Anatomy and Physiology of the Auditory System
This chapter presents an integrated description of the anatomy and physiology of the auditory mechanism. The information presented here can be found in greater detail in textbooks and hundreds of journal publications; here information from Abele and Wiggins (2015), Barin (2009), Dallos (1973), Goldberg et al. (2012), Goutman, Elgoyhen, and Gomez-Casati (2015), Hudpseth (2014), Lemmerling, Stambuk, Mancuso, Antonelli and Kubilis (1997), Luers and Hüttenbrink (2016), Olson, Duifhuis and Steele (2012), Pickles (2013), and Volandri, Di Puccio, Forte, and Manetti (2012) has been used to organize a contemporary description of the relevant structures and functions of the auditory and vestibular systems.
A comprehensive understanding of the auditory mechanism is critical to anyone interested in pursuing a career in audiology, speech-language pathology, hearing or speech science, or any other career related to communication. There are several reasons for this. First and foremost, children learn speech and spoken language through their auditory mechanism. Second, an understanding of the normal auditory mechanism allows appreciation for the way in which various diseases and conditions affect hearing. Third, knowledge of the auditory mechanism is essential to understanding the design and purpose of formal tests of hearing—the types of tests an audiologist performs to identify and diagnose a hearing problem. Even the simplest evaluation of hearing, such as a pure-tone audiometric test (when a single-frequency tone is presented through headphones and the person being tested is asked to raise a hand each time it is heard) is designed with knowledge of the anatomy and physiology of the cochlea, the end organ of hearing, and central auditory pathways. Other, more advanced evaluations of the hearing mechanism also use sound energy to estimate the status of different parts of the auditory system. Some of these diagnostic tests require no behavioral response from the listener; that is, they depend on physiological reactions to sound energy that can be measured automatically. Others, like the pure-tone audiometric test, are designed to elicit a behavioral reaction to sound energy characteristics controlled by a tester. This latter class of tests depends on a knowledge of the discipline called auditory psychophysics, the topic of Chapter 14. Fourth and finally, assistive hearing devices, ranging from a behind-the-ear hearing aid to a cochlear implant, are based on principles of normal auditory structure and function. It is the understanding of such principles that makes possible the design of devices that can help override the limitations of an impaired auditory mechanism.
Discussions of peripheral auditory anatomy typically classify structures as belonging to one of three major divisions: the outer ear, the middle ear, and the inner ear. Much of the peripheral auditory mechanism is encased in the temporal bone, one of the several bones forming the skull. The discussion of the inner ear also includes material on the vestibular system, which is technically not part of the peripheral auditory mechanism but is closely related to the cochlea. The chapter concludes with discussion of the neural component—the auditory nerve and auditory pathways—of the system.
Figure 13–1 shows the complex shape and notable landmarks of the temporal bone. Figure 13–1A provides a view of the lateral (side) surface of the temporal bone (boundary sutures shown by the red trace). The temporal bone shares boundaries with the occipital bone in back, the parietal bone above, and the sphenoid bone in front. The mastoid process of the temporal bone is behind and slightly below the bony external auditory meatus, the small tube leading from the outside of the skull to the tympanic membrane (eardrum); the entrance of this tube is seen when looking at the side of the head into the ear. The outer part of the tube is enclosed in cartilage, and the remaining two-thirds of the external auditory meatus is enclosed in the temporal bone. Deep to the mastoid process, within the mastoid bone, are air-filled cells that communicate with the air-filled middle ear cavity.
Figure 13–1. A. Sagittal view of skull showing the boundaries of the temporal bone, in red. B. Interior of the base of the skull, viewed from above with top half of skull removed. continues
Figure 13–1. continued C. Lateral (left) and medial (right) surfaces of the complexly shaped temporal bone.
Figure 13–1B shows the interior base of the skull from above as if the top half has been removed. Note the position of the temporal bone relative to the sphenoid bone (in front) and occipital bone (behind). The temporal bone projects toward the midline, forming part of the base of the skull. The temporal bone has a broad lateral base and narrows to a blunt tip at its medial termination point. The more medial part is called the petrous part of the temporal bone. (The petrous part is not visible on the lateral surface of the temporal bone as shown in Figure 13–1A.) The petrous part of the temporal bone encases the structures of the inner ear and forms several walls of the middle ear cavity. Note the opening of the internal auditory meatus, a narrow canal roughly 10 mm in length through which nerve fibers run from the cochlea (inner ear structure of hearing) and vestibular apparatus (inner ear structure of eye-head coordination and balance) on their way to the brainstem. The opening of the internal auditory meatus into the base of the skull is in the posterior part of the petrous portion of the temporal bone. Auditory nerve fibers running in the opposite direction, from the brainstem to the cochlea, also travel through the internal auditory meatus as part of the auditory nerve, as well as nerve fibers associated with the facial nerve (cranial nerve VII). The primary blood supply to the inner ear, the labyrinthine artery which is a branch of the basilar artery which ascends the ventral surface of the brainstem (see Chapter 15), also travels to the cochlea and vestibular apparatus through the internal auditory meatus. The nerve fibers plus the labyrinthine artery emerge at the inner opening of the internal meatus.
The complexity of the temporal bone is best appreciated in Figure 13–1C, which shows it disarticulated from the other bones of the skull. The lateral surface of the bone is shown on the left, the middle surface on the right. The right-hand image shows the petrous part of the temporal bone with its wedge-like projection into the middle part of the skull base.
PERIPHERAL ANATOMY OF THE AUDITORY SYSTEM
Figure 13–2 shows an artist’s rendition of the peripheral anatomy of the auditory system. The phrase “peripheral anatomy of the auditory system” refers to all structures involved in audition that extend from the pinna to the entry point of the auditory nerve into the brainstem. The structures are shown as if the head has been cut into front and back halves, with the front half removed. The image shows the peripheral auditory mechanism separated into three major parts: the outer ear, the middle ear, and the inner ear. The outer ear plus middle ear are components of the conductive part of the auditory mechanism; the inner ear and auditory nerve are the sensorineural parts of the mechanism. The tympanic membrane is included as a component of both the outer and middle ears, because one side of the membrane faces the external auditory meatus and the other side faces the middle ear cavity. Figure 13–3 is a schematic chart of these divisions. Both Figures 6–2 and 6–3 should be referred to frequently throughout the following sections.
Figure 13–2. Coronal-plane view (head cut in front and back halves, view from the front) showing the structures of the peripheral auditory system and their divisions into the outer, middle, and inner ear.
OUTER EAR (CONDUCTIVE MECHANISM)
The outer ear includes the pinna (or auricle) and the opening into the external auditory meatus (abbreviated EAM in Figure 13–3; also called the external auditory canal). Part of the tympanic membrane (eardrum)—the sheet of tissue that terminates the external auditory meatus—is also considered a structure of the outer ear. The tympanic membrane is the boundary between the outer and middle ear.
The pinna (also called the auricle) is composed of cartilage and fat tissue. In humans, the pinna collects and directs sound energy into the external auditory meatus, toward the tympanic membrane (eardrum). In most humans, the pinna is not a movable body part, at least not to the extent as in animals (like your cat) that use muscles to aim their pinnae, with great flexibility, at interesting sounds.
Careful examination of a human pinna shows many creases, folds, and cavities. Anatomists have names for all these features, perhaps as many as 15 (or more) anatomical landmarks on and within the pinna. Figure 13–4 shows a human pinna with seven labeled landmarks. Pinna morphology varies a great deal across individuals; the seven chosen landmarks in this figure are observed in most individuals.
Figure 13–3. Schematic diagram summarizing anatomical components of the outer, middle, and inner ear and their relationship to the functional distinction between conductive versus sensorineural auditory components.
In humans, the shape and structures of the pinna play a role in the ability to localize sound sources that are higher or lower relative to a horizontal plane running through the head, at the level of the tragus. What is known is that the pinna, because of its shape and morphological characteristics, modifies the amplitudes of frequency components of sound waves striking the head. These subtle modifications provide cues to the location of a sound source in the up-down dimension.
The concha is the shallow depression in the pinna, a sort of foyer to the external auditory meatus. As shown in Figure 13–4, the concha may be separated into two chambers by a raised bar of cartilage that is a continuation of the crus of helix, the helix being the folded edge of the pinna. The concha collects sound waves and directs them into the external auditory meatus.
Inside the helix and anterior to it is the antihelix, a ridge of cartilage that follows the curve of the helix from the bottom to near the top of the pinna. As the antihelix approaches the top of the pinna, it splits into two ridges, often in a “Y” pattern, leaving the triangular fossa as the small cavity between them. This fossa serves primarily as an anatomical landmark, sometimes used to identify embryological malformations of the external ear.
The tragus is a small, rounded projection from the front of the external ear, toward the rear of the head. Apart from its obvious value as a prominent anatomical landmark, the tragus plays a role in locating sound sources in the up-down and front-back dimensions relative to the head.
The role of the pinna in sound localization may be small in humans, but in some cases significant. Chapter 14 presents information on how anatomical characteristics of the pinna may affect cues to the localization of sound in the up-down dimension relative to the head. The substantial amount of variation in pinna anatomy across individuals may account partially for findings in the literature of substantial cross-person variability of sound localization skills.
External Auditory Meatus (External Auditory Canal)
The external auditory meatus is a tube extending from the interior of the pinna, seen as a small opening in the concha (see Figure 13–4), to the tympanic membrane. Meatus is a Latin term meaning opening or canal. The entrance of the external auditory meatus, into which you fit your earphones or earplugs, is easy to see in most people. In adults, the external auditory meatus is roughly 2.5 cm in length and 0.7 cm in diameter, although these dimensions vary quite a bit across individuals (in this chapter, all anatomical sizes, areas, and dimensions are for young adults). The external auditory meatus is not a straight, level tube; rather it runs slightly “uphill” (see Figure 13–2) and has a small bend between its opening and the tympanic membrane, where it ends. The bend is backward (and, thus, is not discernible in Figure 13–2) and occurs in many people at the location where the canal’s surrounding tissue changes from cartilage to bone. You may have noticed that your medical practitioner, when inserting an otoscope (ear scope) into your ear canal, gently pulls the pinna toward the back of the head. The practitioner does this to straighten out the natural bend in the tube for a more direct view of the tympanic membrane. The bend in the external auditory meatus is also a barrier to foreign objects moving easily from the outer ear to the delicate tissues of the tympanic membrane; in this sense, the shape of the canal serves a protective function. As can be seen in Figure 13–2, the part of the external auditory meatus close to the concha is surrounded by cartilage, which is a continuation of the tissue that makes up much of the pinna. The remaining length of the ear canal is encased by part of the temporal bone. The external auditory meatus ends as a closed tube at the tympanic membrane.
The primary auditory function of the external auditory meatus is to conduct sound energy from the concha to the tympanic membrane. The sound energy is in the form of molecule-sized pressure waves, as described in Chapter 7. The external auditory meatus acts like a resonator, causing sound energy at certain frequencies to vibrate with greater amplitude compared with the amplitude at other frequencies. Specifically, the external auditory meatus of an adult amplifies vibratory amplitude (sound wave energy) at frequencies around 3300 Hz. The specific resonant frequency at which the sound energy is “boosted” is determined by the length of the tube. The greater the length of the external auditory meatus, the lower its resonant frequency (Chapter 7). The resonant frequency is consistent with resonance characteristics of a tube closed at one end. Like all resonators, the boost in energy is not only at the peak frequency, but at surrounding frequencies as well.
The external auditory meatus protects the tympanic membrane and is a conduit for the removal of dead tissue away from the tympanic membrane. The tympanic membrane is protected by cerumen (ear wax) that is secreted by sweat glands in the cartilaginous walls of the external auditory meatus. The fluid produced by these microscopic glands mixes with a substance called sebum to create cerumen. This waxy mixture drains into the walls of the ear canal by sliding down tiny hairs that emerge from the cartilage and project into the canal. Cerumen lubricates the ear canal, presents a barrier to larger foreign objects (insects, for example), and may also block the movement of bacteria or fungal agents toward the tympanic membrane.
The dead tissue that is removed through the external auditory meatus comes from the tympanic membrane. The tympanic membrane has three tissue layers. The layer on the lateral side (that is, facing the pinna) is a continuation of the skin of the external auditory meatus, which has a superficial layer composed of keratinized epithelium. These superficial cells lack a nucleus or other small organs (often called organelles) that are typical of other tissue cells in the body. They are called “keratinized” because they are essentially dead cells (hence the lack of a nucleus and organelles typically involved with metabolic functions). The keratinized cells serve a protective function, with the outermost layer continuously “shedding.” The shed cells do not pile up in front of the tympanic membrane, but rather are carried down the incline of the external auditory meatus and dispersed as they approach the concha.
Imagine an experiment in which a pressure wave (sound wave) made up of frequencies of equal energy between 1 and 10,000 Hz is introduced at the entrance to the ear canal (external auditory meatus). Now imagine placing a very small, sensitive microphone deep in the ear canal, precisely at the location where the ear canal ends at the tympanic membrane. You might think that the microphone would pick up the same sound that entered the ear canal. But think again. We know that the ear canal is a tube open at one end (at the concha) and closed at the other end (at the tympanic membrane) and that such tubes have specific resonant frequencies, depending on their length. The ear canal is a tube roughly 2.5 cm long and closed at one end; by the quarter-wavelength rule the primary (lowest) resonant frequency is 3300 Hz. This means that the pressure wave arrives at the microphone at the tympanic membrane with an energy boost at 3300 Hz, and to a lesser degree at frequencies immediately surrounding the computed resonant frequency. The change in the spectrum of a sound from one location to another is called a transfer function. The flat spectrum (equal energy at all frequencies) introduced at the pinna opening of the ear canal is transformed by the acoustic characteristics of the external auditory meatus to a spectrum at the tympanic membrane favoring frequencies at and around 3300 Hz.
The tympanic membrane, or eardrum, is shown in Figure 13–5. The tympanic membrane is the boundary between the outer and middle ear. The foundation of that boundary is bone. The circular perimeter of the tympanic membrane is linked to the bony foundation via a cartilage-ligamentous ring called the annulus (which is whitish in appearance compared with the slightly darker skin-tone of the ear canal). The annulus fits into a small circular, bony depression at the boundary of the outer and middle ear, anchoring the tympanic membrane in place.
Figure 13–5 depicts the right tympanic membrane as seen through an otoscope. The otoscope has a viewing lens and a light source to illuminate the ear canal and the tympanic membrane. As a result of the shallow conical shape of the tympanic membrane and its tilt with the lower half farther from the scope than the upper half, light reflects off the normal tympanic membrane as a “cone of light” that is directed forward, downward, and to the right. The cone of light directed to the right of the observer, as in Figure 13–5, is typical when viewing the right ear; the cone is directed to the left when viewing the left tympanic membrane. Parts of the middle ear bones (ossicles—malleus, incus, and stapes) can be seen through the membrane.
In the otoscopic view, the tympanic membrane looks more or less flat—not conical, not tilted along its top-to-bottom axis relative to the observer. The cone shape and the tilt are seen in the cross-sectional rendition of the tympanic membrane in Figure 13–2. The tympanic membrane has the shape of a flattened bowl with a conical base that points into the middle ear cavity. The “tip” of the inverted cone is called the umbo, and serves as the point of attachment for the lower end of the malleus, one of the three middle ear bones described below.
As noted above, the tympanic membrane is composed of a three-layer sheet of tissue. The layer at the termination of the external auditory meatus is composed of epithelial tissue that is a thinned continuation of the skin lining the canal. The layer of tissue facing the middle ear cavity is also very thin epithelium, but with a different cell structure than the layer facing the external auditory meatus: the layer facing the middle ear has an epithelial structure much like the mucosal tissue that covers the upper airways. The middle layer is the primary vibratory component of the tympanic membrane and is composed of fibrous tissue interlaced with collagen fibers. The highly elastic collagen fibers are arrayed throughout the middle layer in many different directions (from center to edge, radially, horizontally), forming a dense interlacing of tissue having complex vibratory capability. This middle layer is highly sensitive to very small pressures exerted by the vibrating air molecules that create sound waves, but capable of resisting extremely high static (non-vibratory) pressures such as those encountered in a poorly pressurized airplane cabin.
The three-layer tissue structure is called the pars tensa and makes up nearly the entire surface area of the tympanic membrane. The exception is a small region in the upper part of the membrane called the pars flaccida (shown in Figure 13–5; the pars flaccida is also called Shrapnell’s membrane), which lacks the middle, fibrous layer present in the rest of the tympanic membrane.
The overall thickness of the three layers of the tympanic membrane is little more than one-tenth of a millimeter (equal to 0.0001 meter). The membrane has a diameter of roughly 8 to 10 mm and a surface area of about 55 mm2. The surface area of the tympanic membrane plays an important role, described below, in how the middle ear mechanism transforms sound energy from the medium of air (in the external auditory meatus) to the medium of fluid (in the cochlea).
MIDDLE EAR (CONDUCTIVE MECHANISM)
The middle ear is a tiny (1–2 cm3) air-filled cavity lined with a mucous membrane and surrounded by bone. Figure 13–6 shows the size of a cube with sides 1.15 cm in length; the volume of such a cube is 1.52 cm3, giving a fair idea of the tiny volume of the middle ear cavity (the volume is variable across humans and may even be smaller—within a range of 0.5 to 1 cm3—in many individuals; see Stepp & Voss, 2005). The middle ear cavity is complexly shaped and contains tiny, movable bones (ossicles), ligaments, two muscles, nerves, and the proximal end of a tube that leads to the nasopharynx.
The middle ear can be seen in coronal section in Figure 13–2. This two-dimensional illustration shows the lateral boundary of the cavity (the medial surface of the tympanic membrane plus the bone above it), the medial boundary (the bony covering of the inner ear, best defined by the region around and below the attachment of the footplate of the stapes into the oval-shaped “window” of the bony labyrinth—the bony encasement of the inner ear structures), and the bony floor and roof of the cavity. The section does not provide a good view of the posterior wall of the cavity or the front of the cavity, the latter cut away for the illustration.
The middle ear cavity can be conceptualized as having six surfaces—medial, lateral, superior, inferior, posterior, and anterior—much like a cube can be described with its six surfaces except that these surfaces are irregular and of unequal area. Figure 13–7 presents a schematic representation of the middle ear cavity with five of its six surfaces labeled with selected structures. The view is directed toward the medial wall of the middle ear cavity. The sixth surface is the lateral surface of the middle ear cavity, largely made up of the tympanic membrane. For this illustration the lateral surface has been cut away to allow a view of the middle ear cavity from lateral-to-medial. The different surfaces, or cavity walls, are referred to throughout the discussion of middle ear anatomy. To anticipate a fuller discussion of landmarks on the walls of the middle ear (tympanic) cavity: the medial wall shows the oval and round windows of the bony labyrinth, the prominence of the facial canal, and the promontory; the lateral wall is defined almost completely by the tympanic membrane; the superior wall, or roof of the middle ear cavity, is a thin bony plate called the tegmen tympani, separating the middle ear cavity from the cranial cavity (the space inside the skull); the posterior wall contains the aditus in its upper part and lower on the wall the pyramidal eminence, a minuscule, cone-shaped protuberance containing the stapedius muscle, which sends out a tendon to attach to the stapes (only the muscle and tendon are shown, not the bony casing); the inferior surface is a thin, plate-like bone separating the middle ear cavity from the internal jugular vein; and the anterior wall shows the location of the bony opening of the auditory (eustachian) tube and the bony canal housing the tensor tympani muscle, which sends out a tendon to attach to the malleus. Each of these features is discussed below.
The middle ear cavity is often partitioned into an upper chamber, called the epitympanum (also referred to as the attic of the middle ear), a middle chamber, the mesotympanum, and a lower chamber, the hypotympanum. There are no clear-cut boundaries between these chambers. Nevertheless, the epitympanum is typically regarded as the chamber above the tympanic membrane and contains the head of the malleus and the body of the incus (note in Figure 13–2 their location in the small, upper cavity of the middle ear). The epitympanum also includes an opening in its posterior wall (see Figure 13–7) called the aditus, which is directed posteriorly to the antrum, an airspace deep within the mastoid bone. The aditus and antrum allow the air in the middle ear cavity to communicate with air-filled cells of the mastoid bone. The aditus, antrum, and cells of the mastoid are shown in the coronal view of Figure 13–8. In this simplified view, the bony, ligamentous, and muscular structures have been removed from the middle ear cavity.
Figure 13–6. Volume of middle ear cavity in the form of a cube. Actual size is shown at the left, with an expanded cube at the right shown for illustration purposes. Approximate dimensions are for the real (left) cube.
Figure 13–7. Schematic representation of the middle ear cavity, looking in from the tympanic membrane, which has been removed. Structures on the posterior, anterior, superior, inferior, and medial walls are shown.
The mesotympanum is the space between the top and bottom edge of the tympanic membrane. The mesotympanum contains the malleus and long process of the incus, as well as the stapes and the oval and round windows (openings into inner ear, described below). The oval and round windows are features of the medial wall of the tympanic cavity (see Figure 13-7).
The hypotympanum, or lowest space of the cavity, does not contain functional components of the middle ear but is directly above the internal jugular vein (see Figure 13–7), an important part of the cardiovascular system by which blood is drained from the head. The inferior surface of the middle ear cavity—that is, the floor of the cavity—is separated from the internal jugular vein by a thin plate of bone. Just below this thin plate the internal jugular vein makes a right-angle turn along its pathway from the posterior part of the brain to larger arteries in the chest. Knowledge of the hypotympanum’s relationship to this venous structure is critical to patient safety during middle ear surgery.
Ossicles and Associated Structures
The ossicles are the three smallest bones in the human body. They extend across the middle ear cavity from the tympanic membrane to the oval window of the cochlea. The individual ossicles plus their articulated configuration are shown in Figure 13–9. To appreciate the miniaturized size of these bones, consider that all three fit (at the same time) quite easily on the lower half of the surface of a penny (see actual size cube in Figure 13–6).
The malleus (also called the “hammer”) has a handle-like part called the manubrium, which is attached along its length to the tympanic membrane. The lowest point of attachment, at the inferior end of the handle, is called the umbo. The umbo is a prominent landmark on the tympanic membrane when it is viewed through an otoscope (see Figure 13–5). At the top of the handle is a short neck, and above that the head of the malleus. A curved edge on one side of the head of the malleus is the point of articulation with the incus.
Figure 13–9. The ossicles shown as individual, disarticulated bones (top) from left ro right the malleus, incus, and stapes, and in their fully articulated configuration (bottom).
The incus (or anvil) is the middle ossicle. The incus has a short body that issues two processes, one short and stubby, the other long and ending in the hook-like lenticular process. The body of the incus contains a curved surface for its articulation with the malleus, fitting into the curved surface of the malleolar head. The hook-like end of the long limb of the incus, the lenticular process, is a subtle modification of the bone that forms a joint with the stapes.
The stapes (or stirrup) is the most medial and smallest ossicle. The very short head of the stapes is attached to the lenticular process of the incus to form the incudo-stapedial joint. A short neck connects the head of the stapes to two arches, the anterior and posterior crura, which extend from the neck and attach to an oval-shaped base, the footplate of the stapes. The stapes fits into the oval window, an opening cut into the bony casing of the inner ear (see Figure 13–7). The footplate is held in that window by a fibrous ligament, the annular ligament (like the one securing the tympanic membrane to its circular, bony frame) that adheres to the perimeter of the footplate and attaches to the bony perimeter of the oval window.
The malleo-incudal and incudostapedial joints are synovial, meaning they are both joined together by a fibrous capsule filled with synovial fluid. Synovial fluid, a relatively viscous (thick) secretion from the capsule cells, reduces friction between the articulating surfaces of the joints as they move against each other. Movement at these joints is associated with the conduction of sound vibration from the tympanic membrane to the cochlea, as well as with displacement of the ossicular chain when static (non-vibrating) pressures are applied to the tympanic membrane. The malleo-incudal joint is more strongly linked than the incudo-stapedial joint. When there is a break in the ossicular chain, such as might occur when the skull is fractured, separation of the ossicles is much more likely to occur at the incudostapedial joint.
Surprisingly, the precise role of the ossicular joints in the conduction of sound energy from the tympanic membrane to the footplate of the stapes is unknown. Especially because birds, one of our more direct evolutionary forebearers, have a single ossicle that responds to sound energy with piston-like (rigid body) movements, it is fair to ask how the evolution of a single-ossicle transmission system to a three-ossicle system benefits human auditory capabilities. What is known is that the three ossicles do not move as a strictly rigid, uniform system (see Nakajima et al., 2005 and Volandri et al., 2012 for excellent reviews). Rather, there is some independence of ossicle motion during vibration by sound waves, with most of the non-piston-like movement, observed in experiments in which two ossicles are seen to have somewhat separate motions when they vibrate, occurring at the incudomalleolar joint. Such semi-independent motion of individual ossicles, however, has yet to be shown as providing a clear auditory advantage over the simpler, single-ossicle system.
The ossicles are anchored to the walls of the middle ear cavity by several ligaments (as well as muscles, described in the next section). There are six ligaments in the middle ear cavity, three associated with the malleus, two with the incus, and one with the stapes. Figure 13–10 shows a coronal view of the ligaments and muscles of the middle ear.
The malleal ligaments include the anterior, lateral, and superior ligaments. The anterior malleal ligament has one attachment on the anterior wall of the tympanic cavity and the other at the neck of the malleus. Because the view in Figure 13–10 is coronal, from the front of the head, only one point of attachment is indicated. The lateral malleolar ligament has an attachment on the lateral wall of the middle ear cavity, just above the tympanic membrane, and another on the lower part of the head of the malleus. The superior malleolar ligament is attached to the “roof” of the middle ear cavity (tegmen tympani) and the superior part of the head of the malleus.
The incus is supported by the posterior and superior ligaments. The posterior incudal ligament attaches the short limb of the incus to the posterior wall of the middle ear cavity, very close to the entrance (aditus) to the mastoid bone. The superior incudal ligament extends from the roof of the tympanic cavity to the body of the incus. Only one ligament attaches to the stapes. This is the annular ligament, which anchors the perimeter of the stapes footplate to the bony oval window.
Together these six ligaments (along with the muscles, described below) stabilize the ossicular chain and permit its piston-like motions (on an axis through the umbo to the footplate of the stapes) as well as motions of the joints that may be partially independent of the piston action. The ligaments are important to the efficient functioning of the ossicular chain in transmitting sound energy from the tympanic membrane to the fluid in the cochlea.
Figure 13–10. View of the right middle ear, showing points of attachment for six ligaments and two muscles.
The two muscles of the middle ear cavity are the tensor tympani and the stapedius (see Figure 13–10). The tensor tympani muscle originates within a bony canal in the front wall of the middle ear cavity, just above the bony portion of the auditory tube (the tube that connects the middle ear cavity to the nasopharynx, also called the eustachian tube). The muscle fibers run backward along this bony canal, toward the middle ear cavity, and at the opening into the cavity give off a tiny tendon. This tendon makes a sharp turn toward the lateral wall of the tympanic cavity and inserts to the neck of the malleus. Contraction of the tensor tympani muscle pulls the handle of the malleus medially and forward, retracting the tympanic membrane into the middle ear cavity. This action may stiffen the tympanic membrane and the ossicular chain, thereby reducing the vibratory efficiency of the conductive mechanism. The muscle is thought to provide a protective function for excessive movement of the entire conductive mechanism, which may cause damage to structures of the inner ear. Contraction of the tensor tympani muscle can occur in response to very high intensity sounds or even during chewing. The tensor tympani muscle is innervated by a motor branch of the trigeminal nerve (cranial nerve V).
Fibers of the stapedius muscle originate in a tiny conical hole in the posterior wall of the middle ear cavity and give way to a tiny tendon that inserts to the posterior crus and neck of the stapes. In Figure 13–10 only the tendon is shown, because the muscle fibers are hidden within the pyramidal eminence (see Figure 13–7). When the muscle contracts, it pulls the stapes away from its “fit” into the oval window, the movement allowed by the flexibility of the annular ligament. The precise nature of the pull is to tilt the footplate away from the oval window, with the front part of the footplate most affected. Contraction of the stapedius muscle stiffens the entire ossicular chain and is thought to protect the inner ear from excessive displacement of the footplate into the fluid of the inner ear. The stapedius muscle plays a significant role in the acoustic reflex, which is thought to protect inner ear structures from damage due to excessively high sound levels. The stapedius muscle is innervated by a motor branch of the facial nerve (cranial nerve VII) and is a key component of the acoustic reflex (see below).
Scientists don’t always agree. Although the complicated arrangement of the ossicles seems to be in the service of efficient transmission of sound energy from the tympanic membrane to the cochlea, there are dissenters who have a bone to pick with this traditional view. Luers and Hüttenbrink (2016) argue that the ossicular arrangement in humans, with moving joints and strangely oriented extensions, is not specialized for sound transmission, but rather serves the combined functions of responding to molecule-size pressure variations—sound waves—and protection against the wide range of “ambient” static pressures that may exceed sound pressures by a million (or more) times. Why do they argue so? First, joint movement at the malleo-incudal and incudo-stapedial joints is different when responding to sound vibrations compared with large, non-sonic pressures (such as in an airplane cabin). The joint movements observed in response to large, static pressures seem to be exactly what you would expect if the ossicles were moving to limit displacement of the stapes into the oval window. These movements have a lot of joint rotation, unlike the more piston-like movement of the ossicular chain during sound vibration. Second, reconstructive otosurgeons (surgeons who “rebuild” damaged structures of the middle ear) have found that the most effective middle ear prosthesis for a person with a missing ossicle (or two) is a straight strut. In other words, when re-creating a pathway for sound between the tympanic membrane and the oval window, they do not mimic the contortions and complex articulations of the healthy ossicles; rather, they simply connect the two ends with a straight column of surgical material. The result is excellent sound transmission across the middle ear. Third, and finally, the ossicles of an owl’s ear are basically arranged in a straight line—and the hearing of owls is known to be exquisite. This suggests that a complicated, human-like ossicle arrangement is not required for excellent sound transmission from tympanic membrane to cochlea. This bone of contention about the middle ear bones is not all bad. Such controversies keep science healthy and moving forward.
The auditory tube (known also as the eustachian tube, after the sixteenth-century Italian anatomist Bartolomeo Eustachi) is shown in Figure 13–2 as a bone-encased, open tube in the lower, anterior wall of the middle ear cavity. The tube extends downward, forward, and medially from this bony part, becomes cartilaginous along its path, and terminates in a closed but flexible end in the nasopharynx at roughly the same level as the nostrils. The total length of this tube in adult humans is about 3.8 cm (1.5 inches). When the tube opens, it connects air in the middle ear cavity to air in the nasopharynx.
The nasopharynx end of the auditory tube is usually closed, but can be opened by swallowing, yawning, or chewing. The opening of the auditory tube is by muscular means, and likely includes simultaneous contraction of the tensor veli palatini muscle, the superior constrictor muscle, and the levator veli palatini muscle (Okada et al., 2018; and see Chapter 4). Immediately after the tube is opened, it springs back to the closed position due to the elasticity of the tissue. Intermittent opening of the auditory tube is important to expose air in the middle ear cavity to atmospheric pressure, which is typically the pressure in the nasopharynx when the mouth, velopharynx, or both are open. This intermittent opening of the auditory tube maintains atmospheric pressure in the middle ear cavity. Under normal circumstances, pressure at the surface of the tympanic membrane facing the external auditory meatus is also atmospheric. Therefore, in a healthy ear, pressure on both sides of the tympanic membrane (the external ear canal side and the middle ear side) is atmospheric. Under these pressure conditions, the tympanic membrane is at its rest position and produces the most efficient vibratory activity in response to sound energy. A pressure imbalance across the tympanic membrane pushes the membrane outward (greater pressure in middle ear cavity compared with external auditory meatus) or inward (greater pressure in external auditory meatus compared with middle ear cavity), either of which stiffens the tympanic membrane and ossicles and reduces the vibratory efficiency of the conductive mechanism. When the auditory tube does not open intermittently, as may occur in the case of a middle ear infection, pressure in the middle ear cavity may decrease relative to pressure in the external auditory meatus and push the tympanic membrane inward, away from its rest position, thereby stiffening the conductive mechanism. This results in a temporary conductive hearing loss that resolves when the infection clears. When a bulging or retracted tympanic membrane is viewed through an otoscope, the cone of light may be dimmer or completely absent.
Medial and Lateral Wall Views of the Middle Ear: A Summary
Figures 13–11 and 13–12 offer summary views of the middle ear structures. Figure 13–11 shows middle ear structures as viewed from the inner ear, looking out to the eardrum; this view looks toward the lateral wall of the middle ear cavity and associated structures. Anterior is to the left and superior to the top. The stapes has been removed to provide a direct view of the lenticular process of the incus as well as the attachment of the handle of the malleus to the tympanic membrane. Note the tensor tympani muscle within the bony canal just above the auditory tube, and its tendinous attachment to the handle of the malleus. The internal carotid artery, the main supply of blood to the cerebral hemispheres of the brain, is seen just in front and below the tympanic membrane (see also Figure 13–7). Cranial nerve VII (the facial nerve) is posterior to the tympanic membrane and runs in a bony canal in the back wall of the middle ear cavity. It gives off a branch called the chorda tympani that runs between the malleus and incus before exiting the skull (just below the bottom portion of the nerve as shown; the top portion of the vertical section of nerve has been cut). The chorda tympani carries taste sensation from the anterior two-thirds of the tongue into the main bundle of fibers of cranial nerve VII, which travels through the internal auditory meatus to the brainstem.
Figure 13–12 shows middle ear structures viewed from the vantage point of the tympanic membrane, looking toward the medial wall of the middle ear cavity. In this view, the incus and malleus have been removed; anterior is to the right. The crura of the stapes are shown clearly. The promontory is the bony casing of the basal turn of the cochlea. Note the attachment of the stapedius muscle to the posterior wall of the middle ear cavity at one end, and its distal tendon to the neck of the stapes at the other end. The proximal tendon of the stapedius muscle, arising from a tiny foramen in the posterior wall of the middle ear cavity, cannot be seen in this image. The bony casing (prominence) of one of the semicircular canals is also shown. Note the course of the facial nerve after it exits the brainstem and internal auditory meatus to enter the bony casing of the middle ear. The nerve runs horizontally and then turns south, as it were, toward its exit from the skull. Figure 13–12 shows the beginning of the turn (with bone cut away and the nerve exposed), labeled the geniculum of the facial nerve. From the geniculum the facial nerve descends in the bony casing of the middle ear, exiting the skull through the stylomastoid foramen, one of the many openings in the base of the skull through which cranial nerves pass from inside to outside the skull (or from outside to inside).
Figure 13–11. View of middle ear cavity as if looking at the structures from the inner ear, toward the tympanic membrane. The stapes has been removed for better viewing.
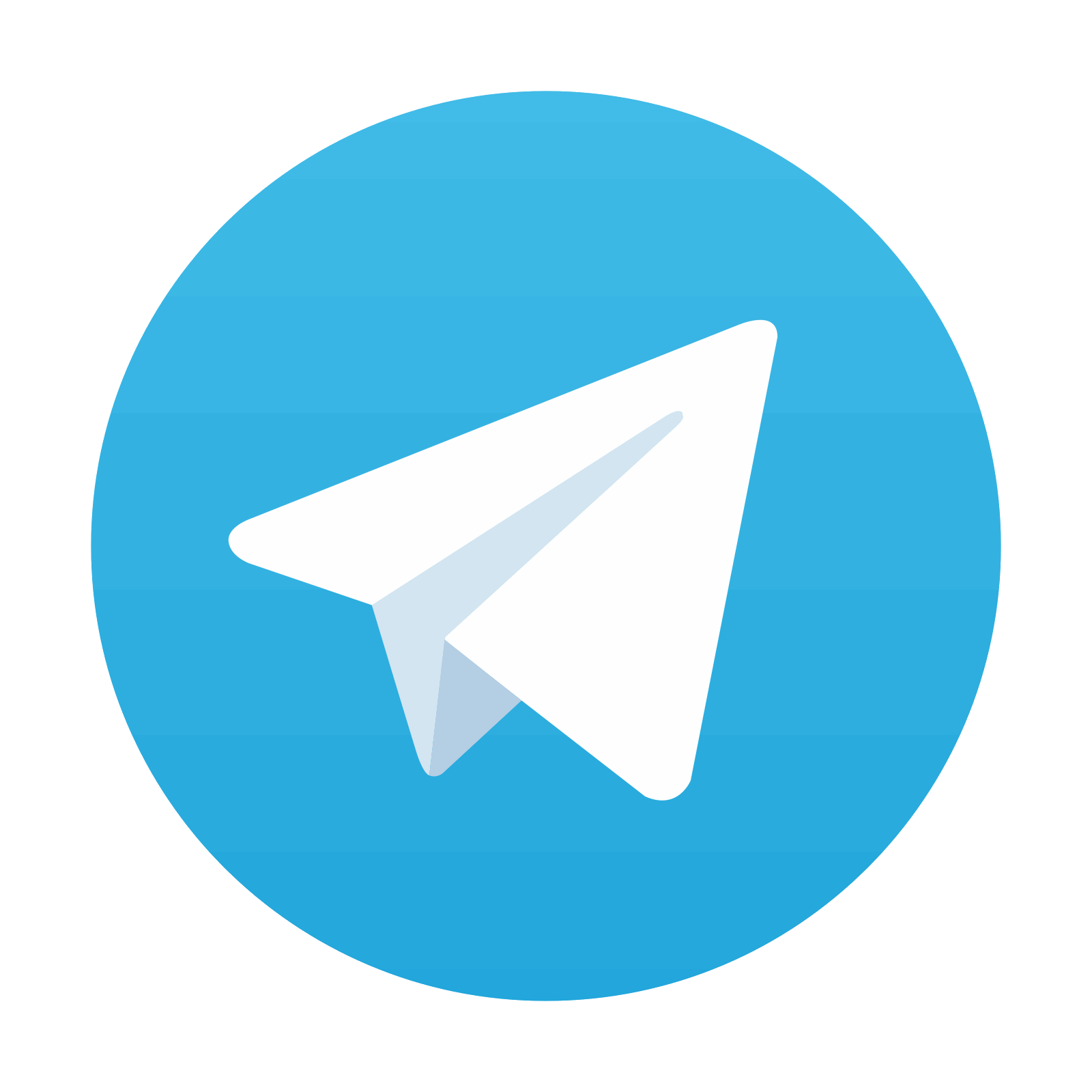
Stay updated, free articles. Join our Telegram channel

Full access? Get Clinical Tree
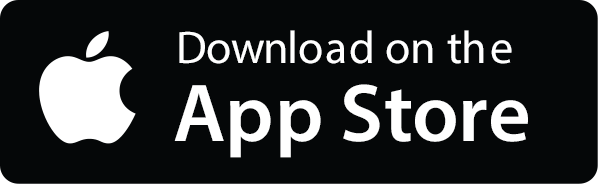
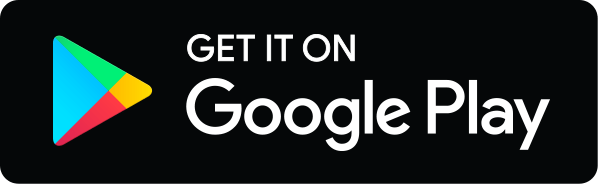