narrowband noise of various frequencies and asking the patient to indicate when the pitch is similar to that of their tinnitus. As tinnitus may be a spectrum of sounds, spectral matching is recommendable (Henry 2016).
Loudness matching involves increasing the level of the pitch-matched signal (usually in 1 dB steps) from threshold until the patient indicates that the loudness of the signal and tinnitus are equal. Both procedures should be performed separately for each affected ear if possible.
It is important that the patient understands the difference between pitch and loudness during these matching procedures and that they are informed that slightly inexact responses will not hinder their treatment. The matched frequency and level are often indicated by a ‘T’ sign on the audiogram.
Minimum masking level (MML) procedures are sometimes carried out with the aim of determining the patient’s ability to suppress the tinnitus signal in the presence of competing noise. The level of white noise (a random broadband signal that includes energy at all audible frequencies) is gradually increased until the patient reports any change in the tinnitus (such as it becoming louder, softer or entirely suppressed). Tinnitus matching and MML testing are, however, much less simple than one might expect. For example, many different matching procedures have been tested since the 1940s, and this area is rife with methodological difficulties (see Henry and Meikle 2000).
Tinnitus can be inhibited for up to approx. 1 min in some patients following some types of auditory stimulation. This is known as residual inhibition. According to Henry (2016), studies should be performed to develop optimal stimuli for extending this effect as basis for a new therapeutic approach.
Given that parameters of the tinnitus percept, such as loudness, pitch and quality, do not correlate with the perceived severity of tinnitus (Meikle et al. 1984), tinnitus severity may be better assessed from questionnaires (such as the Tinnitus Handicap Inventory) which address the patient’s perception of their tinnitus and its impact on their lives, rather than psychophysical investigation.
The Tinnitus Handicap Inventory (THI) (Newman et al. 1996) contains 25 self-reporting closed questions related to personal and social situations as well as feelings about tinnitus, designed to assess the severity of the patient’s tinnitus (slight, mild, moderate, severe or catastrophic). Other tinnitus questionnaires exist, such as the Iowa Tinnitus Handicap Questionnaire (Kuk et al. 1990), the Tinnitus Reaction Questionnaire (Wilson et al. 1991) and the Tinnitus Functional Index (TFI) (Henry et al. 2014). But as to our knowledge, there are no standardised questionnaires for children (BSA 2014).
In patients with hearing impairment, the ten-item Tinnitus and Hearing Survey (THS) (Henry et al. 2015) separates complaints caused by hearing loss from self-perceived problems induced by tinnitus by two specific subscales.
Given the strong connection between tinnitus and anxiety/depression, some clinics also use tools such as the Hospital Anxiety and Depression Scale (Zigmond and Snaith 1983) to assess the psychological impact on the patient.
16.5.5 Other Investigations
If, e.g. chronic otitis media or otosclerosis is suspected, high-resolution computed tomography may be useful. Magnetic resonance imaging is usually the preferred method in patients with asymmetric hearing loss or asymmetric tinnitus to rule out a cerebellopontine angle tumour, such as vestibular schwannoma. Magnetic resonance angiography or venography may be necessary in cases with suspected cerebral ischemia, dural sinus thrombosis or neoplastic lesion (Sismanis 2011). Doppler ultrasonography may be useful for exploring the vascular structures in the neck. Blood tests for haemodynamic and metabolic diseases are also important.
16.6 Assessment of Tinnitus in Children and Teenagers: A Specialist Paediatric Audiology and Hearing Therapy Perspective1
Prevalence figures for tinnitus in children in studies vary from 12 to 36% for normally hearing children and go up to 66% for children with known hearing loss (Shetye and Kennedy 2010). The impact of tinnitus in children is in many ways similar to that in adults, affecting sleep, concentration and listening skills (Kentish et al. 2000).
History
Description of tinnitus sounds: children can use very descriptive language to describe what they hear such as ‘buzzing bees’, ‘swishing’ and ‘like a train’. Younger children may find it helpful to draw a picture of their tinnitus. Where possible details of onset, duration and how often it is heard are useful.
Impact of tinnitus: there are currently no standardised tinnitus questionnaires for children. The impact and concerns of both the parents and the child should be investigated. Finding out about the effects of the tinnitus on school and home life is important. Standardised measures of anxiety and depression in children do exist and these can be helpful.
Hearing difficulties and other audiovestibular symptoms: it is important to rule out any physiological reason for the tinnitus. Asking about their history of otitis media with effusion is also useful.
Medical and neurological factors: any history of head trauma or noise trauma should be noted. Other general medical problems may also be relevant, for example, migraine can be associated with auditory sensitivity and tinnitus.
Factors affecting their tinnitus: some children or families may have noticed factors that make the tinnitus better or worse, such as tiredness, school situations or stress. It is very important to find out about the child’s whole life and potential areas of stress for him or her, such as bereavement or family breakdowns.
Clinical Assessment
Children with tinnitus can find audiometry particularly stressful; they may have a history of being difficult to assess or have variable results on previous tests. The testing environment should be child-friendly and the children given time to do the test at their own pace. It is important to establish accurate, ear-specific thresholds by using age-appropriate testing techniques. Observing the child closely throughout the test allows any signs of anxiety to be seen and reassurance given. Otoscopy and middle ear admittance measurements are always needed to rule out any pathology that requires further medical treatment. At the moment, loudness discomfort measures or any tinnitus-matching tests are not recommended, as there is very limited evidence for either the diagnostic or therapeutic benefit of such tests (see Henry and Meikle 2000 for a review of such psychoacoustic measures).
16.7 Speech Audiometry at the Phoneme and Word Level
16.7.1 Introduction
The assessment of auditory processing and recognition of speech in infants and children is important and complex: the child’s performance in a speech recognition test does not only depend on the auditory skills themselves but also on factors such as language, cognition, articulation and speech, visual cognition, attention and concentration. Typically, these factors are dynamic and changing over time.
Structured overview of auditory tests at four functional levels
Level | Phonemes/syllables | Words |
---|---|---|
Detection | • Ling 6 sound test • Ling sounds-based ‘PTA’ (pure-tone audiometry) | |
Discrimination | • A§E (auditory speech sounds evaluation; Govaerts et al. 2006) • H-LAD (Heidelberger Lautdifferenzierungstest; Brunner et al. 1998) • WADT (Wepman auditory discrimination test; Ross 1979) | |
Identification/recognition | Pictures tasks • MTS (Monosyllable-Trochee-Spondee test; Erber and Alencewicz 1976) • AAST (adaptive auditory speech test; Coninx 2005) | |
Comprehension |
16.7.2 Phoneme/Syllable Level

The Ling Audiogram shows the detection thresholds of the six Ling sounds. In this example, the detection of the two fricatives is most likely not good enough for them to be heard in normal running speech
The Ling test uses isolated phonemes. A phoneme-based test, supplying useful information on the basic listening level, can also be based on a syllable form or even by using words.
As an example, in the A§E test (Govaerts et al. 2006), syllables are used to assess detection and discrimination skills in the child. For both skills, an operant conditioning protocol is used: visual reinforcement audiometry (VRA). The response of the child to the detection of a stimulus or the discrimination of a stimulus change has to be reliably observed by using paradigms that are adapted to the developmental level of the child. For the difficult-to-test younger children, VRA (Coninx and Moore 1997) might be the only successful procedure; for older children the usual techniques of play audiometry can be used. With the VRA paradigm, not only phoneme detection but also phoneme discrimination can be investigated. In case of discrimination testing, the visual reinforcer is presented in relation to a sudden change in a sequence of ‘stable’ phonemes or syllables (e.g. da-da-da…) to another sequence of ‘stable’ stimuli (e.g. ta-ta-ta…). A response of the child to the sudden phoneme change is visually reinforced.
For older children, easier and faster ‘same-different’-based tasks can be used instead. The stimuli would be two isolated phonemes (/s/-/f/ or /a/-/e/), two consonant-vowel syllables (/sa/-/fa/ or /ba/-/be/) or a minimal word pair, differing only in the same phoneme contrast (seat-feet or ball-bell). Various responses from the child can be used: verbal feedback (‘same’ versus ‘different’) or pointing to a picture representing same or different. Isolated phonemes or consonant-vowel syllables are considered to be better than words, because the test result for word pairs might depend on the vocabulary of the child. This is caused by lexical neighbourhood processes (Janse and Newman 2013). Examples of word-pair tests are H-LAD (German, Brunner et al. 1998) and WADT (English, Ross 1979).
16.7.3 Word Level
The most frequently used form of speech audiometry in children is based on words. Children develop skills to identify or recognise words earlier than full sentences, as well as earlier than isolated phonemes (a subskill of phonological awareness). For test applications, the words have to be chosen carefully in order to be within the vocabulary of children in the target group age. The words can be presented as stimuli in a quiet or noisy environment. The response of the child can be different: repeating the spoken word or pointing (or comparable action) to a picture or object that represents the spoken word.

The six spondee words of the AAST UK version. The red dot in the centre cell is a visual prompt during stimulus presentation
Open-set tests are similar to many tests used for adult listeners. This choice has to be carefully considered, as interference with the speech and articulation skills and the vocabulary of the child will interfere with the auditory skills as such. A gold standard for an open-set test—for English—is the PB-K test (Haskins 1949), which uses a phonetically balanced word list for children in kindergarten.
Closed tests as described above are used to assess a word recognition score at a selected fixed intensity, at 65 dB SPL or at the individually different most comfortable level (MC).
In order to find a speech recognition threshold (SRT), the test has to be repeated several times; alternatively, adaptive paradigms have to be used. A typical example is the adaptive auditory speech test (AAST) for children aged 3–4 years and above (Coninx 2005). Only six spondee words are used, requiring little vocabulary and minimised influence of working memory and visual cognition when the child is searching for the correct picture to click. When using touchscreen technology, even young children 3–4 years old can do the test and are highly motivated and concentrated. After typically 1–1.5 min, the SRT measurement is completed. The result of a test has to be interpreted by taking the age-dependent normal values into account. An important advantage of AAST is the multi-lingual applicability with nearly language-independent normal values.
For tests in noise, steady-state noise can be used (more likely related to cochlear dysfunctions) (Smits et al. 2013) or fluctuating noises, such as the International Female Fluctuating Masker (IFFM, Holube et al. 2011). The thresholds in IFFM are more likely to be related to retrocochlear dysfunctions, i.e. auditory processing disorders.
Future development in speech audiometry for children will increasingly include modern technology with a touchscreen. This not only increases the level of motivation but also opens options for remote screening and testing.
16.8 Auditory Processing Tests
16.8.1 Diagnosis
There is no universally agreed diagnostic procedure for auditory processing disorder (APD). Diagnostic recommendations have been made by the American Speech-Language-Hearing Association (ASHA 2005a, b) and by the American Academy of Audiology (AAA 2010) on the basis of several distinct forms of impaired auditory processing outlined below. These recommendations are a quasi-international standard, but in practice the recommendations are adhered to only loosely in the USA (Emanuel et al. 2011) and even less so in other countries (Hind et al. 2011). In Germany, the diagnosis of ‘auditory processing and perception disorder’ (APPD) (German: auditive Verarbeitungs- und Wahrnehmungsstörung (AVWS)) includes auditory-specific cognitive impairment as a designated form of APPD (GSPP 2014), but only if that impairment is judged not to be described by other, more widely accepted terms (e.g. attention deficit disorder, language impairment). Some countries have issued practice guidelines in recent years, rather than stipulating comprehensive diagnostic measures and criteria. For example, Australian Hearing has implemented standard procedures for the diagnosis, assessment and management of some aspects of APD (Hearing 2013), and the British Society of Audiology (BSA APD SIG 2011a, 2018) has provided an evidence-based commentary to guide practice.
An attempt to reach a consensus on APD definition, diagnosis and management was the principal aim of two ASHA Technical Reports (ASHA 1996, 2005b). Auditory processing was determined to be the neural mechanisms underlying auditory discrimination and pattern recognition; temporal, spatial and binaural aspects of hearing; and perception of competing and degraded auditory signals. APD was defined as a disorder of one or more of these processes, which were noted to be necessary for both non-verbal and verbal perception. Impairments should be diagnosed by using a variety of non-standard indices (e.g. case history, observation of behaviour, questionnaires) and standardised auditory measures that use both non-verbal and verbal stimuli. Electrophysiological testing (e.g. auditory brainstem response, cortical event-related potentials) was also suggested, but details were not provided. Performance of at least two standard deviations (s.d.) below the (standardised) mean on two auditory processing tests, or 3 s.d. on one test, is ‘generally required for diagnosis’. However, both the ASHA Reports and the AAA (2010) Guidelines suggest that the number and specific type of tests used should depend on individual cases. There is no specification of the number of tests that are to be used, and the criteria, like those proposed by other groups, are arbitrary. Statistically, the more tests performed, the more likely an individual will fail two of them. Wilson and Arnott (2013) showed in a large sample of children (n = 150) that diagnosis rates of APD can range from 7 to 93% depending upon which criteria are applied, even using the same test battery.
16.8.2 Differential Diagnosis
Because APD typically presents as problems involving speech perception, most guidelines recommend verbal (speech-based) testing. For example, the commonly used SCAN test battery (Keith 2009) has four core tests that all use verbal stimuli. Verbal stimuli used in APD diagnosis vary from nonmeaningful phonemes (e.g. ‘da’) or vowel-consonant-vowel (VCV) syllables (e.g. ‘ata’) through commonly used short words (e.g. ‘dog’) to longer words and whole sentences. These stimuli all involve a lesser or greater degree of memory and linguistic processing that strong neurological evidence suggests occurs beyond the central auditory nervous system, primarily in the anterior temporal and frontal brain lobes. An ongoing debate concerns whether APD should be diagnosed when only ‘unimodal’ auditory deficits (i.e. deficits specific to hearing) are found (Cacace and McFarland 2013).
To segregate distinctly auditory deficits, non-verbal testing for APD diagnosis is also recommended. Non-verbal tests attempt to isolate those aspects of auditory processing, as listed above (discrimination, pattern recognition, etc.), that are the building blocks of everyday listening. However, there is scant evidence that either these tests or those involving verbal stimuli are very good predictors of the listening problems that people being assessed for APD typically report (Moore et al. 2010; Watson and Kidd 2009). The most common of those problems are, for children, academic difficulties and attentive listening to speech, especially in challenging environments (AAA 2010; Campbell et al. 2012). In addition, listening to both speech in everyday life, and to verbal or non-verbal tests in the clinic, necessarily accesses cognitive resources that are mostly ‘supra-modal’ (not specific to hearing). These resources include attention, memory, fluid intelligence, motivation and emotion. Professional guidelines urge the clinician to ensure that the person being tested is attentive, but clinicians cannot adequately control or measure cognitive influences on perception with current test procedures.
16.8.3 Occurrence of APD (not ADP!) with Other Learning Problems
Only a small proportion of children referred to audiology clinics are assessed for APD, and only a fraction of them receive a diagnosis of APD (Hind et al. 2011; Moore and Hunter 2013). However, around 20% of all children have speech, language, attention, behaviour, reading or intellectual difficulties. A substantial proportion of them perform poorly on tests of auditory processing (Amitay et al. 2002; Ferguson et al. 2011; Miller and Wagstaff 2011; Sharma et al. 2009) or have behaviour that overlaps extensively with that seen in children with APD (Chermak et al. 1999, 2002). Conversely, most children diagnosed with APD have multiple difficulties. Do those children all have APD? The relation between perception and cognition has been debated for well over 100 years, i.e. whether hearing or vision determines intelligence or vice versa (Spearman 1904). Surprisingly, this is still a current debate, for example, in the extensive literature on language and reading difficulties (Ziegler et al. 2005, 2009). The problem faced by hearing specialists is whether to diagnose APD, recognising the non-specific nature of the diagnosis, or to refer a child for a more broadly based assessment. In either case, identifying individual difficulties is the first step towards appropriate treatment.
16.8.4 How Should APD Be Diagnosed?

Draft hierarchical scheme for the diagnosis and rehabilitation of APD by using specific assessments that incorporate a problem-orientated approach and differential and adaptive testing (Moore 2012; Dillon et al. 2012). The scheme includes conventional (audiometry) and research procedures. A validated questionnaire (e.g. ECLiPS (Barry and Moore 2014, Barry et al. 2015)) assesses everyday listening skills which, if deficient, lead to further objective assessments. Electrophysiological testing (auditory brainstem and frequency-following response) determines ear and brainstem function objectively. Speech-in-noise testing (e.g. LiSN-S) and ‘forced attention’ dichotic CV syllables (Hugdahl et al. 2009) differentiate ‘bottom-up’ and ‘top-down’ processing difficulties
16.8.5 Current Situation and Way Forward
Several groups around the world have now issued APD statements, guidelines or white papers, including the American Speech-Language-Hearing Association (ASHA 2005a, b), the American Academy of Audiology (AAA 2010), the British Society of Audiology (BSA APD SIG 2011a, b, 2018; Moore et al. 2013), the Canadian Interorganizational Steering Group for Speech-Language Pathology and Audiology (2012), the German Society of Phoniatrics and Paediatric Audiology (Nickisch et al. 2015), the Australian National Acoustics Laboratory (NAL 2015), the Dutch Position Statement (De Wit et al. 2017) and more recently a group in Europe which has published its perspective on APD (Iliadou et al. 2017). As outlined at the beginning of this section, there is no universally agreed diagnostic procedure for APD, but all of the above documents contribute to international debate and better understanding. High-calibre research, alongside international and interdisciplinary dialogue, is imperative for informing future evidence-based practice. The APD MESHGuide, a new evidence based online resource, offers practical guidance (Campbell et at. 2019).
Remediation is considered in Sect. 18.14.
16.9 Acoustic Immittance Measurements
16.9.1 Introduction
The ability of the tympanic membrane and ossicular chain to transfer sound waves from the outer ear canal through the middle ear is known by a variety of related terms that have specific technical differences but are often used interchangeably in common practice: admittance, compliance, conductance, immittance and susceptance. The opposite (i.e. the ability of the mechanism to resist the transfer of sound) is typically known as impedance or reflectance.
The stiffer the tympanic membrane, the smaller the amount of sound energy admitted through it (low admittance) and the greater the amount of sound energy reflected by it (high impedance). Sound energy is optimally transferred across the tympanic membrane when the air pressure within the middle ear is equal to that in the outer ear canal, which is typically an atmospheric pressure of 0 daPa (decapascals). Most common disorders of the middle ear, such as middle ear effusion or Eustachian tube dysfunction, affect the air pressure within it, thus stiffening the tympanic membrane.
Tympanometry measures admittance at various external air pressures in order to assess the presence of middle ear disorders. Acoustic reflex testing assesses the basic function of cochlear and retrocochlear structures involved in the acoustic reflex arc by measuring the lowest sound level that triggers contraction of the stapedius muscle (a response that stiffens the ossicular chain and tympanic membrane, resulting in measurably reduced admittance).
While tympanometry and acoustic reflex testing are not direct measures of hearing sensitivity, tympanometry especially plays a crucial role in audiological diagnostics, providing further information in cases of conductive hearing loss and functioning as a cross-check to support the findings of other tests. Other acoustic immittance tests exist (e.g. acoustic reflectometry and tests of Eustachian tube function) but are not common in clinical audiology and are not discussed in this article.
The patient does not need to respond actively during acoustic immittance tests and should ideally be still and quiet.

Block diagram showing key components of an audiometer, the probe and ear tip and an air pump and manometer, to change and monitor air pressure in the outer ear canal; the probe tone is generated by the oscillator and conducted to the ear canal via the earphone; the microphone measures the level (dB SPL) of the probe tone in the opening of the outer ear canal and admittance is calculated. Image from Benton et al. (2004), with kind permission from Wiley © (2004)
International standards for the calibration of tympanometers (ISO 60645-5 2005) must be adhered to.
16.9.2 Tympanometry
In tympanometry, the air pressure in the outer ear canal is varied over the course of a few seconds, usually from a positive pressure of approximately 200 daPa to a negative pressure of approximately −400 daPa at a rate of 50 daPa/s (e.g. British Society of Audiology (BSA) 2013). The rate and direction of pressure change can be varied in many tympanometers, but variations in these parameters play little role in typical clinical testing. During this pressure change, the probe tone (usually 226 Hz) is played constantly into the outer ear canal, and the sound that is reflected from the tympanic membrane is detected by the microphone.
Results and Interpretation

Classic tympanogram shapes: (a) normal, (b) hypermobile, (c) reduced compliance, (d) flat, (e) negative
Tympanogram interpretation when using a 226 Hz probe tone
Result | Interpretation | Description | |||
---|---|---|---|---|---|
Peak compliance (mL, mmho/L or cm3) | Peak pressure (daPa) | Ear canal volume (cm3) | Commonly used term | Audiogram type (Jerger 1970) | |
Adults: 0.3–1.6 Children (6m–6y): ≥0.2 (BSA 2013) | Adults: −50 to +50 Children: −200 to +50 (BSA 2013) | Adults: 0.6–1.5 Children: 0.4–1.0 (BSA 2013) | Middle ear function within the normal range | Normal | A |
Higher than normal range | Normal range | Normal range | Hypermobile tympanic membrane or ossicular discontinuity | Hypermobile | AD |
Lower than normal range | Normal range | Normal range | Reduced tympanic membrane mobility or ossicular fixation | Reduced compliance | AS |
Flat | Flat | Normal range | Middle ear effusion | Flat | B |
Normal range | Higher than normal range | Normal range | Possible acute middle ear pathology | Positive | – |
Normal range | Lower than normal range | Normal range | Eustachian tube dysfunction | Negative | C |
Flat | Flat | Higher than normal range | Grommet in situ and patent or perforated tympanic membrane | Large ECV | – |
Flat | Flat | Lower than normal range | Occluding wax or incorrectly placed probe | – | – |
A classification system is sometimes used when reporting tympanometric results (e.g. Jerger 1970, Table 16.3), but it is preferable to report the actual values obtained. Some commonly used terms for reporting audiogram shape are also indicated in Table 16.3 (Fig. 16.20a–e showing common results of tympanometry).
Many tympanometers also show gradient, which essentially reports the sharpness or roundedness of the peak, but different manufacturers use different algorithms to calculate this (BSA 2013) so interpretation is not always clear. Gradient does not play an important role in typical clinical interpretation guidelines.
Contraindications
Tympanometry should be preceded by otoscopy or microscopy in order to check for occluding wax (which should be removed before testing), inflammation or infection of the outer ear (which would contraindicate the test owing to the risk of pain or cross infection) and to provide a visual check of the status of the tympanic membrane. Previous tympanic membrane surgery may contraindicate tympanometry because of the risk of the change in air pressure damaging the TM.
The change in air pressure should not be painful or uncomfortable for the patient in the absence of infection or inflammation. Subjects with pressure-related vertigo, such as that resulting from a perilymph fistula in the vestibular system, may feel some dizziness, but other patients typically do not.
Tympanometry can be performed on compliant patients of any age, with a small but crucial change in probe tone frequency for younger patients (see below).
Probe Tone Frequency
For children younger than 6 months corrected age (BSA 2013) or with an ear canal volume less than 0.9 mL (Limberger et al. 2007), a probe tone frequency of 1000 Hz provides a better measure than the typical 226 Hz. This is because the walls of the infant ear canal are more flexible than those of older children and adults, and a large contribution to the tympanometry results can come from the compliance of the outer ear canal itself, rather than just the middle ear. This effect is reduced when using a higher-frequency probe tone.

Interpretation of tympanometry when using a 1 kHz probe tone. Image adapted with kind permission of the British Society of Audiology (2013)
Wideband Tympanometry

A hypothetical 3D tympanogram on which frequency (in red), pressure (in orange) and absorbance (in blue) are shown. Image copyright and with kind permission of Interacoustics
16.9.3 Acoustic Reflex Testing

Schematic diagram of the acoustic reflex arc. ME middle ear; SM stapedius muscle; VIII vestibulocochlear (8th) nerve; SOC superior olivary complex; VII MN medial nucleus of 7th nerve; VII facial (7th) nerve
The acoustic reflex threshold (ART) is the lowest signal level (dB SPL) from which the acoustic reflex is triggered. This is usually performed at the pressure level of peak compliance for the individual subject, in order that the response obtained is as large as possible. Various stimuli can be used, typically pure tones (500, 1000, 2000 and 4000 Hz) and broadband noise. Normal values are 90–95 dB SPL for tones and 70–75 dB SPL for broadband noise (Margolis 1993). Pathology affecting any of the structures of the reflex arc can lead to an increase in the threshold or obliterate the response. The acoustic reflex can also be used as an objective measure of recruitment in sensorineural hearing loss (Metz recruitment) (e.g. Thomsen 1955).
Ipsilateral measurements involve stimulating and measuring the response in the same ear; contralateral measurements involve stimulating one ear and measuring the response in the other. Combining the results of ipsi- and contralateral measurements can help identify the site of lesion within the reflex arc (e.g. Emanuel 2009). Contralateral measures are also used to avoid test artefacts that can result from interference of the probe and stimulus tones on ipsilateral measurements (Benton et al. 2004).
Esser et al. (1987) proposed comparing acoustic reflex thresholds elicited by pure tones with those elicited by narrowband noises. They found a 10 dB higher pure-tone acoustic reflex threshold in children with auditory processing disorder (APD). Kunze et al. (2016) could not find such differences in children with or without APD. Another test paradigm in occasional use is the reflex decay. In this test, an acoustic reflex is evoked by a sound signal sustained over a longer duration, usually 10 s. The reflex decay is the amount of time during this acoustic stimulation by which the reflex is sustained at a level above 50%. The normal value is ≥5 s. A shorter duration (i.e. a quicker decay) may indicate retrocochlear or other central pathology, such as myasthenia gravis (e.g. Bischoff et al. 1989). This test is, however, not used very often these days because of the prevalence of imaging techniques such as MRI.
Acoustic reflex testing can be performed on patients of any age; however, given that high signal levels are required to evoke a response even in normal-hearing patients, caution should be used, especially in patients reporting sensitivity to loud sounds, such as suspected hyperacusis, patients with lowered uncomfortable loudness levels or patients with tinnitus. Contraindications are otherwise the same as for tympanometry.
16.10 Otoacoustic Emissions
16.10.1 Nature and History of Otoacoustic Emissions
Otoacoustic emissions (OAEs) are very low-level sounds that arise during active contraction of the outer hair cells in the organ of Corti. OAEs can emerge spontaneously or be evoked intentionally. The principle of OAE measurement is the detection of these sounds.
OAEs result from the active mechanism of cochlear amplification (see Sect. 1.4), a function that plays a significant role in the sensitivity and discrimination of hearing (Heinz et al. 2001). Detection of the OAE is therefore an important objective indicator of inner ear function. It does not, however, provide information about the general status of the auditory system.
The history of OAE measurement dates back to 1978, when David Kemp first demonstrated their existence and utility. Since then, several types of OAE, which can be used in the investigation of inner ear function, have been discovered. The most important clinical applications of OAEs are the transient-evoked OAE (TEOAE) and distortion product OAE (DPOAE). The most common use of TEOAEs these days is in newborn hearing screening programmes (see Sect. 17.1) and of DPOAEs is in audiological monitoring during therapy with ototoxic drugs (see Sect. 14.9).
16.10.2 Measurement of OAEs
All forms of OAE are based on the same principle: the detection of sounds produced by the cochlea by using a microphone placed in the outer ear canal. The difference between the various OAE methods lies essentially in the stimuli presented and the processing of results.

Automated transient-evoked otoacoustic emission (TEOAE) testing in a neonate. Photograph with kind permission of the parents
In all OAE tests, the response must be distinguished from the presence of noise. Various strategies are used to aid this process, such as filtering of the response to focus only on the frequency range of interest. Averaging strategies work on the principle that the response occurs within a particular time window following the stimulus but that noise is essentially random. The results of repeated stimulation and measurement (‘sweeps’) can therefore be averaged to reduce the effects of noise. Artefact rejection is also employed in order to reject sweeps that feature recorded sound higher than a particular sound pressure level, thereby omitting transient background noise.
Measurement Conditions
The main conditions for acquiring reliable OAE results are:
Proper sealing of the probe in the outer ear canal (i.e. no gap between the probe and the ear canal, orientation of the probe towards the tympanic membrane, no blockage of the ear canal (e.g. by earwax)
Use of fully functional equipment (appropriate calibration, probe patency)
Minimal environmental/background noise and subject movement during testing
Absence of middle ear pathology (see below)
Because OAEs are produced in the cochlea but measured by a microphone in the outer ear canal, the ability to measure them is hindered by the presence of any obstruction in the middle or outer ear. Such obstructions may include amniotic fluid and debris present in the ears of neonates on the first day of life, possibly until the second day of life.
OAEs do not measure the status of the entire auditory system: they may still be present in retrocochlear pathology (e.g. auditory neuropathy).
The reproducibility of OAEs decreases with age, and they are almost never present in any cochlear pathology. The correspondence of age-related ‘normal’ hearing with OAE presence in older age is, therefore, not reliable.
16.10.3 Spontaneous OAEs
Definition and Principle
Spontaneous otoacoustic emissions (SOAEs) are typically stable pure tones that are measurable at low intensity (10–15 dB SPL) in the outer ear canal (Hall 1999) and that occur without external stimulation. Current models of SOAE genesis suggest that SOAEs arise as a consequence of a complex sustained motion of the outer hair cells in the organ of Corti (Kemp 2002).
Importance, Interpretation and Limitations
SOAEs are measurable in 30–40% of healthy young people (Burns et al. 1992). SOAEs can occur at a broad range of frequencies, but the specific frequency measured is not particularly important, as it does not relate to the outer hair cell function at a particular tonotopic area of the cochlea. The detectability of the SOAE is critically dependent on the health of the cochlea; therefore the presence of an SOAE is a particularly sensitive indicator of the integrity of cochlear function. However, the absence of measurable SOAE should not necessarily be taken to indicate pathology or hearing loss.
16.10.4 Transient-Evoked OAEs
Definition and Principle
Transient-evoked otoacoustic emissions (TEOAEs) are very brief sounds, emitted over a 0.5–4 kHz frequency range as a response to transient (i.e. very brief) acoustic stimuli (usually click stimuli) presented at intensities of 80–85 dB SPL. A series of transient stimuli with very short pauses in between is used during stimulation.
Importance and Limitations
TEOAE testing is quick (usually lasting just a few seconds for each ear), non-invasive and technically simple and requires no active participation by the patient, and the result is very reliable. These features make TEOAE testing particularly useful for neonatal hearing screening, applied in an automated version.
The presence of amniotic fluid or debris in the ears of neonates may mean that the TEOAE cannot be recorded shortly after delivery.
Newborns at risk of retrocochlear pathology, such as auditory neuropathy, should not be tested solely with TEOAEs. Automated auditory brainstem response (AABR) audiometry should be used in these cases.
Interpretation
High-level click stimuli reliably evoke a response if the hearing threshold is 20 dB HL or better across frequencies (Kemp 1978). TEOAEs can also be evoked (though less reliably) where mild sensorineural hearing loss is present (Robinette et al. 2007). The presence of TEOAE indicates healthy cochlear function (Kemp 2002) or, at worst, mild hearing loss (Ramos et al. 2013). A positive response is indicated where the signal-to-noise ratio (SNR) is 3–6 dB (i.e. the level of the response is 3–6 dB higher than that of the noise), depending on the protocol and equipment used. Modern screening devices typically include automated data analysis, with positive result (TEOAE present) indicated as ‘pass’ or similar. A negative response (‘refer’) indicates that normal hearing cannot be confirmed and the patient requires further investigation (such as rescreening or AABR testing if within a newborn hearing screening programme).
Clinical devices enable more detailed analysis of the test data for scientific and research purposes. First studies are performed in the clinical application of high-frequency TEOAEs (Goodman 2011).
16.10.5 Distortion Product OAEs
Definition and Principle
DPOAEs are responses from non-linear elements in the cochlea to stimulation by the travelling wave of basilar membrane displacement that is the physical manifestation of sound as it moves through the cochlea. The stimulus is different from that used in TEOAE testing: instead of a click stimulus, two pure tones with closely related frequencies (f1, f2) and intensity characteristics (L1, L2) are presented simultaneously to the same ear. The intermodulation of these two tones results in predictable basilar membrane activity at various different relative frequencies (known as distortion products). Clinical DPOAE testing measures the amplitude of the response at one of these frequencies, usually 2f1-f2, which has the largest amplitude.
Importance, Interpretation and Limitations
DPOAEs complement TEOAEs in clinical practice. DPOAEs seem to be less sensitive to minor and subclinical conditions of the hearing system (e.g. Kemp 2002). They allow the measurement of a wider frequency range than do TEOAEs (Kemp 2002). The response corresponds to the hearing threshold near the f2 frequency (Robinette and Glattke 2007). Furthermore, the DPOAE can be detected at higher frequencies (up to 8 or 10 kHz), and the measurement of DPOAE amplitudes seems to be a promising method for the early detection of high-frequency cochlear damage (e.g. ototoxicity, noise). DPOAEs can be detected up to a cochlear hearing loss of 50 dB HL, so they cannot be used to identify lower degree hearing impairments.
The examination is usually performed and interpreted for individually tested frequencies, though many OAE devices run through a standard battery of tested frequencies. The frequency-specific characteristic of DPOAEs means that manufacturers have been able to place on the market devices with DPOAE-based hearing threshold estimation (DP audiogram) capability. The extrapolation of DPOAE input/output function can be used to estimate pure-tone thresholds up to 8 kHz (Stavroulaki et al. 2001). The clinical importance of DPOAE is nonetheless a subject of further research.
16.10.6 Other Applications of OAEs
Sustained-frequency otoacoustic emissions are acoustic responses to the presentation of an ongoing tonal stimulus. The wide frequency range (0.5–8 kHz) of these emissions enables the study of inner ear function at specific frequencies, whereas TEOAEs and DPOAEs rely on the combined response or interaction of various areas of the basilar membrane. Because they are identifiable even in cases of severe hearing loss, sustained-frequency otoacoustic emissions may be useful as an objective tool for the quantification of hearing loss. Nevertheless, the method is still an object of study and is not routinely used in practice.
The bibliography includes some articles in which other applications of OAE have been studied, such as synchronised SOAE (Jedrzejczak et al. 2008), stimulus-frequency OAE (Ellison and Keefe 2008) and others. The utility of these methods is under study, and their clinical use in routine practice is currently marginal.
16.11 Evoked Response Audiometry
16.11.1 Impact of Evoked Response Audiometry on Objective Threshold Audiometry
The gold standard for hearing threshold assessment is pure-tone audiometry (PTA). This subjective examination requires active cooperation of the investigated subject. On the basis of this principle, a significant number of subjects (infants, psychically and mentally diseased persons, malingerers) cannot pass the examination. Objective threshold audiometry is aimed at covering this gap. Among its methods, evoked response audiometry (ERA) plays a most prominent role in newborn hearing screening and in auditory diagnostics of children and their hearing rehabilitation (Dršata et al. 2015). Optimising these methods has been a subject of intensive research, the essential goal of which is finding a method with the best correlation to the behavioural threshold (Stapells 2011).
Different stages of the auditory pathways can be studied with ERA signals. Early latency responses originate from the brainstem (the auditory brainstem response, ABR). Auditory steady-state responses (ASSR) have generators in the brainstem as well as in more central areas (Picton et al. 2003). Middle latency responses come from the primary auditory cortex and long latency response from the non-primary cortex (cortical ERA, CERA).
The more centrally the source of activity can be found, the more the activity is dependent on the arousal of the patient. Nonetheless, we are in a favourable position to measure responses from the brainstem in sleep as well as in narcosis.
16.11.2 Broadband and Frequency-Specific ABR
There are two kinds of ABR measurements: one with broadband stimuli such as clicks, to test the integrity of the auditory pathway and to determine an overall hearing threshold, and the other with frequency-specific stimuli, to estimate the hearing thresholds at these frequencies.
Jewett (1970) and Jewett et al. (1970) first described evoked responses from the brainstem. Therefore, the potentials from the brainstem were named after him, Jewett waves, enumerated I to V or J1 to J5. The sources of these waves were analysed by dipole analysis of the EEG (Scherg 1991). Scherg (1991) showed that wave I is identical with the compound action potential of the auditory nerve. Wave II is generated at the opening of the internal acoustic meatus, the porus acusticus internus. Scherg (1991) found the source of wave III in the efferent part of the ventral cochlear nucleus, still in the ipsilateral hemisphere. Waves IV and V were thought to originate from the nucleus olivaris superior and lemniscus lateralis, on the ipsi- and contralateral sides, respectively.
At any point in time after … 2.5 ms, activities in many different places in the pathway overlap.

Time courses of different stimuli are shown: left click, middle tone burst and right chirp stimulus
Although the click and broadband chirp stimuli excite the whole cochlea and generate relatively well-synchronised neural responses, these stimuli are not frequency-specific, and so these methods cannot assess the hearing levels at individual frequencies.

Schematic spectrum of a frequency-specific notched-noise stimulus. The noise (in grey) is notched at the stimulus frequency and masks the possible side bands of the tone burst (blue line)
Comparable to the application of broadband chirps for overall threshold ABR measurements, narrowband chirps can also be used for frequency specificity, for which a part of the whole chirp is cut out and covers, for example, only one octave (Wegner and Dau 2002). Especially at low frequency, this narrowband chirp is more efficient than tone bursts (Mühlenberg and Schade 2012). Narrowband chirps have been proven to generate a frequency-specific response, with a reduction in the test time in newborns (Ferm et al. 2013).
The reliability and technical mastering of the described methods have resulted in commercially available ABR systems that offer different kinds of stimulation.
16.11.3 Auditory Steady-State Responses
Auditory steady-state responses (ASSR; steady-state evoked potentials, SSEP; amplitude-modulation following response, AMFR) are auditory evoked potentials from the brainstem and more central structures (Beck et al. 2007). The stimuli are usually continuous pure tones, modulated mostly with modulation frequency around 80 Hz in the amplitude alone or both the amplitude and frequency domains (see Sect. 16.12).
16.11.4 Cortical ERA
Cortical ERA (CERA), also called measurement of late auditory evoked potentials (LAEP), is much less sensitive to muscle activity than ABR, with the drawback that it is more dependent upon vigilance and attention. Different kinds of stimuli come into consideration: clicks, tone bursts (frequency-specific with a length of ≥50 ms) or speech signals (consonant-vowel or vowel-consonant-vowel). The examination requires the patient’s alertness or at the most video sedation.
The reliance of CERA on the patient’s mental vigilance makes the method suitable mostly for calm but awake children and adults (Stapells 2002). The observed response pattern is in a time window between 50 ms and 1000 ms and in a frequency range from near 0 Hz up to 600 Hz.
The CERA has its diagnostic value in the examination of the auditory pathway beyond the brainstem up to the cortical areas. The clinical application of CERA is not widespread and is at its beginnings. It can be useful in questions of maturation (after hearing aid use or cochlear implant), auditory neuropathy, auditory processing disorders, psychogenic hearing disorders, autism, lesions of the central pathway or aggravation and simulation (Walger et al. 2014).
16.11.5 Specific Issues of ERA
Patient Preparation and Test Implementation
For measurement of ERA—broadband and frequency-specific—the patient has to be relaxed and quiet. This means in the case of small children that they have at least to sleep. In the case of well-collaborating parents of children without neuropsychological disorders, the examination can be performed in a deep natural sleep following previous sleep deprivation. However, sedation or general anaesthesia is often to be considered for infants. Among sedatives and anaesthetics, different drugs are used (e.g. chloral hydrate, midazolam, ketamine and others), with variable pharmacokinetics, side effects and brain activity influences. Anaesthesia is accompanied by risks and a high logistic effort and therefore to be avoided if possible. The alternative is natural sleep. Sleep can be induced by oral administration of melatonin, a naturally produced hormone adjusting the circadian rhythm (Schmidt et al. 2007).

Electrode positions are shown for ERA measurement. Photo from electrodes with kind permission from Ambu GmbH, photo from preamplifier of the BERA system Corona with kind permission from Pilot Blankenfelde medizinisch elektronische Geräte GmbH, photo of child with kind permission of the parents

For stimulation in ERA measurement, a headphone (left, type DT 48 with kind permission from Beyerdynamic), a transducer for bone-conduction ERA (middle, type B-71 with kind permission of Radioear) and insert earphones (right, with kind permission from 3M) can be used. The earphones are shown with different ear tips for different sizes of the ear canal
Frequency-specific ERA examination is carried out principally in an identical way as for conventional click-evoked ABR. Obviously, the estimation of hearing thresholds at individual frequencies takes a significantly longer time, and feeble responses to the specific stimuli are more sensitive to the ambient noise and measurement conditions than with a conventional click-evoked ABR.
Estimated Audiogram (EA)
The ABR objective threshold audiometry is based on detection of the most prominent Jewett V wave. The key point for the correct hearing level estimation is a faultless identification of the Jewett’s wave complex. An expert audiologist takes the pattern of waves, their latencies and intensities and the age of the patient and measurement conditions into account when identifying Jewett waves and the thresholds. Evaluation algorithms specified by the manufacturers, based on automatic ERA analysis, may help to eliminate possible human errors. Nevertheless, the results of these algorithms have to be scrutinised critically, especially in nonoptimal measurement conditions.
ASSR measurements generally follow a complete automatic protocol. Thresholds at different frequencies as well as in both ears can be measured simultaneously (Korczak et al. 2012). This possibility leads to a shorter measurement time for a complete threshold estimation. Nevertheless, the major advantage of ABR (controlled by an audiologist) over ASSR remains the traceability of the results and the information about the auditory pathway given by the wave pattern above thresholds.
Level-Latency Curves

Level-latency curves are shown for different pathologies. In grey is the normal range of latencies for wave V shown, in blue their mean. In red the divergent latencies for the noted pathologies are shown
In conductive hearing loss, the stimulus reaches the cochlea quieter than normal. Therefore, the curves are parallel but shifted to higher intensities. The difference Δt remains normal.
In sensory hearing loss, the curves at high levels are almost normal and prolonged only at levels near threshold. In the case of low-frequency loss, tV can even be shortened because of the lacking later low-frequency portion of the signal. The curve shape conforms to a defect of the outer hair cells and normal neuronal processing.
In contrast, in retrocochlear hearing loss latency, tI is normal but tV is severely prolonged. The wave pattern can also be irregular, e.g. in auditory neuropathy (Schmidt et al. 2012).
The interpretation of the level-latency graphs provides important hints for diagnostics. But be aware: in infants it is more difficult because of maturation of the auditory pathway, so additional diagnostics should be taken into account.
Bone Conduction in ABR
If conductive hearing loss is suspected, measurement of bone-conduction ABR is indicated. Unlike in (the normal) air-conduction ABR, the stimulus is presented by a transducer (Fig. 16.28) as similarly used for bone-conduction behavioural thresholds. The transducer ideally is held by an elastic band or clamp. The intensities used reach a maximal of 50 dB, which is sufficient for diagnostics of conductive problems. A large early artefact may occur owing to the vibrating mass, but the ABR response to frequency-specific stimuli appears after that artefact (Stapells and Oates 1997). In cases of threshold differences between ears, masking comparable to that for behavioural measurement is necessary. To identify conductive or mixed hearing loss, ear microscopy, tympanometry, stapedius reflex measurement and otoacoustic emissions should be taken into account.
Hearing Rehabilitation Based on ERA Results
The estimated audiogram is not identical to the behavioural threshold of hearing examined with PTA. The correlation of estimated audiogram and the behavioural threshold is strongly influenced by the chosen method and measurement conditions, individual hearing loss and condition (maturation) of the auditory pathways as well as experience of the audiologist. Nevertheless, in preschool children, infants and non-cooperating young patients, threshold estimation by physiological measurements is the best that can be achieved. In group means, the thresholds are within 10–15 dB of the behavioural threshold. In cases of steep hearing loss, the difference may be larger (~20 dB) in the range of the steep slope. The hearing loss is rather underestimated (Johnson and Brown 2005). The results of the electrophysiological measurements have to be checked for plausibility in synopsis with otoacoustic emissions, middle ear status, stapedius reflex measurements, sound field behavioural audiometry and language development of the child. The estimated thresholds are suitable for establishing the basal hearing aid settings. It is necessary to control the fitting, the development of hearing and language of the children regularly.
The results of objective threshold audiometry in the hearing rehabilitation process must be continually monitored, also with the help of behavioural methods, as soon as their achievement is possible.
16.12 Indication and Interpretation of Auditory Steady-State Responses
16.12.1 Characteristics of Auditory Steady-State Responses (ASSR)

The site of the ASSR response production (the author’s own study based on the materials from the Department of Phoniatrics and Audiology, University of Medical Sciences in Poznań). ECochG electrocochleography; ABR auditory brainstem response; MLR middle latency response; SP sustained potential; LAEP late auditory evoked potential
16.12.2 Aim of the ASSR Production
The ASSR test is aimed first at producing a simple objective measurement of hearing whose result correlates directly with the audiogram; it is an instrument complying with the principle of audiological diagnostics, the ‘cross-check principle’ according to Jerger and Hayes (1976), in particular in the diagnostics of young children. The automatic analysis of the ASSR is based on a transformation into frequency space, e.g. by a fast Fourier transform (FFT). In the next step, it is evaluated by statistical testing to determine if there is a response to the stimuli at the modulation frequency or not. The methods used can be the calculation of phase coherence values or the F-test (Dobie and Wilson 1996). Phase coherence is calculated if the measured signal has random phases: then there is no response; if in each repetition the phase is the same and related to the stimulus, there is a valid response. In the F-test the energy at the modulation frequency is compared with that of neighbouring frequencies. Different variations and flavours of these algorithms are possible (Picton et al. 2003). Each device manufacturer uses its own estimation algorithm, and usually they do not disclose their methods.

An example of the ASSR registration in an 11-year-old child (legend: X-axis, frequency in kHz; Y-axis, sound pressure level in dB)
16.12.3 Indication and Interpretation of ASSR
The indication for the ASSR test is the need to obtain an objective audiogram, besides its use in newborn hearing screening. As the ASSR method makes use of tonal, frequency-specific stimuli (similar to that in the audiometric test), the test result can be compared with the behaviourally obtained audiogram. It can be a perfect instrument for objective hearing tests in children and uncooperative patients through earphones and in a free acoustic field through loudspeakers. The problem of this method is the need for a correction to estimate the hearing threshold. In normal-hearing adults and older children, this correction is averaged at 11 dB for 2 kHz up to 17 dB for 500 Hz (Tlumak et al. 2007). In hearing-impaired adults, it varies from 8 to 14 dB. Despite this correction the method is considered to give a good estimate of hearing threshold. In children <6 years and infants, compared with ABR thresholds—which may lie 10 dB above behavioural thresholds—the ASSR for normal hearing is 17 dB, 13 dB, 13 dB and 9 dB worse than ABR in normal hearing and 9 dB, 9 dB, 9 dB and 6 dB worse in patients with hearing loss at 500 Hz, 1000 Hz, 2000 Hz and 4000 Hz, respectively (Van Maanen and Stapells 2010). These corrections vary in different publications (e.g. Chou et al. 2012; Luts et al. 2006; Rance et al. 2005) because of different study groups, stimuli, measurement time, noise floor and other parameters. Considerable variability in interpersonal and intrapersonal results is noticed. A large number of audiologists seem to indicate that so far the method represents poor diagnostic value (Pruszewicz and Obrębowski 2010; Tlumak et al. 2012). Owing to the good correlation of the ABR and ASSR thresholds, the method is quite usable. In commercial ASSR systems, an automatic correction is normally included; therefore the result of measurement is an estimated audiogram. The audiological assistant or semi-skilled examiner does not need to know much about the interpretation of the response, as in click or notched-noise ABR, as the measurement system delivers an audiogram. But this audiogram should be interpreted with caution depending on the degree of hearing loss, age and measurement conditions.
16.13 Promontory Test
Aim and Method
The promontory test assesses whether external electrical stimulation at the cochlear promontory generates an auditory percept. The test was initially introduced to ascertain deaf patients’ candidacy for cochlear implantation, since conductivity of the auditory nerve is an essential prerequisite for a successful outcome after implantation.
The subjective test aims to assess thresholds and dynamic ranges of hearing sensation and can be performed in an outpatient procedure, in which the patient is seated and the external ear canal is under local anaesthesia. Under microscopic control, a needle electrode is then transtympanically positioned at the cochlear promontory, via the external ear canal. Different stimulation paradigms have been introduced to determine whether a patient will benefit from electrical stimulation. Commonly used stimulation rates include 50, 100 and 200 Hz. During stimulus application, the patient is asked to describe the hearing sensation associated with the different stimuli. For each stimulation rate, the patient indicates if there is an increase in perceptual loudness as stimulant current is increased. Further, the discrimination between the different stimulation rates is assessed. Thresholds and dynamic ranges can then be documented for the different stimulation rates.
Alternatively, to avoid the application of a needle electrode, the promontory test can be modified, and a ball electrode can be positioned in the external ear canal in contact with the tympanic membrane.
Interpretation and Diagnostic Value
In the early years of cochlear implantation, in which most patients scheduled for implantation suffered from complete deafness, the promontory test was an essential part of candidacy assessment. Since then, the indications for cochlear implantation have expanded to include patients with profound sensorineural hearing loss and residual hearing. In these cases, the promontory test is usually postponed, because reliable thresholds in pure-tone audiometry describe the basic conductivity of the auditory nerve. The promontory test has, however, been suggested to be a useful tool in predicting postoperative speech perception in patients at risk of poor auditory nerve functioning (neuropathies), especially in postinflammatory conditions such as meningitis, or after long auditory deprivation (Alfelasi et al. 2013).
16.14 Electro-audiometry
In deaf or nearly deaf patients, the only possibility of evoking an impression of hearing is the electrical stimulation of the auditory system. Electrical stimulation can be used to measure the capability of the auditory pathway, as does acoustic brainstem audiometry. This examination can provide information about the status of the auditory pathway as well as thresholds for electrical stimulation to be used in cochlear implants.
Electro-audiometry may be used before cochlear implantation to examine if the post-cochlear system is adequate for electrical stimulation via a cochlear implant. There are two possibilities of measurement: the patients report their subjective impression of the stimulation (the so-called promontory test; see Sect. 16.13), or, perhaps more reliable, the nerve response is recorded with synchronous averaging by auditory brainstem response acquisition equipment. Nevertheless, its predictive value is low and the method has disappeared from medical guidelines (Lesinski et al. 1997).


Measurement of evoked compound action potentials with a cochlear implant (Software Custom Sound EP ver. 5.0, Cochlear Ltd., Australia). In the left panel, compound action potentials are shown for one electrode (no. 6) at increasing current levels (current levels are arbitrary units of the manufacturer and can be translated to currents in microampere range). In the middle panel, the amplitude differences of the marked positive and negative peaks are drawn over the current levels. The linearity of the amplitudes enables the extrapolation of a threshold. In the right panel, the extrapolated amplitude thresholds of the evoked compound action potentials are drawn for different electrodes. They generate a profile for speech processor fitting
The test is controlled by proprietary software of the implant producer. As result of the measurement, threshold values are obtained that can be used for adjusting the speech processor programme of the implant recipient (Smoorenburg et al. 2002). This measurement is useful in non-cooperative patients such as small children, but it also enables adequate fitting of the speech processor in patients with intense tinnitus.
An electrically evoked brainstem response can also be measured in special cases, for example, if there is doubt that the auditory pathway is functioning well. The stimulation is executed with intra-cochlear electrodes and acquisition of the response with surface electrodes as in normal brainstem response measurement (Brown 2003).
16.15 Balance/Vestibular Assessment in Children
16.15.1 Developmental Balance Assessment in Children: Development of Balance, History and Observation
Development of Balance
Balance is a complex modality that develops with input from the visual, proprioceptive/somatosensory and vestibular systems. This sensory information is integrated and processed within the central nervous system and results in outputs that help stabilise our eyes and body in space through the vestibulo-ocular (VOR) and vestibulospinal (VSR) reflexes, respectively, and help in perception and awareness of our posture and movement in space through cortical connections. Development of balance depends on the development of each of these sensory systems and on the development of the integrating pathways.
The vestibular labyrinth and receptors are developed to an adultlike form by 32-week gestation. The vestibular nerve is the first cranial nerve to complete myelinisation. The slow (reflexive) component of VOR can be seen at birth, but the fast (saccadic or central) component is immature and continues to develop until the age of 2 years. Absence of the VOR by the age of 10 months is an abnormal finding (Eviatar and Eviatar 1979; Fife et al. 2000). Infants prefer visual inputs to maintain balance, whereas adults rely more on somatosensory information. Infants perform better under conditions of sensory loss [e.g. visual difficulties] than conditions of sensory conflict, indicating that the central vestibular integrative pathways are immature. The role of the vestibular system in postural control depends on these integrative pathways, and the ability to resolve intersensory conflict is mature approximately at the age of 15 years (Steindl et al. 2006; Shinjo et al. 2007).
Balance difficulties in children may present as delayed motor milestones, poor tone and floppy posture, frequent falls and unusual gait. They may be related to visual, neurological or vestibular pathology or psychological factors. Cardiac pathology and metabolic disturbances such as hypoglycaemia should also be considered. A comprehensive balance evaluation should include a clinical assessment of all the above conditions. The vestibular aspects of a child’s balance can be assessed from history, examination and selected investigations.
History
The onset and progress of the balance difficulty is often a pointer to its cause. In combination with deafness, poor tone and delayed walking in a neonate are likely to be due to a congenital vestibular hypofunction. Sudden vertigo, resolving in days, preceded by a viral infection and followed by fluctuating imbalance is likely to be related to vestibular neuritis. Associated conditions should be noted, as balance difficulties occur in Pendred’s syndrome (with deafness and goitre), Usher syndrome (with deafness and retinitis pigmentosa), CHARGE syndrome (with deafness and dysmorphism) and migraine (often with headaches and vertigo). Family history is a pointer to inherited conditions (i.e. affected parents/siblings in branchio-oto-renal syndrome). Perinatal history is important, as deafness and cerebral palsy can be related to neonatal jaundice and perinatal hypoxia.
Observation and Examination
The key to successful examination of balance in a child, particularly the young toddler, involves knowledge of normal development, a keen eye for observing the child during natural activity and the ability to engage children in play. The general examination must include looking for dysmorphism, otoscopy, examination of the nose and throat, looking for neurocutaneous markers and a complete systemic examination to look for associated conditions and syndromes. A detailed neurological examination is mandatory. Clinical vestibular examination includes assessing the VOR, the vestibulospinal reflex (from postural tests) and the developmental reflexes.
16.15.2 Tests for Posture/Vestibulospinal Reflex
Romberg’s test: the child stands with feet together, and sway is noted with eyes both open and closed. A variation is the sharpened Romberg’s test whereby this stability is evaluated in a heel-to-toe stance.
Foam test: the child stands on foam with eyes open and closed and sway is noted.
Unterberger’s test: the child is asked to march on the spot with the eyes closed and the deviation is noted.
Tandem gait: the child walks heel to toe and steadiness is noted.
The postural tests are limited by lack of specificity for vestibular dysfunction and paucity of normative data for children.
16.15.3 Tests for Eye Movements and VOR
The VOR in children is dependent on the attention and state of arousal of the child, unintended ocular fixation due to light leaks and difficulty with head stabilisation during testing. Examination of children is helped by using child-friendly visual targets (e.g. stickers, toys). Begin by looking for a squint and spontaneous nystagmus. The best way to look for vestibular nystagmus is by using Frenzel’s or Video-Frenzel’s glasses in the central, right and left gaze (angle of gaze should be <30°). Smooth pursuit is best checked with a toy and optokinetic nystagmus with a striped drum with child-friendly pictures.
Halmagyi’s Head Thrust
The child’s head is gently rotated by approximately 30° in the yaw plane (about the vertical body axis) while asking the child to focus on the examiner’s nose. A rapid head thrust is incorporated ensuring maintenance of visual fixation. A ‘catch-up’ saccade indicates loss of function of the ipsilateral lateral semicircular canal.
Head-Shaking Nystagmus
The child’s head is briskly rotated in the yaw plane with eyes closed for about 30 s. The eyes are then opened and examined preferably using Frenzel’s/Video-Frenzel’s glasses. Vestibular asymmetry causes nystagmus beating away from the side of the lesion.
Vibration-Induced Nystagmus
This can be done with a vestibular vibrator applied to the mastoid process for approximately 10 s. The test also elicits vestibular asymmetry. The nystagmus begins when the vibration is applied and beats opposite to the direction of the lesioned side.
Dynamic Visual Acuity
Children may be evaluated with modification of the Snellen eye chart using child-friendly characters. The child is asked to read this chart from a distance of 1.5 m with the head steady. A baseline visual acuity is obtained. The child’s head is rotated in the yaw plane at approximately 1–2 Hz. A loss of three lines or more of visual acuity may indicate a VOR deficiency.
16.15.4 Tests for Neonatal Reflexes
In the article of O’Reilly et al. (2011), various approaches are presented.
Moro Reflex
This is elicited by holding the child supine and allowing its head to drop by approximately 30° in relation to the trunk. There is extension and abduction of the arms with fanning out of the fingers followed by adduction of the arms at the shoulder and crying. This reflex normally disappears by the age of 5–6 months. Its presence before this age is indicative of vestibular function.
Farmers Rotation Test
The baby is held at arm’s length (facing the examiner) and rotated in one direction around the examiner. There is a deviation of the eyes and head in the direction of the rotation, in the presence of vestibular function. This response can be elicited up to the age of 6 weeks. At a later age, the nystagmus supersedes the eye deviation.
Parachute Reflex
The child is held face down horizontally and moved downward suddenly. This causes an extension and abduction of the lower limbs and outstretched hands. A backward and sideward parachute reflex can also be elicited. This can occur beyond the age of 5 months and is indicative of vestibular function.
Head Righting Reflexes
These develop by the age of 4–6 months. The child’s trunk is held 30° from the vertical, and a normally responding infant will tilt its head so as to remain vertical. At about age 5 months, the child will also move the lower limbs away from the side to which they have been tilted.
16.15.5 Other Tests: Dix-Hallpike Positional Test
This involves rapidly taking the child from a seated to a lying back position with the head turned to one side (right or left). The eyes are observed for nystagmus. The child is then rapidly taken to the seated position and the eye observation is repeated. The presence of horizontal, rotatory, geotropic nystagmus with vertigo after a short latent period and fatigue on repeated testing occurs in benign paroxysmal positional vertigo (BPPV).
16.15.6 Vestibular Investigations
Adequate preparation for the tests is important. Vestibular sedatives are withheld for 24–48 h prior to testing, and it is important that the child eats only a light meal before the appointment.
Electronystagmography and Video-Oculography
These are techniques used for recording eye movements during rotational chair testing. They can also be used for recording eye movements for gaze, smooth pursuit, saccades and optokinetic nystagmus. It is important to understand the differences between child and adult traces, and normative data should be collected. Testing in a dark room, the need for placing electrodes or goggles and the rotational chair stimulus itself make these tests challenging in children. Furthermore, inattention and alertness will influence responses.
Video Head Impulse Test (vHIT)
could be used successfully to test dizzy children (Khater and Afifi 2016). This test provides vestibular ocular reflex analysis of high-frequency vestibular activity of the horizontal and vertical semicircular canals. It is based on Halmagyi’s head thrust response. With the video head impulse test, eye movements can be recorded by a high-speed lightweight video camera and mini-gyroscopes fitted to eye glasses (MacDougall et al. 2009; Petrak et al. 2013).
Computerised Rotational Chair Testing
The response to impulsive and sinusoidal rotation indicates the presence of vestibular function. The limitation is that this test assesses the overall vestibular function rather than for individual ears. The advantage is that children may sit on their mother’s lap and this test is better tolerated than the caloric test. Using this method, young infants can be examined for VOR. The gain, phase and time constant of the nystagmus is calculated. In the event that recording of the nystagmus is difficult, simple observation with an infra-red camera is useful in detecting per-rotatory and post-rotatory nystagmus. VOR gain increases linearly as a function of age from 3 to 9 years (Casselbrant et al. 2010).
Bithermal Caloric Testing
Warm and cool irrigation of water or air may be used. This test allows individual ear testing of vestibular function and, from the Jongkees formula, will give the canal paresis and directional preponderance. Studies of ice-cold caloric responses in infants indicate that nystagmus is observed by the age of 6 months in full-term infants (Eviatar and Eviatar 1979). Practically, children younger than 5 years of age may not tolerate this test well.
Computerised Dynamic Posturography
The test can be used in children as young as 3–6 years of age (Foudriat et al. 1993). The sensory organisation test (SOT) is the commonest subtest used. This test consists of six conditions of varying combinations of fixed and moving platforms and visual surroundings done with eyes open or closed. The child must try to maintain the best balance possible despite conflicting clues. Normative data should be obtained, although it has been shown that younger children perform worse than older ones (Valente 2007). Special adaptations should be made for testing children. Information may be gained regarding how well a patient integrates visual, vestibular and proprioceptive cues and whether there is an overdependence on one modality.
Vestibular Evoked Myogenic Potentials Test (VEMPs)
Both cervical and ocular VEMPs (cVEMPs and oVEMPs) have been done in children to evaluate the integrity of the otolith system (Valente 2007; Wang et al. 2008; Lin et al. 2010). cVEMPs are commonly recorded from the sternocleidomastoid muscle and test the vestibulocollic reflex, whereas the oVEMPs assess the otolithic VOR. Rectification methods may be employed to correct for the difference in background muscle activity. Latency, threshold and asymmetry may be measured. VEMPs are well tolerated and can give information on individual ear otolith function. VEMPs have been demonstrated in neonates and children and the wave latencies may be shorter than in adults (Valente 2007).
Subjective Visual Vertical/Subjective Visual Horizontal (SVV/SVH) Test
This test involves setting a linear marker to the vertical or horizontal in a dark room, in the absence of visual clues. The patient is asked to adjust the marker to his/her subjective vertical/horizontal. The degree of deviation is recorded. This can be done in older children but head restraint may be required to avoid the effect of head tilt. The values may be abnormal in acute unilateral vestibular lesions.
16.16 Evaluation of Speech Communication in Hearing-Impaired Children
16.16.1 Introduction: Relation of Hearing, Language and Speech
According to Aslin and Smith (1988) and Carney (1996), basic sensory perception (sound detection) is followed by coding perceptual representations (phonetic discrimination) and cognitive/linguistic processing (word recognition). These levels are mirrored in speech production by primitive vocalisations, complex vocal utterances (babbling) and phonemic or syllabic speech patterns (Eisenberg et al. 2007). Hearing loss (HL) in early childhood impairs the development of speech recognition and speech production by hindering the generation of correct recognition categories, linguistic and motoric patterns. Recognition of word boundaries, of syllables, of prosodic elements of speech, of sound patterns in words is basically essential for language development in early infancy.
Results in speech recognition and production depend on the type and degree of hearing loss, onset of manifestation, age at detection and start of intervention, additional handicaps, kind and quality of hearing device supply, communication mode, support by parents and intensity of therapeutic training.
Prolonged phase of primitive vocalisations.
Delay in onset of babbling and differences in babbling behaviour, less consonantal types.
Delay in development of phonemic/syllabic speech patterns.
Lagging behind in vowel and consonant production.
More problems in recognising consonant than vowel contrasts.
More problems in recognising consonant manner and rear place than consonant voicing and front place.
Substitution particularly for fricatives and affricates.
Mild to moderate hoarseness.
Nasal resonance problems (Eisenberg et al. 2007).
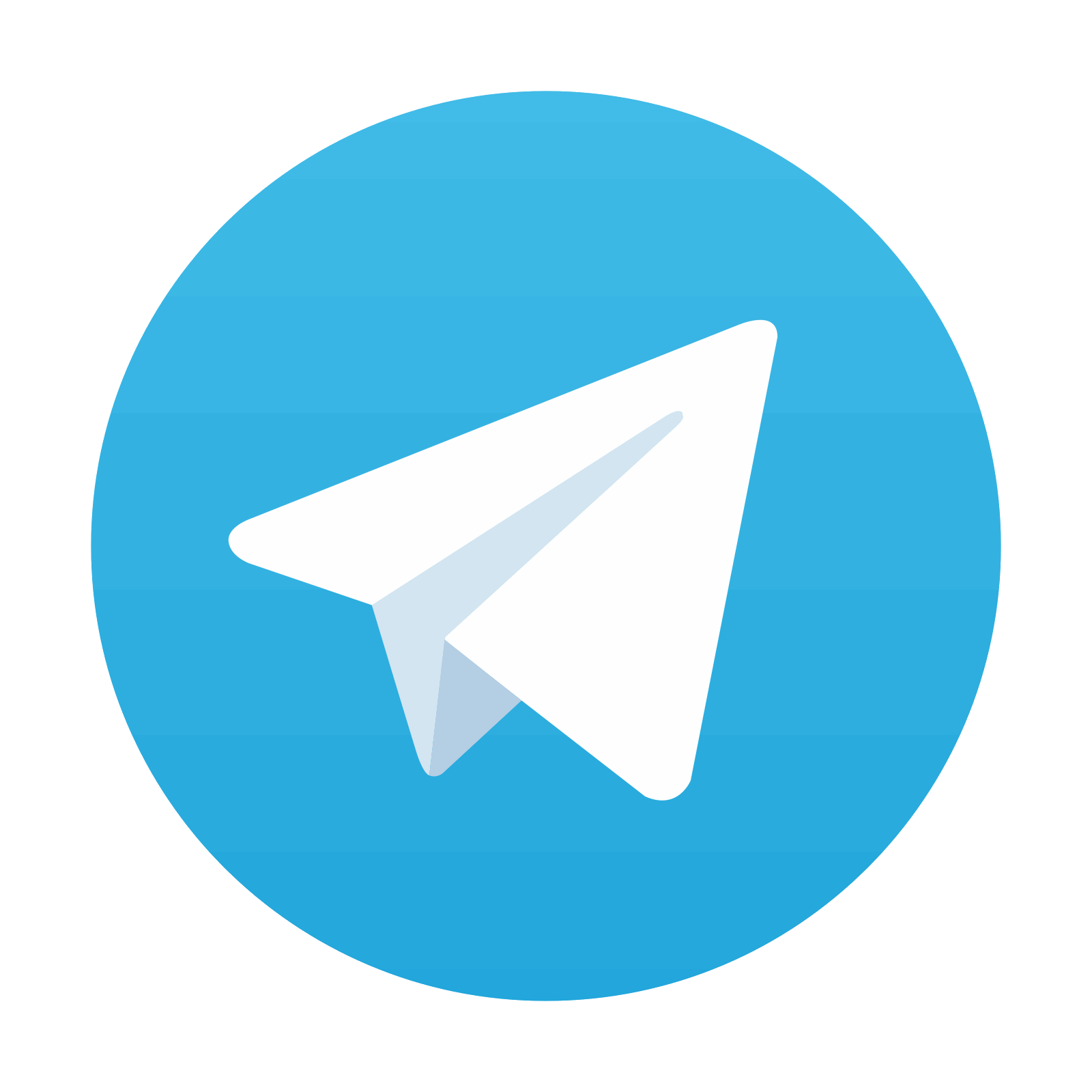
Stay updated, free articles. Join our Telegram channel

Full access? Get Clinical Tree
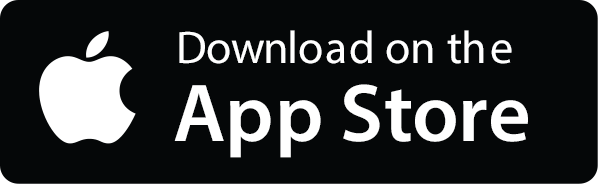
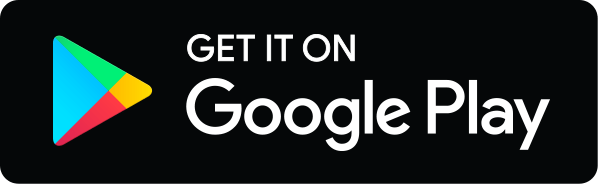